The pupillary light response as a physiological index of aphantasia, sensory and phenomenological imagery strength
Abstract
The pupillary light response is an important automatic physiological response which optimizes light reaching the retina. Recent work has shown that the pupil also adjusts in response to illusory brightness and a range of cognitive functions, however, it remains unclear what exactly drives these endogenous changes. Here, we show that the imagery pupillary light response correlates with objective measures of sensory imagery strength. Further, the trial-by-trial phenomenological vividness of visual imagery is tracked by the imagery pupillary light response. We also demonstrated that a group of individuals without visual imagery (aphantasia) do not show any significant evidence of an imagery pupillary light response, however they do show perceptual pupil light responses and pupil dilation with larger cognitive load. Our results provide evidence that the pupillary light response indexes the sensory strength of visual imagery. This work also provides the first physiological validation of aphantasia.
Editor's evaluation
This is a rigorous study of the relation between the vividness of visual imagery and the pupillary light response that can result from it. It provides evidence for the absence of imagery in individuals that self-report as aphantasic. The results will likely be of interest to researchers in a range of disciplines such as psychology, neuroscience and philosophy.
https://doi.org/10.7554/eLife.72484.sa0Introduction
Our pupil’s ability to change size is an important physiological response that adjusts the amount of light hitting the retina to optimize vision and protect the retina. Pupils constrict in response to brightness whereas they dilate in response to dark conditions (known as the pupillary light response or reflex); while these responses are related, they are considered to be driven by different neural pathways (see Mathôt, 2018 for a review). These involuntary pupil responses were once thought to be driven only by afferent visual stimulation, or automatic activation from emotional responses (Bradley et al., 2008; Partala and Surakka, 2003), however, recent studies suggest that pupil size is sensitive to higher order perceptual and cognitive processes. For example, subjective interpretation of equiluminant stimuli, such as greyscale images of the sun elicit greater pupil constriction than those of the moon (Binda et al., 2013b). The target of covert visual attention can drive pupillary light responses (Binda et al., 2013a), as can visual working memory content (Zokaei et al., 2019), but see Blom et al., 2016. Further, evidence suggests that it might be mental imagery that is driving some of these cognitively induced pupil responses (Laeng and Sulutvedt, 2014) and recent work has shown that there are pupillary light responses even when reading or listening to words conveying some level of brightness (Mathôt et al., 2017). Hence, it remains unknown if the variations in pupil response to equiluminant stimuli are due to high-level semantic content or low-level visual imagery.
Visual imagery is considered a useful and often essential tool in many aspects of cognition. It plays an important role in the retrieval of items from short- and long-term memory (Pearson, 2019), visual working memory (Keogh and Pearson, 2011; Keogh and Pearson, 2014; Pearson and Keogh, 2019), acquisition of language (Just et al., 2004), and spatial navigation (Sack et al., 2005; Guariglia and Pizzamiglio, 2007). It is also used for simulating both past and potential future events (Schacter et al., 2012; Schacter and Madore, 2016), the latter often as a form of self-motivation for goal attainment (Szpunar et al., 2007). As essential to cognition as it might appear, large individual differences exist in visual imagery and its vividness. Some people report imagery as so vivid it feels almost like perception, while a small percentage of otherwise healthy people seemingly do not have the capacity for visual imagery at all – they report that when they think about how an object looks, there is no sensory-like experience of it whatsoever (Galton, 1880). This condition has been recently termed ‘aphantasia’ (Zeman et al., 2015); it can be congenital, persisting throughout one’s lifetime (Zeman et al., 2015) or acquired (Zeman et al., 2010), is associated with a range of differences in general cognition (Dawes et al., 2020; Keogh et al., 2021a, Keogh and Pearson, 2021), including dampened fear responses to imagined scary scenarios (Wicken et al., 2021). The existence of aphantasia has also been established using objective techniques that measure the low-level sensory elements of imagery (Keogh and Pearson, 2018).
The rationale of the current study was to accurately and objectively utilize individual differences in mental imagery (both in the general population and aphantasia) to provide strong evidence that it is the sensory strength and subjective vividness of imagery that drives the cognitive pupillary light response. Similar rationale has been previously used by linking the vividness and objective sensory strength of imagery to behavioural or neurological measures (Bergmann et al., 2016; Shine et al., 2015; Wassell et al., 2015). If imagery plays a causal role in endogenous pupil size changes, then individual differences in imagery should be reflected in these measures.
Here, we utilized both subjective and objective measures of visual imagery ability and show that, within the same individual, greater pupillary light responses during imagery are associated with reports of stronger and more vivid imagery. We then used this task to compare imagery strength between individuals and test the veracity of the self-reported lack of imagery in aphantasia. We show that while aphantasic individuals display pupil contraction to perceptual brightness and dilation with effort (cognitive load), they do not show any evidence of pupil change in response to attempts at imagery – providing the first objective physiological evidence confirming the existence of aphantasia.
Results
The imagery pupillary light response in the general population
In the pupillometry imagery task (based on Laeng and Sulutvedt, 2014; see Figure 1A), participants who reported having visual imagery were presented with one or four ‘Bright’ or ‘Dark’ triangles for 5 s (see Figure 1—figure supplement 1 for images used). Following this they viewed a blank screen for 8 s (which allowed any after-images to fade) and were then instructed to imagine the prior image/s for 6 s, after which they rated the vividness of their imagery from 1 to 4. Pupils showed a clear pupillary light response to perceptual images (Figure 1B; perception section; a significant effect of perceptual luminance F(1, 41) = 190.02, p < 0.001.) This trend was mirrored in the imagery period showing a significant main effect of imagery luminance (Figure 1B, box insets: imagery section; F(1, 41) = 67.42, p < 0.0001), indicating that imagery also demonstrates a pupillary light response. Post hoc analysis using the Bonferroni correction for multiple comparisons found that for both Set-Size-One and Set-Size-Four, the pupil size in the Dark condition was significantly greater than in the Light condition during imagery (p < 0.001 and p < 0.05, respectively, see Figure 1C). There was no main effect of set size during perception F(1, 42) = 2.67, p = 0.11. However, there was a significant main effect of set size during imagery F(1, 41) = 6.48, p = 0.015, with less constriction/more dilation for Set-Size-Four (when averaged across the brightness conditions). This is consistent with previous studies suggesting that pupil size is influenced by cognitive load (Kahneman and Beatty, 1966; Laeng et al., 2011; van der Wel and van Steenbergen, 2018). Post hoc analysis also demonstrated that in the Bright condition, Set-Size-Four resulted in significantly more pupil dilation during imagery than Set-Size-One (p = 0.001). However, in the Dark condition pupil dilation during Set-Size-Four imagery was not significantly different to Set-Size-One (p = 0.266).
