Paradoxical neuronal hyperexcitability in a mouse model of mitochondrial pyruvate import deficiency
Figures
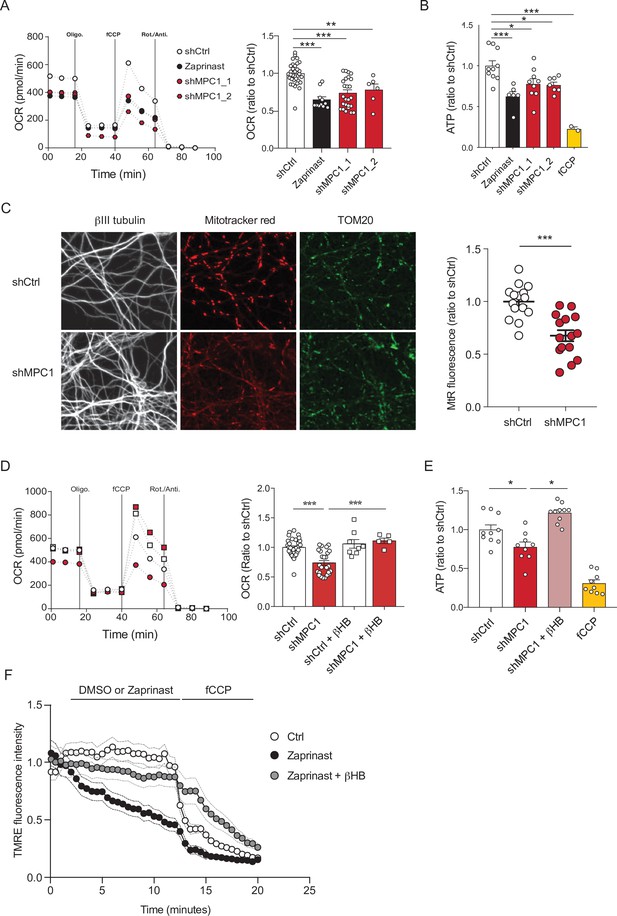
MPC-deficient neurons display defects in mitochondrial respiration and membrane potential.
(A) Profile and quantification of oxygen consumption rates (OCR) cortical neurons expressing either shCtrl, or shMPC1_1 and shMPC1_2 for 7 days, or in the presence of Zaprinast (5 μM, 1 hr). Data were obtained using the Seahorse XF analyzer. Assays were performed in the presence of pyruvate (5 mM) and glucose (5 mM) as carbon sources. Quantification of basal OCR is expressed as ratio of ShCtrl. N = 10,7,9,7 and 2 independent experiments. N = 33,11,25 and 6 independent experiments. One-way ANOVA + Tukey’s post-hoc test (shCtrl vs Zaprinast p = 0.0001, shCtrl vs shMPC1_1 p = 0.0001, shCtrl vs shMPC1_2 p = 0.0013). (B) ATP content in MPC-deficient cortical neurons treated with either shCtrl, Zaprinast or shMPC1_1 and shMPC1_2. fCCP (4 μM) treatment reveals the non-mitochondrial ATP. N = 10,7,9,7 and 2 independent experiments. One-way ANOVA + Tukey’s post-hoc test (shCtrl vs Zaprinast p = 0.0006, shCtrl vs shMPC1_1 p = 0.0223, shCtrl vs shMPC1_2 p = 0.0242, shCtrl vs fCCP p = 0.0001). (C) Mitochondrial membrane potential of MPC-deficient cortical neurons. Neurons were incubated with Mitotracker red (MtR) (1 μM) prior fixation, immunostained for βIII tubulin (neuron) and TOM20 (mitochondria). Quantification of Mitotracker red fluorescence in each βIII tubulin-positive cell (red) was reported to TOM20 signal (green). N = 15 neurons from three independent experiments. Unpaired t test (shCtrl vs shMPC1 p = 0.0001). (D) Profile and quantification of oxygen consumption rates (OCR) in cortical neurons expressing shCtrl or shMPC1_1 for 7 days. Data were obtained using the Seahorse XF analyzer. Assays were performed in the presence of pyruvate (5 mM) and glucose (5 mM) as carbon sources + 10 mM βHB when indicated. Quantification of basal OCR is expressed as ratio of control condition shCtrl. N = 12 independent experiment. One-way ANOVA + Holm Sidak’s post-hoc test (shCtrl vs shMPC1 p = 0.0001, shMPC1 vs shMPC1+βHB p = 0.0002). (E) ATP content in MPC-deficient cortical neurons treated with shCtrl or shMPC1 in presence or absence of 10 mM βHB. fCCP (4 μM) treatment reveals the non-mitochondrial ATP. N = 10, 9, 10, 9 independent experiments. One-way ANOVA + Holm Sidak’s post-hoc test (shCtrl vs shMPC1 p = 0.0145, shMPC1 vs shMPC1+βHB p = 0.0143, shCtrl vs fCCP p = 0.0001). (F) Cortical neurons were incubated with TMRE (50 nM) +/- βHB (10 mM) for 30 min and recorded by live microscopy. Neurons were incubated with DMSO or Zaprinast (5 μM) 2.5 min after the beginning of the acquisition and recorded for 5 min prior fCCP injection. N = 15 independent experiments. One-way ANOVA + Holm Sidak’s post-hoc test (shCtrl vs Zaprinast p = 0.0028, Zaprinast vs Zaprinast+βHB P = 0.0008).