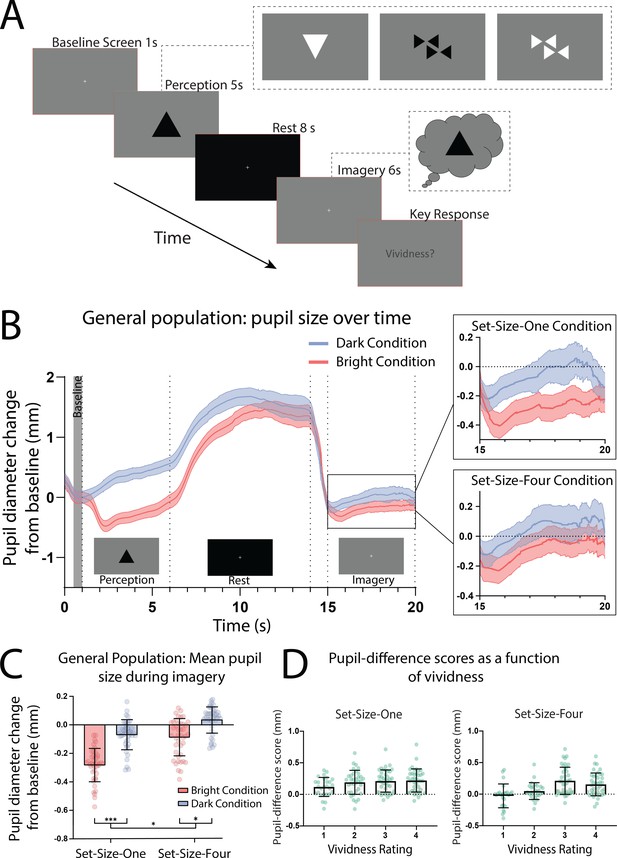
Pupillary response task schematic and eye-tracker results for the general population.
(A) Pupillometry imagery experiment timeline. Each trial began with the presentation of a white fixation cross at the centre of a grey screen (baseline screen) for 1 s. An image was then presented at the centre of this grey screen for 5 s (either one or four triangles of varying brightness, see Figure 1—figure supplement 1 for illustrations of all stimuli). Participants were instructed to focus on the stimuli during this time and memorize its size, orientation, and level of brightness. Next, a black screen with a white fixation cross was presented for 8 s, allowing the perceived after-image to completely fade and pupils to dilate back to equivalent resting levels. The grey baseline screen was then presented again for 6 s. During this time, participants were cued (via two auditory beeps) to actively start imagining the stimuli observed previously during that trial, while maintaining focus on the fixation cross. These beeps were presented 1 s into the grey screen period leaving 5 s of imagery time. Lastly, participants were prompted to report the vividness of their imagery during those previous 5 s on a scale of 1–4 (1 being ‘not vivid at all – no shape appeared in imagery’; 4 being ‘very vivid – almost like seeing it’) via key response. (B) Mean pupil size waveforms for the general population, presented as mm change from baseline. Left panel: data averaged across the course of a trial for Bright (red lines) and Dark (blue lines) conditions for the general population. Right panels: Set-Size-One and Set-Size-Four conditions are shown separately during the imagery period (i.e. pupil size from seconds 15 to 20). Shaded error bands represent the standard error of the mean (± standard error of the mean [SEM]). (C) Mean pupil size change from baseline during imagery (i.e. averaged from seconds 15 to 20 of trials) of Bright (red bars) and Dark stimuli (blue bars). (D) Pupil-difference scores (difference in pupil size during imagery between bright and dark conditions) as a function of subjective vividness ratings for Set-Size-One and Set-Size-Four conditions. Data points represent one participant. Error bars indicate ± SEM, calculated across participants. *p < 0.05, ***p < 0.0001.
-
Figure 1—source code 1
r Code for LME analysis of vividness ratings.
Source code file 1 provides the r code for the LME used to analyse the vividness data in Figure 1D.
- https://cdn.elifesciences.org/articles/72484/elife-72484-fig1-code1-v2.zip
-
Figure 1—source data 1
Source data for Figure 1C.
- https://cdn.elifesciences.org/articles/72484/elife-72484-fig1-data1-v2.csv
-
Figure 1—source data 2
Source data for Figure 1D.
- https://cdn.elifesciences.org/articles/72484/elife-72484-fig1-data2-v2.csv
Prior behavioural work suggests we have reasonable metacognition of visual imagery, that is we are able to estimate the strength of imagery on a trial-by-trial basis (Pearson et al., 2011; Rademaker and Pearson, 2012). Here, we compared pupil responses to the trial-by-trial ratings of vividness. Pupil-difference scores are shown as a function of intraindividual vividness ratings for Set-Size-One and Set-Size-Four (see Figure 1D). A 2 × 4 linear mixed-effects analysis (2 (set size: 1, 4) × 4 (vividness rating: 1, 2, 3, 4)) demonstrated there was a significant effect of vividness (χ2(3) = 49.54, p = 1.004e−10), with larger pupillary light response for more vivid imagery trials (for both set sizes, see Figure 1D and fixed effects estimates in Supplementary file 1). These data demonstrate that the pupillary light response tracks the phenomenological vividness of visual imagery from moment to moment.
If the sensory strength of imagery is indeed driving the imagery pupillary light response, then the degree to which this response occurs should be related to independent objective measures of imagery strength in each individual. To assess this, we utilized the binocular rivalry method (Pearson, 2014; Pearson et al., 2008), which allows the objective assessment of the sensory strength of imagery, without relying on any subjective reports (Chang and Pearson, 2018). This is achieved by measuring the degree to which an individual’s imagery biases subsequent binocular rivalry perception. We compared pupil-difference scores (imagery of dark stimuli–bright stimuli, such that larger scores indicate a larger pupillary light response) with imagery strength measured using the binocular rivalry paradigm, in which higher priming scores indicate stronger imagery (Figure 2A; Pearson et al., 2008; Pearson et al., 2011). Within the general population, degree of pupil change in the Set-Size-One condition correlated positively with imagery strength, using Pearson’s correlation coefficient (rp(41) = 0.62, p = <0.0001, see Figure 2B: green circles and green trendline). The Set-Size-Four pupil data set violated normality (Shapiro–Wilk test, p = 0.003), therefore, the Spearman’s correlational coefficient was used to assess its relationship with binocular rivalry priming. A significant positive correlation was found between Set-Size-Four pupil-difference scores and binocular rivalry priming (rs(41) = 0.46, p = 0.002, see Figure 2C: green circles and green trendline). This provides further evidence that the sensory strength of imagery content is driving the imagery pupillary light response.
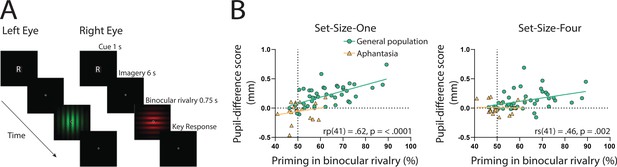
Binocular rivalry task schematic and correlational results.
(A) Example of an imagery trial for the binocular rivalry paradigm. Participants were cued to imagine either a red or green Gabor pattern prior to binocular rivalry with the letter ‘R’ or ‘G’ (750 ms). Participants then imagined the image for 6 s, after which they were presented with the binocular rivalry display (750 ms) and were asked to indicate which image was dominant. Trials where participants reported seeing the pattern they were cued to imagine as dominant were denoted as ‘primed’ trials. The number of primed trials divided by the total number of trials (excluding mock trials and mixed percepts) was used to calculate a percent primed score for each participant. (B) Correlation between visual imagery strength, as measured by the pupillary response task (pupil-difference score: difference between bright and dark conditions) and visual imagery strength as measured by the binocular rivalry task. Set-Size-One (left) and Set-Size-Four (right) conditions are shown. Scatterplots show the general population (green circles and green trendline) and aphantasic individuals (yellow triangles and yellow trendline) data. Correlation coefficients refer to the general population only (green trendline). All data points represent one participant.
-
Figure 2—source data 1
Source date for Figure 2B.
- https://cdn.elifesciences.org/articles/72484/elife-72484-fig2-data1-v2.csv
Aphantasia and the imagery pupillary light response
Our results indicate that the strength of the content of imagery drives the imagery pupillary light response in participants who experience visual imagery. The involuntary nature of this response provides a valuable objective measure of imagery strength. Accordingly, we sought to utilize this finding to test the veracity of a condition called aphantasia, that is if these individuals truly lack visual imagery, they should not show a pupillary light response to imagined images. However, if aphantasic individuals do show an imagery-based pupillary light response, one might interpret this as a form of imagery existing, but below threshold for conscious phenomenological awareness. We ran this same study in 18 aphantasic participants and compared their performance to that of the general population. These participants had contacted the lab reporting their lack of visual imagery and asked to participate in our research. They were also unaware of the goals and hypotheses of the current study. Aphantasia was confirmed in these individuals using self-report questionnaires (Vividness of Visual Imagery Questionnaire [VVIQ] score <32) and by means of our binocular rivalry priming method (priming <65%), based on cut-off points used in previous research (Keogh and Pearson, 2018).
Here, we again found a strong effect of stimulus luminance in the perceptual phase of the task for the aphantasic participants (Figure 3A: perception section; F(1, 17) = 81.18, p < 0.001), reflecting a functional pupillary light response. However, we found no significant effect of luminance on pupil size during imagery Figure 3A, box insets: imagery section; F(1, 17) = 0.193, p = 0.67 and Figure 3B shows the lack of pupil diameter change for bright stimuli (red bars) and dark stimuli (blue bars). Similarly, to the general population, there was no main effect of set size during perception F(1, 17) = 1.92, p = 0.18, however interestingly, there was a significant main effect of set size during imagery F(1, 17) = 6.185, p = 0.02, with greater pupil diameters for Set-Size-Four compared to Set-Size-One (when averaged across the brightness conditions). This suggests that the aphantasic participants were actively engaging in the imagery task and exerting greater cognitive effort for the larger set size (van der Wel and van Steenbergen, 2018). In comparison to the general population, 61.11% (11/18) of the aphantasic individuals had difference scores that were lower than or equal to 0 for set size one as compared to 9.5% (4/42) of the general population (see Figure 2B). To confirm this absence of an imagery effect in the aphantasia population, we compared the pupil-difference score obtained when comparing the bright and dark conditions for the control and aphantasia groups, and computed a Bayes Factor (H0: score = 0; H1: score ≠ 0; see Materials and methods). Controls showed very strong evidence for H1 (BF10 > 1010; Bayesian one-sample t-test), whereas the aphantasia population showed evidence for the null effect (BF01 = 3.180). A direct comparison between the control and aphantasia groups using a Bayesian repeated measure analysis of variance (ANOVA; see Materials and methods) showed very strong evidence for an effect of group (BF10 > 106). Finally, and as expected, pupil-difference scores (imagery of dark stimuli–bright stimuli) did not significantly predict imagery strength (measured using the binocular rivalry paradigm) for the aphantasic population (Figure 2B: yellow triangles; Set-Size-One: rp(17) = 0.20, p = 0.44); Set-Size-Four: (rp(17) = −0.08, p = 0.76). It should be noted that we could not perform an analysis on the vividness data in the same way as was done with the general population (Figure 1D) as the aphantasic individuals did not have any variation in their vividness ratings, reflecting their lack of subjective visual imagery (see Figure 3—figure supplement 1).
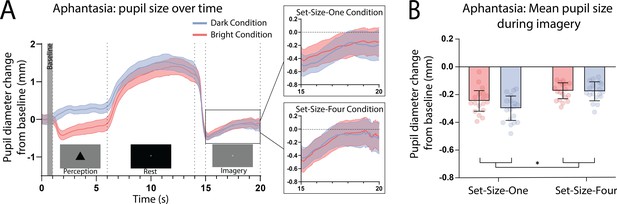
Pupillary response eye-tracker results for the aphantasic population.
(A) Mean pupil size waveforms over time. Left panel: data averaged across the course of a trial for Bright (red lines) and Dark (blue lines) conditions for the aphantasic population. Right panels: Set-Size-One and Set-Size-Four conditions are shown separately during the imagery period. (B) Mean pupil size change from baseline during imagery (i.e. averaged from seconds 15 to 20 of trials) of Bright (red bars) and Dark stimuli (blue bars). Error bars indicate ± standard error of the mean (SEM), calculated across participants. *p < 0.05.
-
Figure 3—source data 1
Source data for Figure 3B.
- https://cdn.elifesciences.org/articles/72484/elife-72484-fig3-data1-v2.csv
Age disparities between the groups are a potential confounding variable. This factor is of particular importance because the sensitivity of the pupillary light response, as well as maximum pupillary constriction velocity and acceleration, are thought to decline with age, beginning at 40–50 years old (Fotiou et al., 2007; Lobato-Rincón et al., 2014). However, trial time-course pupil waveforms are very similar for both general and aphantasic populations (Figures 1B and 3A, respectively). Both groups exhibited similar levels of pupil change during the perception phase of the task. Furthermore, a two-way ANCOVA was run on pupil-difference scores between general population and aphantasic groups with age as a covariate. Levene’s test and normality checks were carried out and the assumptions were met. We found a significant difference in pupil-difference score (F(1, 57) = 4.763, p = 0.033) between the groups when accounting for age. This provides evidence that decreased pupil responsiveness with age was not driving the observed effects.
Another possible explanation of our findings could be that the passive viewing of the perceptual images, lingering visual persistence and sluggish pupil responses could be driving our results. If this is the case, we would expect that pupil diameter during the perception of the images should correlate with pupil size during imagery for the corresponding images. Further, the pupillary light reflex during perception should be more pronounced in the control than the aphantasic populations. To investigate this possible alternative explanation of our data we first assessed the correlations between pupil diameter during perception of bright and dark images for Set-Size-One and -Four and their corresponding imagery conditions (control participants only). We found there were no significant correlations between any of the perception and imagery conditions, or the difference scores for set size one and four (all p > 0.40, see Figure 2—figure supplement 1). This lack of a correlation suggests that those individuals who have the largest pupillary light response while viewing the images, do not also have the greatest imagery driven pupillary light responses, making it unlikely that the pupil response while seeing the image is driving the mental imagery pupillary response. Next, we assessed whether the aphantasic individuals demonstrated any significant difference in their pupil responses to perceptual stimuli by running a 2 (image: bright and dark) × 2 (set size: 1 and 4) × 2 (group: aphantasic and controls) repeated measures ANOVA on the pupil diameter during the 5-s perceptual period of the task (see Figure 1A for task timeline). There was no main effect of imagery group F(1, 58) = 1.15, p = 0.29 and no significant interactions between imagery groups and any other factor (all p > 0.22, see Figure 3—figure supplement 2). These findings suggest the observed pupil responses during the imagery period of the task is unlikely to be a carry-over effect of the previous sensory response to perceived images.