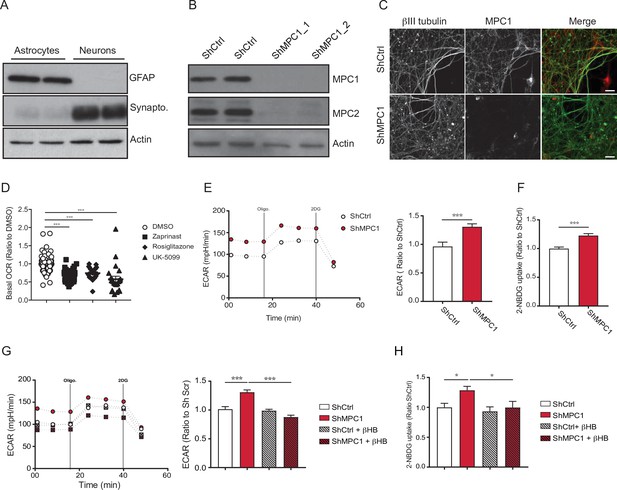
Effects of MPC inhibition in cortical neurons in vitro.
(A) Western blot analysis of lysate from cultured astrocytes (left) and cortical neurons (right). Glial Fibrillary Acidic Protein (GFAP) was used as an astroglial marker, synaptophysin was used as a neuronal marker and actin as a loading control. This analysis shows that the cultures of cortical neurons are devoid of astrocytes. (B) Western blotting of lysates prepared from cortical neurons expressing shCtrl or different shRNAs targeting MPC1 (shMPC1_1 and shMPC1_2). Both MPC1 and MPC2 expression is decreased in neurons expressing shMPC1. Actin was used as a loading control. (C) Immunostaining of cortical neurons expressing shCtrl or shMPC1 for MPC1 (red) and anti-βIII-tubulin (green), a neuronal marker. Note the absence of MPC1 immunostaining in neurons expressing shMPC1 (scale bar: 20 μm) (D) Oxygen consumption rates of cortical neurons cultured in the presence or absence Zaprinast (5 μM), Rosiglitazone (5 μM) and UK5099 (1 μM) for 1 hr, using the Seahorse XF analyzer. Assays were performed in the presence of pyruvate (5 mM) and glucose (5 mM) as carbon sources. Quantification of basal OCR expressed as a ratio to the control condition, DMSO. N > 20 independent experiments. One-way ANOVA followed by Holm Sidak post hoc test (DMSO vs all conditions p = 0.0001). (E) Profile and quantification of the extracellular acidification rate (ECAR) in cortical neurons expressing shCtrl or shMPC1. Left panel: the following compounds were injected at the times indicated by vertical lines: Oligomycin (oligo.), 2-Deoxyglucose (2DG); Right panel: quantification of ECAR is expressed as a ratio to the shCtrl control condition. N = 15 independent experiments. Unpaired t test (shCtrl vs shMPC1 p = 0.0026). (F) 2-NBDG uptake in neurons expressing shCtrl or shMPC1. N = 15 independent experiments. Unpaired t test (shCtrl vs shMPC1 p = 0.0001). (G) Profile and quantification of the extracellular acidification rate (ECAR) in cultures of primary cultures of cortical neurons expressing shCtrl or shMPC1 +/- βHB (10 mM). Left panel: the following compounds were injected at the times indicated by vertical lines: Oligomycin (oligo.), 2-Deoxyglucose (2DG); Right panel: quantification of ECAR is expressed as a ratio to the shCtrl condition. N > 4 independent experiments. One-way ANOVA followed by Holm Sidak post hoc test (shCtrl vs shMPC p = 0.0001, shMPC1 vs shMPC1 + βHB p = 0.0001). (H) 2-NBDG uptake in neurons expressing shCtrl or shMPC1±with βHB (10 mM) 30 min prior experiment when indicated. N > 6 independent experiments. One-way ANOVA followed by Holm Sidak post hoc test (shCtrl vs shMPC p = 0.0377, shMPC1 vs shMPC1 + βHB p = 0.0377).