Pupil size has been shown to depend on eye position (Drewes et al., 2014; Gagl et al., 2011) and the preparation to make a saccade to an upcoming image (Jainta et al., 2011; Mathôt et al., 2015; Wang et al., 2018). Pupil modulation and eye position are also both controlled by largely overlapping circuitry (Wang and Munoz, 2018). It could then be the case that group differences in eye position or saccades (either while viewing or imagining the triangles) may explain our data. To assess how eye movements and position (eccentricity) might be related to our findings, we analysed both eye position and saccades made while viewing and imagining the images, to see if these differed as a function of group. Eccentricity was extracted using the ‘saccades’ package in R (von der Malsburg, 2015) and saccades were detected using a velocity-based algorithm (Engbert and Kliegl, 2003) using the same R package. There were no significant differences between the groups for the number of saccades made during perception or imagery of the stimuli (see Figure 3—figure supplement 4 and Figure 3—figure supplement 5). There were also no differences in mean eccentricity values when comparing the two groups (Figure 3—figure supplement 3), and no correlation with eccentricity and the pupillary light response (Figure 2—figure supplement 3), binocular priming (Figure 2—figure supplement 4), or vividness ratings (Figure 2—figure supplement 5), suggesting that differences in fixation or eye movements between the two groups is unlikely to drive the observed group differences in regard to the mental imagery pupillary light response.
Taken together these data from the general population and aphantasic individuals suggest that it is the content and ability to form vivid visual images, not the voluntary attempt to do so or the semantic content, that is driving the imaginary pupillary light response, providing the first evidence that these pupil changes are due to the sensory strength of imagery content and are not driven by higher-level semantic content.
Discussion
Our results provide novel evidence that our pupils respond to the vividness and strength of a visual image being held in mind, the stronger and more vivid that image, the greater the pupillary light response. Our data provide the first evidence linking the pupil response to strength and vividness of imagery, not only between individuals, but also within an individual as imagery vividness fluctuates from moment to moment (Dijkstra et al., 2017; Pearson et al., 2011; Rademaker and Pearson, 2012). Finally, we show that, as a group, there is no evidence of this pupil response in individuals without mental imagery (aphantasia).
How might the content of mental imagery be driving the pupillary light response? One interpretation of these findings is that this imagery pupillary response is a by-product of the top-down modulation of midbrain-level visual circuitry (pretectal olivary nucleus, superior colliculus; Joshi and Gold, 2020), which occurs when imagining vividly, resulting in these regions interpreting this modulation as coming from external or afferent stimuli, and responding accordingly (Larsen and Waters, 2018; Schwalm and Rosales Jubal, 2017). In this case, the pupil would be responding to imagined luminance in much the same way that it responds to retina-based light sources. This is consistent with current data and models proposing shared mechanisms between visual imagery and perception (Dijkstra et al., 2017; Dijkstra et al., 2019; Ganis et al., 2004; Naselaris et al., 2015; Xie et al., 2020) and the idea that visual imagery functions much like a weak version of afferent perception (Pearson, 2019), supporting the idea that the stronger or more vivid an individual’s imagery is, the more ‘perception like’ their imagery is.
An alternative mechanistic account might be that pupil diameter is encoded along with the original visual information for example bright object, and hence is replayed during memory decoding to form the mental image. This would be in a similar manner to theories proposing a functional role of eye movements during imagery generation from memory (Wang et al., 2020). It will be up to future work to uncover the exact mechanist account of imagery induced pupil changes.
Here we also provide the first objective physiological evidence of an extreme lack of visual imagery in aphantasic individuals. Aphantasia has largely been defined using subjective means (Dawes et al., 2020; Jacobs et al., 2018; Pounder et al., 2018; Zeman et al., 2015, but see Keogh and Pearson, 2018). Accordingly, people have remained sceptical about its true nature and possible psychogenic basis (de Vito and Bartolomeo, 2016). Our data demonstrate that using a non-visual strategy (no imagery in aphantasia) to think about bright and dark objects does not induce a pupillary light response. These data simultaneously provide strong evidence linking the pupillary light response to mental imagery, as well as supporting the behavioural work showing that aphantasic individuals indeed lack visual sensory imagery (Keogh and Pearson, 2018). Because the pupillary light response is involuntary (Bouffard, 2019), we can consider these findings as an unbiased neurophysiological measure of aphantasia. Not only do these data show that pupillary light response can be an objective index of imagery strength in studies of imagery in general populations, our data also provide a new low-cost objective measure for aphantasia that is uniquely based on a physiological mechanism and not reliant on self-report.
Could a lack of active engagement during imagery explain the aphantasia results? Put another way, are such participants refusing to imagine (de Vito and Bartolomeo, 2016)? We think this is highly unlikely as pupil size did increase as a function of set size for aphantasic individuals when attempting imagery, as has previously been shown in the general population, demonstrating the typical relationship between cognitive effort or arousal and pupil dilation (Kahneman and Beatty, 1966; van der Wel and van Steenbergen, 2018). This demonstrates active task engagement, suggesting that aphantasic individuals were not simply ‘refusing’ to actively participate in the task due to demand characteristics or a belief that they are unable to imagine (de Vito and Bartolomeo, 2016).
Further, we ran Bayesian one-sample t-tests on the binocular rivalry and pupillary light response difference scores (see Figure 2) comparing their performance to chance to see if there was any evidence they were performing significantly below chance. We found no significant evidence of below chance performance for either group on either the binocular rivalry or pupillometry imagery tasks (see Figure 2—figure supplement 7). Taken together, with the set-size pupillary effect we observed in our aphantasic participants, it seems unlikely that our aphantasic individuals were not engaging in the tasks. However, we cannot fully rule out this possibility. Further, there was no significant evidence of an abnormal pupillary response in our aphantasic cohort when viewing images, thus it is likely the lack of an imaginary pupillary light response is due to their lack of visual imagery. It also reveals that regardless of what imagery strategy aphantasic participants are implementing (e.g. propositional, spatial, language-like) to recall information about the shapes, they require greater cognitive effort to simultaneously maintain a larger number of shapes in their mind.
One limitation of our study is we did not include catch trails in our pupillometry task, that is we did not include trials where we asked participants to report on what image they had been asked to imagine. We did however include catch trials in our binocular rivalry task through presenting mock binocular rivalry trials. If aphantasic participants are showing a response bias we would expect see a reduction in these mock priming trials when compared to the control population, which we did not find (see Figure 2—figure supplement 6). Adding catch trials to future experiments, in addition to set-size manipulations, may help to further confirm participant engagement. However, adding a simultaneous memory component to the task may lead some subjects to use a non-visual imagery strategy and as such, a reduction or dilution of the pupillary light response (see Pearson and Keogh, 2019). Future studies of visual imagery, and even more importantly when investigating aphantasia, should aim to include appropriate positive controls that allow for the identification of task engagement even when an individual doesn’t have visual imagery. This will allow researchers to exclude the alternate explanation that those individuals who do not show evidence of imagery are not just refusing to imagine or not completing the task correctly.
Another possible explanation of our results is that perceptual pupillary light responses are lingering throughout each trial and driving the observed imagery pupil response. If this is the case, then pupil responses during perceptual viewing and imagery should be correlated, however we did not find any such correlations (see Figure 2—figure supplement 1). Further, when directly comparing the perceptual pupil responses between the general population and aphantasic individuals, there was no main effect of group or interaction between group and stimuli brightness or set size (see Figure 3—figure supplement 2). This demonstrates that there is no significant difference in the perceptual pupillary responses between the two groups, making it unlikely that aphantasic individual’s lack of an imagery pupillary response is due to a lack of perceptual response. Finally, we also asked participants if they perceived any after images during the imagery period and any participants who reported they did were excluded from the study. Taken together, these results suggest that it is unlikely that the pupillary response to perceptually viewing the images is driving our observed imagery pupillary responses, and the lack thereof in the aphantasic individuals. Instead, it appears the pupillary light response during the visual imagery period reflects the wilful generation of imagery in the mind’s eye of those who experience visual imagery. This is further substantiated by the strength of visual imagery (measured using the binocular rivalry paradigm) correlating with the imagery pupillary light reflex, but not the perceptual pupillary light reflex (see Figure 2B and Figure 2—figure supplement 2).
We also found that in the imagery task, higher within-trial reports of vividness are reflected by greater pupillary light responses (within-subjects effects; see Figure 1D). This indicates that participants were able to accurately evaluate the vividness of individual episodes of imagery in comparison to other vividness episodes on previous trials. However, average vividness ratings did not correlate with their pupil-difference scores, that is, participants who gave higher vividness ratings on average did not necessarily have increased pupil light responses in response to imagery (between-subjects effects; see Figure 1—figure supplement 2). Participant’s scores on the VVIQ also did not correlate with pupil-difference scores (between-subjects effects; see Figure 1—figure supplement 3). This suggests that participants might have difficulties in accurately reporting their strength of sensory visual imagery on an absolute scale (i.e. from ‘no image’ to ‘as vivid as perception’), and brings into question the reliability of these subjective measures of imagery and highlights the utility of using objective or online (i.e. in a task), and less trait-like measures when studying visual imagery.
Recent studies have shown pupil size is also modulated by the content of visual working memory (Blom et al., 2016; Hustá et al., 2019; Zokaei et al., 2019). It is interesting to note here that previous work has shown that imagery has been implicated as one mnemonic that can be used to retain information in mind during visual working memory tasks (Albers et al., 2013; Keogh and Pearson, 2011; Keogh and Pearson, 2014; Keogh and Pearson, 2017). This highlights the possibility that it is imagery, being used as a mnemonic strategy, that is driving the pupillary light response observed in visual working memory experiments (Pearson and Keogh, 2019). Although many participants report using a visual imagery strategy during these tasks, some participants report using a non-visual imagery strategy when remembering visual information, and recent work demonstrates that aphantasic individuals can perform traditional visual working memory tasks just as well as control populations (Keogh et al., 2021b). Measuring the pupillary light response in aphantasic individuals, and those who report not using an imagery strategy, while performing classic visual working memory tasks may help to further elucidate these differences in cognitive strategy use in a more objective manner.
One limitation that is important to note here is that our aphantasic sample contained a relatively small sample (18 participants) due to the relative rarity of this condition. Further our two samples were not age matched, which may have affected our results, however seeing as there was no difference between the two groups for the perceptual pupillary light response, we think this is unlikely to be driving our findings. Future studies should aim to replicate and extend these findings with a larger group of aphantasic individuals and age matched controls.
To conclude, the present study demonstrates that the pupillary light response can be used as a physiological index of individual differences in the sensory and phenomenological strength of visual imagery, including the lack of visual imagery – aphantasia. Combining this measure with the binocular rivalry paradigm in favour of subjective alternatives will increase the reliability and objectivity of imagery test batteries and may lead to the development of more congenial theories of the mind’s eye.
Materials and methods
Participants
Fifty-six psychology students with a mean age of 19.8 years (range 18–31, 27 females) were recruited for the study and participated for course credit. We aimed to obtain analysable data from a minimum of 40 participants, which should be a large enough sample to identify a strong positive correlation between pupil dilation and imagery, which is what we would expect if imagery content were driving the previously observed imagery pupillary light response (g*Power effect size = 0.5, α = 0.05, β = 0.95). Fourteen of these participants were excluded from data analysis for not meeting a priori criteria (see Exclusion criteria), leaving 42 participants in the final general population sample.
The aphantasic individuals come from a rare population and for this reason we did not run a specific power analysis but aimed to collect a minimum of 15 participants. We had nineteen aphantasic individuals agree to participate in the study with a mean age of 35.8 years (aged 18–54, 12 females). One of these individuals was excluded from data analysis for not meeting a priori criteria (see Exclusion criteria), leaving 18 in the final sample. These participants had all contacted the lab regarding their aphantasia and asked to participate in our research. They were all reimbursed $20 AUD per hour for their participation. All participants had normal or corrected to normal vision (i.e. glasses or contacts). Both experiments were approved by the UNSW Human Research Ethics Advisory Panel (HREAP-C 3182).
Apparatus
Apparatus stimuli in all experiments were presented on an LCD display monitor (Dell UltraSharp U2419H) with 60 Hz refresh rate and a 1920 × 1080 resolution. Luminance values of all stimuli were measured using a Konica Minolta chroma meter (CS-100A). Participants placed their chin on a chin rest throughout the experiment to maintain fixation at a distance of 57 cm from the monitor 13 and to limit head movements. The tasks were performed in a blackened room to eliminate any possible fluctuations in ambient light.
In the pupillary response task, pupil sizes and eye movements were recorded using head mounted eye-tracking glasses (Pupil, Pupil Labs GmbH, Berlin, Germany) (Kassner et al., 2014). Pupil diameter of participants’ right eye was continuously sampled at 200 Hz throughout the task. A pupil detection 3D algorithm locates the dark pupil in the infrared illuminated eye camera image, thus recording capabilities are not compromised by an absence of room lighting. Pupil diameter is then scaled to millimetres (mm) based on mean anthropomorphic eyeball diameter and corrected for perspective. The algorithm does not depend on corneal reflection, and is compatible with users who wear contact lenses and most eyeglasses (Kassner et al., 2014).
A second camera mounted on the glasses continuously recorded participants’ field of view. Footage from this camera was subsequently assessed to ensure fixation on the computer monitor was maintained throughout the task. The experiment was designed using MATLAB (version R2017b). ZeroMQ plug-ins were used for cross-communication between eye-tracking and stimulus presentation platforms (Akgul, 2013). Pupil data were recorded with Pupil Capture v.1.10.20 (Pupil Labs) installed on an ASUS (GL502V) PC (Windows 10).
In the binocular rivalry task, participants wore red-green anaglyph glasses to ensure rivalrous stimuli were presented to left and right eyes in isolation. Responses of 1, 2, or 3 on a keyboard were used by participants to indicate which image dominated their perception during binocular rivalry (1 for green; 3 for red; 2 for perceptually mixed green and red).