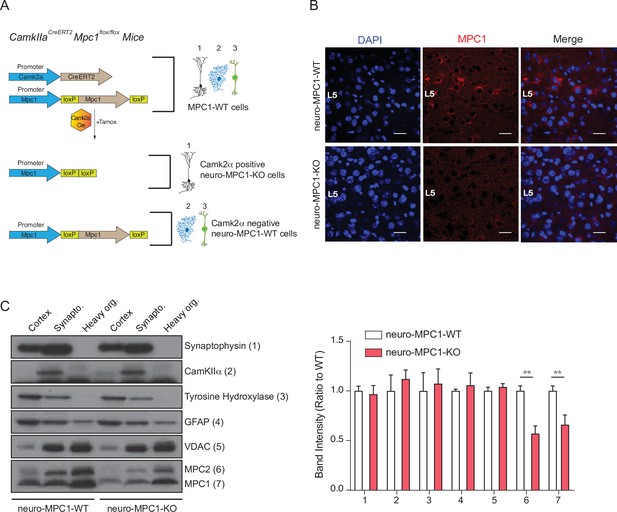
Generation of mice with an inducible deletion of the MPC1 gene in adult glutamatergic neurons.
(A) Strategies used to generate CamKIIaCreERT2-Mpc1flox/flox mice. Upon Tamoxifen injection, expression of the Cre recombinase in CamKIIα glutamatergic neurons drives deletion of the MPC1 gene. These mice are referred to as neuro-MPC1-KO or neuro-MPC1-WT when they are Cre- (1. Glutamatergic neuron; 2. Astrocytes; 3. Inhibitory neuron). (B) Immunostaining of MPC1 (red) in cortical sections from neuro-MPC1-WT and neuro-MPC1-KO mice (scale bar: 100 μm). (C) Western blot analysis of whole cortex, synaptosome lysates and heavy organelles (mainly mitochondria), obtained from brains of neuro-MPC1-WT and neuro-MPC1-KO mice using neuronal (Synaptophysin, tyrosine hydroxylase, CamKIIα) and astroglial markers (GFAP) as well as mitochondrial markers (MPC1, MPC2 and VDAC). Note that synaptosomes are enriched for CamKIIα, a marker of excitatory neurons. Quantification (right panel) shows that except for MPC1 and MPC2, the content of these markers is similar in WT and KO preparations. N = 6 independent neuro-MPC1-WT and neuro-MPC1-KO mice. Mann-Whitney test ((6) neuro-MPC1-WT vs neuro-MPC1-KO p = 0.0286, (7) neuro-MPC1-WT vs neuro-MPC1-KO p = 0.0152).
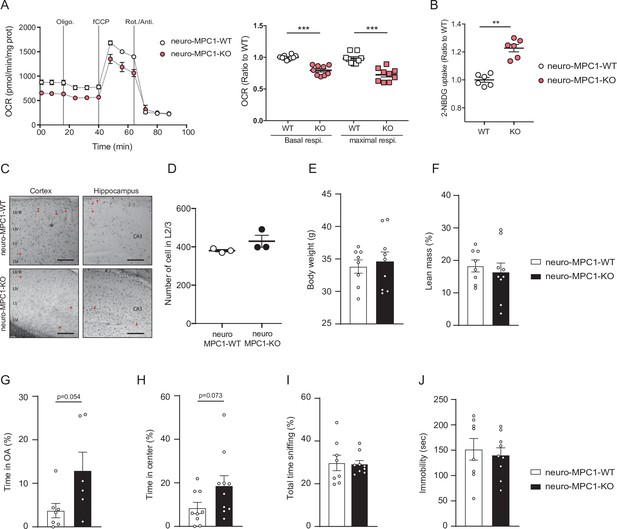
Metabolic effects of MPC deletion in excitatory neurons in vitro and behavioural consequences in neuro-MPC1-WT and KO animals.
(A) Profile and quantification of oxygen consumption rates (OCR) of synaptosomes purified from neuro-MPC1-WT and neuro-MPC1-KO mice. Data were obtained using the Seahorse XF analyzer. Assays were performed in the presence of pyruvate (5 mM) and glucose (5 mM) as carbon sources. Left panel: the following compounds were injected at the time indicated by vertical lines: Oligomycin, fCCP, Rotenone and Antimycin A. Right panel: quantification of basal and maximal OCR is expressed as a ratio of the WT for each condition. N = 10 independent mice for each condition. One-way ANOVA followed by Holm Sidak post hoc test (neuro-MPC1-WT vs neuro-MPC1-KO p = 0.0001). (B) 2-NBDG uptake in synaptosomes purified from neuro-MPC1-WT and neuro-MPC1-KO mice showing an increase of glucose import in neuro-MPC1-KO synaptosome compared to WT. N = 6 independent mice for each condition. Mann Whitney test (neuro-MPC1-WT vs neuro-MPC1-KO p = 0.0022). (C) Coronal sections of cortex and hippocampus P70 of neuro-MPC1-WT and neuro-MPC1-KO mice stained for apoptotic cells using TUNEL assay (TUNEL positive cells are indicated with a red arrowhead)(scale bar: 500 μm). (D) Quantification of total cell number in cortical L2/3 from neuro-MPC1-WT mice and neuro-MPC1-KO. N = 3 independent mice for each condition. Mann Whitney test (neuro-MPC1-WT vs neuro-MPC1-KO p = 0.2061). (E–J) Effect of the inducible MPC1-depletion in body weight (E), fat mass percentage (F), elevated plus maze (EPM) (G), open field (OF) (H), social preference (SP) (I) and forced swim test (FST) (J). N = 6–10 animals/group. Unpaired t test, ((E,F,I,J) neuro-MPC1-WT vs neuro-MPC1-KO p > 0.9; (G) neuro-MPC1-WT vs neuro-MPC1-KO p = 0.054; (H) neuro-MPC1-WT vs neuro-MPC1-KO, p = 0.073).
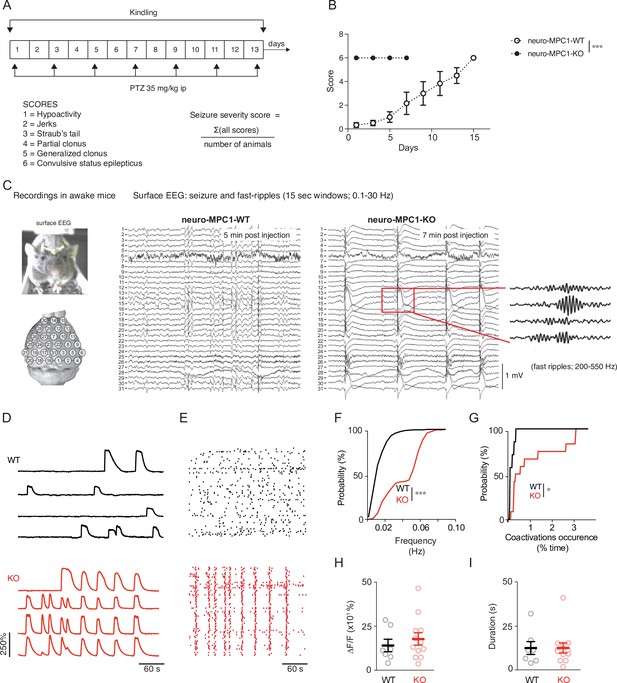
Neuro-MPC1-KO mice are highly sensitive to pro-convulsant drugs and develop acute epileptic-like seizures.