Stimuli
Request a detailed protocolFor the pupillary response task, 32 achromatic shape stimuli were created for participants to perceive and then later imagine in their absence, across 32 trials. The stimuli were evenly divided based on a 2 × 2 factorial design, belonging to one of two luminance conditions (‘Bright’ or ‘Dark’) and one of two set-size conditions (‘Set-Size-One’ or ‘Set-Size-Four’). Shapes belonging to the Bright condition were either white with a luminance of 117 cd/m2 or light grey with a luminance of 65 cd/m2. Shapes in the Dark condition were black (1 cd/m2) of dark grey (9 cd/m2). Set-Size-One stimuli consisted of a single equilateral triangle with 12.5 cm sides, subtending 12.5° of visual angle. Set-Size-Four stimuli consisted of an arrangement of four smaller equilateral triangles with a total surface area and luminance equal to that of the corresponding Set-Size-One triangles (see Figure 1—figure supplement 1 for illustration of all stimuli). Stimuli were also uniquely orientated at either 0°, 90°, 180°, or 270° (e.g. four Set-Size-One black triangles, each with a different orientation. See Figure 1—figure supplement 1 for examples all possible shape orientations). This ensured that all 32 stimuli were unique and participants and were encoding information about a new stimulus on each trial, therefore avoiding the use of long-term memory. Set-Size-Four stimuli therefore subtended either 10.8° or 18.9° of visual angle depending on their orientation.
All stimuli were presented on a grey background screen with a luminance of 26 cd/m2. This same level of background luminance was used during measurement of baseline and imagery phases. A fixation cross on a black background with a luminance of 1 cd/m2 was presented during the resting phase of each trial. All stimuli were created in MATLAB, using the Psychophysics Toolbox 3 extensions (Brainard, 1997).
For the binocular rivalry task, sinusoidal luminance modulated Gabor patterns were used as rivalrous stimuli; vertical-green (CIE chromaticity coordinates: x = 0.275, y = 0.590) and horizontal-red (CIE chromaticity coordinates: x = 0.492, y = 0.372), both with a mean luminance of 8.35 cd/m2 and 7.1° of visual angle. In each trial, both patterns were presented at the same time around a fixation point at the centre of a black background screen. Mock rivalry stimuli (a single 15 Gabor pattern spatially divided into half vertical-green and half horizontal-red) were used on 12.5% of trials to measure the influence of decisional bias or lack of attention to the task. More details on the binocular rivalry task can be found in Keogh and Pearson, 2014.
All participants also complete the VVIQ (Marks, 1973) to get a self-report measure of trait imagery vividness (see Figure 2—figure supplement 8 for VVIQ data for general population and aphantasic individuals).
Procedure
Pupillometry imagery experiment timeline
Request a detailed protocolEach trial began with the presentation of a white fixation cross at the centre of a grey screen (baseline) for 1 s. An image was then presented at the centre of this grey screen for 5 s (either one or four triangles of varying brightness, see Figure 1—figure supplement 1 for illustrations of all stimuli). Participants were instructed to focus on the stimuli during this time and memorize its size, orientation, and level of brightness. Next, a black screen with a white fixation cross was presented for 8 s, allowing the perceived after-image to completely fade and pupils to dilate back to equivalent resting levels. The grey baseline screen was then presented again for 6 s. During this time, participants were cued (via two auditory beeps) to actively imagine the stimuli observed previously during that trial. Lastly, participants were prompted to report the vividness of their imagery during those previous 5 s on a scale of 1–4 (1 being ‘not vivid at all – no shape appeared in imagery’; 4 being ‘very vivid – almost like seeing it’) via key response.
Binocular rivalry paradigm
Request a detailed protocolParticipants were cued to imagine either a red or green Gabor pattern prior to binocular rivalry with the letter ‘R’ or ‘G’ (750 ms). Participants then imagined the image for 6 s, after which they were presented with the binocular rivalry display (750 ms) and were asked to indicate which image was dominant (see Figure 2). Trials where participants reported seeing the pattern they were cued to imagine as dominant were denoted as ‘primed’ trials. Participants completed 2 blocks of 48 trials resulting in a total of 96 trials in total (84 real and 12 mock trials). The number of primed trials divided by the total number of trials (excluding mock trials and mixed percepts) was used to calculate a percent primed score for each participant. Mock trial priming was calcuated by giving a value to each mock trial as either 0 (reporting the catch trial as the opposite colour to that primed), 50 (reporting the catch trial as being mixed), or 100% (reporting the catch trial to be the same as the cued image) (Figure 2—figure supplement 6). These values are then averaged to get a priming value where 50% indicates no bias, while higher values indicate a bais towards reporting the mock trails as being the same as the imagined image, while negative numbers indicate a bias towards reporting the oppoiste image to that which was imagined.
Exclusion criteria
Request a detailed protocolOf the 56 participants recruited for the general population sample, 14 in total were excluded from data analysis due to not meeting a priori criteria.
Pupillary response task exclusions: eight participants were excluded because more than 50% of their pupil data points were below the pupil detection algorithm confidence value of 0.6, provided by the Pupil Capture system. This cut-off point was derived prior to data collection and is the recommended cut-off point for obtaining accurate pupil size data (Pupil Labs). Three participants were excluded due to reporting (during systematic post-task questioning) seeing after-images of the shape stimuli for longer than the 8 s black screen presentation (i.e. seeing after-images during the imagery phase of trials), because pupil size is known to be influenced by the induced compensatory light perception of an after-image (Tsujimura et al., 2003).
Binocular rivalry task exclusions: three participants were excluded due to having mock rivalry priming >66.67% (more than one incorrect response on the mock trials), which indicated either an influence of decisional bias or lack of attention to the task. An a priori cuff-off point of scoring both below 65% priming on the binocular rivalry task and below 32 on the VVIQ was used to exclude participants who potentially did not have visual imagery (i.e. may be aphantasic). No participants fell below this combined cut-off point thus none were excluded on this basis.
Of the 19 participants recruited for the aphantasic population, 1 was excluded from data analysis because more than 50% of their pupil data points were below the pupil detection algorithm confidence value of 0.6, given by the Pupil Capture system. All participants scored below both of the a priori cut-off points of 32 on the VVIQ and 65% on the binocular rivalry task, therefore, no participants were excluded due to this criterion.
Data analysis
Request a detailed protocolFor the pupillary response task, cubic spline interpolation was used to estimate pupil diameter during periods where subjects’ pupils were occluded due to blinking (in accordance with Mathôt et al., 2013). Artefacts in the pupil data were then smoothed using a moving average Hanning window (Kret and Sjak-Shie, 2019). Individual trials in which mean pupil diameter while passively viewing the grey baseline screen was lower than 2 mm or higher than 8 mm were excluded (N(total trials from whole sample) = 8) as values outside this range are unnatural pupil sizes and were clear outliers based on inspection of participants’ pupil-baseline histograms (Mathôt et al., 2018). Trials were averaged to form condition-specific pupil diameter waveforms to represent change in pupil size over time. Mean pupil diameter values during imagery in each trial were baseline corrected using a within-trial baseline subtraction approach (Mathôt et al., 2018) (i.e. subtracted from mean pupil diameter during 0.5 s prior to stimulus perception onset) to account for temporal shifts in pupil size across the experimental session due to fatigue (Morad et al., 2000). A two-way repeated measures ANOVA was used to compare Dark and Bright means during perception and imagery within both set-size conditions. ‘Pupil-difference’ scores were calculated by subtracting Dark condition means from Bright condition means of the corresponding set size for comparison with binocular rivalry percent primed scores. Pupil-difference scores were also separated based on the discrete within-trial vividness ratings to assess metacognition and whether pupil size changes in response to imagery were reflective of subjects’ own experience of vividness of visual imagery.
In the binocular rivalry task, trials where participants reported seeing the pattern they were cued to imagine as dominant in the subsequent binocular rivalry display were denoted as ‘primed’ trials. The number of primed trials divided by the total number of trials (excluding mock trials and mixed percepts) was used to calculate a percent primed score for each participant. Participants’ percent primed scores in binocular rivalry were correlated with their pupil-difference scores (both Set-Size-One and Set-Size-Four) to assess potential for the pupillary response task to measure individual variability in visual imagery strength.
The LMEs were run in R (R Development Core Team, 2018) using the lme4 package and ANOVA’s and ANCOVA were run in SPSS v.25.0 (IBM Corp. Released, 2017 ). For the linear mixed-effects models set size (1 or 4) and vividness ratings (1, 2, 3, and 4) were entered into the model as fixed effects. As random effects intercepts for subjects were entered into the model. p values were obtained by likelihood ratio tests of the full model with vividness included vs. the model without vividness included.
Bayesian statistics were used to determine whether null findings can be interpreted as evidence for an absence of effect (Dienes, 2014). We used Bayesian repeated measure ANOVA (within-subject effect: set size; between-subject effect: group) to compare the control and aphantasia groups as well as Bayesian one-sample t-tests to compare each group with H0, defined as the absence of effect. All Bayesian analysed were performed with JASP (Version 0.10.2).
Data availability
Figure 1 - Source Data 1& 2, Figure 2 - Source Data 3, and Figure 3 - Source Data 4 contain the numerical data used to generate the figures.
References
-
BookZeroMQ: Use ZeroMQ and Learn How to Apply Different Message PatternsPackt Publishing.
-
Smaller Primary Visual Cortex Is Associated with Stronger, but Less Precise Mental ImageryCerebral Cortex (New York, N.Y 26:3838–3850.https://doi.org/10.1093/cercor/bhv186
-
Attention to bright surfaces enhances the pupillary light reflexThe Journal of Neuroscience 33:2199–2204.https://doi.org/10.1523/JNEUROSCI.3440-12.2013
-
Pupil constrictions to photographs of the sunJournal of Vision 13:8.https://doi.org/10.1167/13.6.8
-
The pupillary light response reflects encoding, but not maintenance, in visual working memoryJournal of Experimental Psychology. Human Perception and Performance 42:1716–1723.https://doi.org/10.1037/xhp0000252
-
The functional effects of prior motion imagery and motion perceptionCortex; a Journal Devoted to the Study of the Nervous System and Behavior 105:83–96.https://doi.org/10.1016/j.cortex.2017.08.036
-
Refusing to imagine? On the possibility of psychogenic aphantasiaCortex; a Journal Devoted to the Study of the Nervous System and Behavior 74:334–335.https://doi.org/10.1016/j.cortex.2015.06.013
-
Using Bayes to get the most out of non-significant resultsFrontiers in Psychology 5:781.https://doi.org/10.3389/fpsyg.2014.00781
-
Vividness of Visual Imagery Depends on the Neural Overlap with Perception in Visual AreasThe Journal of Neuroscience 37:1367–1373.https://doi.org/10.1523/JNEUROSCI.3022-16.2016
-
Shared Neural Mechanisms of Visual Perception and ImageryTrends in Cognitive Sciences 23:423–434.https://doi.org/10.1016/j.tics.2019.02.004
-
Microsaccades uncover the orientation of covert attentionVision Research 43:1035–1045.https://doi.org/10.1016/s0042-6989(03)00084-1
-
BookEffect of Age on Pupillary Light Reflex: Evaluation of Pupil Mobility for Clinical Practice and ResearchElectromyography and Clinical Neurophysiology.
-
Systematic influence of gaze position on pupil size measurement: analysis and correctionBehavior Research Methods 43:1171–1181.https://doi.org/10.3758/s13428-011-0109-5
-
Brain areas underlying visual mental imagery and visual perception: an fMRI studyBrain Research. Cognitive Brain Research 20:226–241.https://doi.org/10.1016/j.cogbrainres.2004.02.012
-
BookThe Role of Imagery in Navigation: Neuropsychological EvidenceIn: Mast F, Jäncke L, editors. Spatial Processing in Navigation, Imagery and Perception. Boston, MA: Springer. pp. 17–28.https://doi.org/10.1007/978-0-387-71978-8
-
The pupillary light response reflects visual working memory contentJournal of Experimental Psychology. Human Perception and Performance 45:1522–1528.https://doi.org/10.1037/xhp0000689
-
SoftwareIBM SPSS Statistics version 25.0IBM Corp, Armonk, NY.