(A) Schematic description of the PTZ kindling protocol. (B) Seizure severity scores reflecting the different clinical symptoms as indicated, obtained for neuro-MPC1-WT or neuro-MPC1-KO. N = 8 independent neuro-MPC1-WT and neuro-MPC1-KO mice. Two way ANOVA (F(7,70)=19, p = 0.0001). (C) Illustration of the recording setups in awake mice indicating the position of surface EEG electrodes and representative example of a seizure recorded in a neuro-MPC1-KO mouse after injection of 35 mg/kg PTZ during surface EEG recordings. The inset shows an example of fast ripples generated during an ictal epileptic discharge. (D–I) GCaMP6S calcium imaging of the CA1 area from hippocampal slices in the presence of Carbachol (50 µM) and PTZ (2 mM). Slices were prepared from WT animals (top, black) or from KO animals with no pre-treatment (bottom, red). (D) Ca2+ sweeps recorded in four representative GCaMP6S-expressing neurons. (E) Raster plots of Ca2+ transient onsets extracted from all recorded neurons in a given slice. (F) Cumulative distribution of the frequency of the calcium events in all the recorded neurons. N = 7, 12 independent experiments. Kolmogorov-Smirnov test (WT vs KO p = 0.0001). (G) Cumulative distribution of the occurrence of neuronal co-activations exceeding chance levels as a function of time N = 7, 12 independent experiments. Kolmogorov-Smirnov test (WT vs KO p = 0.0344). Amplitude (H), and duration (I) of the calcium events recorded in all neurons of the hippocampus. N = 7, 12 independent experiments. Mann-Whitney test (Amplitude: WT vs KO p = 0.5918; Duration: WT vs KO p = 0.9182).
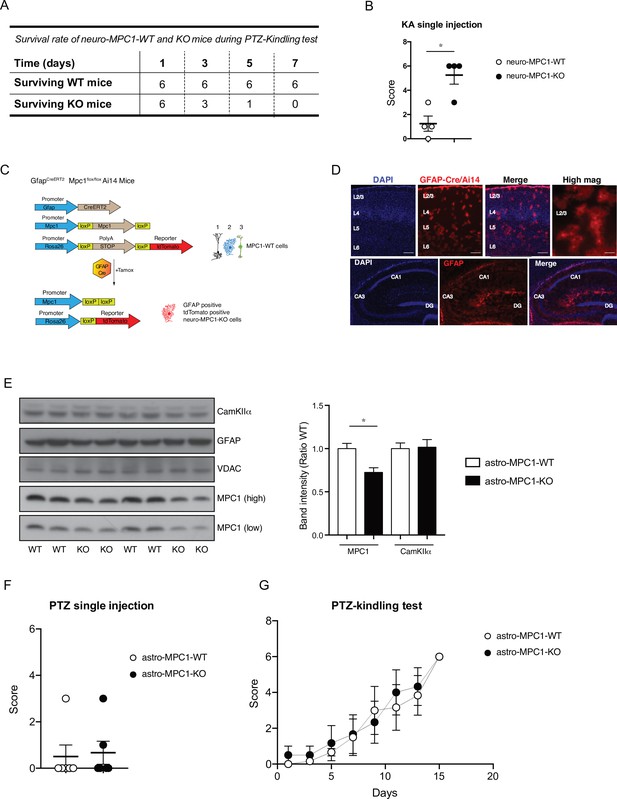
Generation and phenotype of Astro-MPC1-KO mice.