-
Visual working memory performance in aphantasiaCortex; a Journal Devoted to the Study of the Nervous System and Behavior 105:61–73.https://doi.org/10.1016/j.cortex.2017.10.014
-
The pupil reflects motor preparation for saccades – even before the eye starts to moveFrontiers in Human Neuroscience 5:97.https://doi.org/10.3389/fnhum.2011.00097
-
Pupil Size as a Window on Neural Substrates of CognitionTrends in Cognitive Sciences 24:466–480.https://doi.org/10.1016/j.tics.2020.03.005
-
Pupil diameter and load on memoryScience (New York, N.Y.) 154:1583–1585.https://doi.org/10.1126/science.154.3756.1583
-
ConferencePupil: An open source platform for pervasive eye tracking and mobile gaze-based interactionUbiComp 2014 - Adjunct Proceedings of the 2014 ACM International Joint Conference on Pervasive and Ubiquitous Computing.https://doi.org/10.1145/2638728.2641695
-
The blind mind: No sensory visual imagery in aphantasiaCortex; a Journal Devoted to the Study of the Nervous System and Behavior 105:53–60.https://doi.org/10.1016/j.cortex.2017.10.012
-
Attention driven phantom vision: measuring the sensory strength of attentional templates and their relation to visual mental imagery and aphantasiaPhilosophical Transactions of the Royal Society of London. Series B, Biological Sciences 376:20190688.https://doi.org/10.1098/rstb.2019.0688
-
Visual working memory in aphantasia: Retained accuracy and capacity with a different strategyCortex; a Journal Devoted to the Study of the Nervous System and Behavior 143:237–253.https://doi.org/10.1016/j.cortex.2021.07.012
-
Preprocessing pupil size data: Guidelines and codeBehavior Research Methods 51:1336–1342.https://doi.org/10.3758/s13428-018-1075-y
-
The Eye Pupil Adjusts to Imaginary LightPsychological Science 25:188–197.https://doi.org/10.1177/0956797613503556
-
Neuromodulatory correlates of pupil dilationFrontiers in Neural Circuits 1:e21.https://doi.org/10.3389/fncir.2018.00021
-
Pupillary behavior in relation to wavelength and ageFrontiers in Human Neuroscience 10:221.https://doi.org/10.3389/fnhum.2014.00221
-
Visual imagery differences in the recall of picturesBritish Journal of Psychology (London, England: 1953) 64:17–24.https://doi.org/10.1111/j.2044-8295.1973.tb01322.x
-
The pupillary light response reflects eye-movement preparationJournal of Experimental Psychology. Human Perception and Performance 41:28–35.https://doi.org/10.1037/a0038653
-
Pupillary Responses to Words That Convey a Sense of Brightness or DarknessPsychological Science 28:1116–1124.https://doi.org/10.1177/0956797617702699
-
Pupillometry: Psychology, Physiology, and FunctionJournal of Cognition 1:16.https://doi.org/10.5334/joc.18
-
Safe and sensible preprocessing and baseline correction of pupil-size dataBehavior Research Methods 50:94–106.https://doi.org/10.3758/s13428-017-1007-2
-
Pupillography as an objective indicator of fatigueCurrent Eye Research 21:535–542.https://doi.org/10.1076/0271-3683(200007)2111-ZFT535
-
Pupil size variation as an indication of affective processingInternational Journal of Human-Computer Studies 59:185–198.https://doi.org/10.1016/S1071-5819(03)00017-X
-
The functional impact of mental imagery on conscious perceptionCurrent Biology 18:982–986.https://doi.org/10.1016/j.cub.2008.05.048
-
Evaluating the mind’s eye: the metacognition of visual imageryPsychological Science 22:1535–1542.https://doi.org/10.1177/0956797611417134
-
ConferenceNew Directions in Mental-Imagery Research: The Binocular-Rivalry Technique and Decoding fMRI PatternsCurrent Directions in Psychological Science.https://doi.org/10.1177/0963721414532287
-
The human imagination: the cognitive neuroscience of visual mental imageryNature Reviews. Neuroscience 20:624–634.https://doi.org/10.1038/s41583-019-0202-9
-
Redefining Visual Working Memory: A Cognitive-Strategy, Brain-Region ApproachCurrent Directions in Psychological Science 28:266–273.https://doi.org/10.1177/0963721419835210
-
Mental rotation performance in aphantasiaJournal of Vision 18:1123.https://doi.org/10.1167/18.10.1123
-
SoftwareR: A language and environment for statistical computingR Foundation for Statistical Computing, Vienna, Austria.
-
The dynamics of interhemispheric compensatory processes in mental imageryScience (New York, N.Y.) 308:702–704.https://doi.org/10.1126/science.1107784
-
Imagine that: elevated sensory strength of mental imagery in individuals with Parkinson’s disease and visual hallucinationsProceedings. Biological Sciences 282:20142047.https://doi.org/10.1098/rspb.2014.2047
-
Pupil dilation as an index of effort in cognitive control tasks: A reviewPsychonomic Bulletin & Review 25:2005–2015.https://doi.org/10.3758/s13423-018-1432-y
-
Sex hormones predict the sensory strength and vividness of mental imageryBiological Psychology 107:61–68.https://doi.org/10.1016/j.biopsycho.2015.02.003
-
The critical role of mental imagery in human emotion: insights from fear-based imagery and aphantasiaProceedings. Biological Sciences 288:20210267.https://doi.org/10.1098/rspb.2021.0267
-
Lives without imagery - Congenital aphantasiaCortex; a Journal Devoted to the Study of the Nervous System and Behavior 73:378–380.https://doi.org/10.1016/j.cortex.2015.05.019
Article and author information
Author details
Funding
National Health and Medical Research Council (APP1024800)
- Joel Pearson
National Health and Medical Research Council (APP1046198)
- Joel Pearson
National Health and Medical Research Council (APP1085404)
- Joel Pearson
National Health and Medical Research Council (APP1049596)
- Joel Pearson
Australian Research Council (DP140101560)
- Joel Pearson
Human Frontier Science Program (LT000362/2018-L)
- Thomas Andrillon
The funders had no role in study design, data collection, and interpretation, or the decision to submit the work for publication.
Ethics
Informed written consent was obtained from all participants to participate in the experiment and to publish their anonymized data in a journal article. Both experiments were approved by the UNSW Human Research Ethics Advisory Panel (HREAP-C 3182).
Copyright
© 2022, Kay et al.
This article is distributed under the terms of the Creative Commons Attribution License, which permits unrestricted use and redistribution provided that the original author and source are credited.
Metrics
-
- 15,029
- views
-
- 1,337
- downloads
-
- 67
- citations
Views, downloads and citations are aggregated across all versions of this paper published by eLife.
Download links
Downloads (link to download the article as PDF)
Open citations (links to open the citations from this article in various online reference manager services)
Cite this article (links to download the citations from this article in formats compatible with various reference manager tools)
Further reading
-
- Neuroscience
When holding visual information temporarily in working memory (WM), the neural representation of the memorandum is distributed across various cortical regions, including visual and frontal cortices. However, the role of stimulus representation in visual and frontal cortices during WM has been controversial. Here, we tested the hypothesis that stimulus representation persists in the frontal cortex to facilitate flexible control demands in WM. During functional MRI, participants flexibly switched between simple WM maintenance of visual stimulus or more complex rule-based categorization of maintained stimulus on a trial-by-trial basis. Our results demonstrated enhanced stimulus representation in the frontal cortex that tracked demands for active WM control and enhanced stimulus representation in the visual cortex that tracked demands for precise WM maintenance. This differential frontal stimulus representation traded off with the newly-generated category representation with varying control demands. Simulation using multi-module recurrent neural networks replicated human neural patterns when stimulus information was preserved for network readout. Altogether, these findings help reconcile the long-standing debate in WM research, and provide empirical and computational evidence that flexible stimulus representation in the frontal cortex during WM serves as a potential neural coding scheme to accommodate the ever-changing environment.
-
- Neuroscience
Human-specific cognitive abilities depend on information processing in the cerebral cortex, where the neurons are significantly larger and their processes longer and sparser compared to rodents. We found that, in synaptically connected layer 2/3 pyramidal cells (L2/3 PCs), the delay in signal propagation from soma to soma is similar in humans and rodents. To compensate for the longer processes of neurons, membrane potential changes in human axons and/or dendrites must propagate faster. Axonal and dendritic recordings show that the propagation speed of action potentials (APs) is similar in human and rat axons, but the forward propagation of excitatory postsynaptic potentials (EPSPs) and the backward propagation of APs are 26 and 47% faster in human dendrites, respectively. Experimentally-based detailed biophysical models have shown that the key factor responsible for the accelerated EPSP propagation in human cortical dendrites is the large conductance load imposed at the soma by the large basal dendritic tree. Additionally, larger dendritic diameters and differences in cable and ion channel properties in humans contribute to enhanced signal propagation. Our integrative experimental and modeling study provides new insights into the scaling rules that help maintain information processing speed albeit the large and sparse neurons in the human cortex.