(A) Survival rate of neuro-MPC1-WT and neuro-MPC1-KO mice during the PTZ kindling protocol. neuro-MPC1-KO mice died during the test between the second and fourth injection of PTZ. (B) Neuro-MPC1-KO mice are more sensitive to the effect of kainic acid compared to neuro-MPC1-WT mice. Mice were injected ip with 20 mg/kg kainic acid (KA) and scored clinically. N = 4 independent mice for each condition. Mann Whitney (neuro-MPC1-WT vs neuro-MPC1-KO p = 0.036). (C) Strategy used to generate the astro-MPC1-KO mice. Tamoxifen induces the recombination of MPC1Flox/Flox alleles and the expression of the reporter protein tdTomato (Ai14) specifically in the GFAP positive cells (1. Glutamatergic neuron; 2. Astrocytes; 3. Inhibitory neuron). (D) Coronal sections of astro-MPC1-KO-Ai14 of the cortex (upper panel) and hippocampus (lower panel) showing the expression of tdTomato in the astrocytes. L2/3, L4, L5, and L6 correspond to the respective cortical layers. CA1, CA3 and DG (dentate gyrus) correspond to the respective areas of the hippocampus. Scale bar: 100 μm or 10 μm (high mag). (E) Western blot analysis of brain extract from astro-MPC1-WT and astro-MPC1-KO demonstrates a reduced amount of MPC1 in the KO mice whereas the neuronal marker CamKIIα is equal in WT and KO animals. N = 8 independent mice for each condition. One-Way ANOVA followed Tukey’s post hoc test (astro-MPC1-WT vs astro-MPC1-KO P = 0.0373). (F, G) Astro-MPC1-KO mice behave as astro-MPC1-WT mice in the PTZ-kindling protocol. N = 6 independent mice for each condition. Mann Whitney (astro-MPC1-WT vs astro-MPC1-KO p = 0.999).
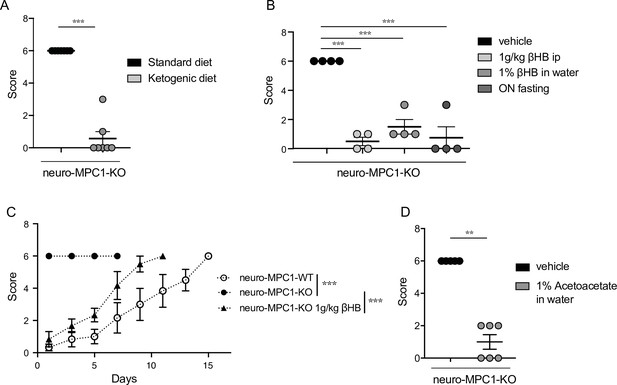
Ketogenic diet prevents the epileptic phenotype of neuro-MPC1-KO mice.
(A) Effect of the ketogenic diet (KD) on PTZ-induced seizure. All neuro-MPC1-KO mice were maintained on the Standard (SD) or ketogenic (KD) diet for 7 days prior to challenge with a single dose of PTZ. Clinical scores were assessed directly following injection. N = 7 independent neuro-MPC1-KO mice. Mann-Whitney (neuro-MPC1-KO SD vs neuro-MPC1-KO KD p = 0.0008). (B) Effects 1% βHB in the drinking water for 7 days, overnight fasting or ip injection of βHB 15 min before administration of PTZ into neuro-MPC1-KO mice. N = 4 independent neuro-MPC1-KO mice. One-way ANOVA + Holm Sidak’s post-hoc test (Vehicle vs all conditions p = 0.0001). (C) Effect of βHB on PTZ-induced seizure: mice were injected ip with 1 g/kg βHB, 15 min before each PTZ injection and scored for clinical symptoms. N = 6 independent mice. Two-way ANOVA + Holm Sidak’s post-hoc test (F(10, 75) = 8, Neuro-MPC1-WT vs neuro-MPC1-KO, neuro-MPC1-KO vs neuro-MPC1-KO + βHB p = 0.0001). (D) Effect of acetoacetate (1% in drinking water) for 7 days on PTZ-induced seizures. Clinical scores were assessed directly following injection. N = 5 and 6 mice for vehicle or acetoacetate IP injected mice, respectively. Mann-Whitney (neuro-MPC1-KO vehicle vs neuro-MPC1-KO Acetoacetate p = 0.0022).
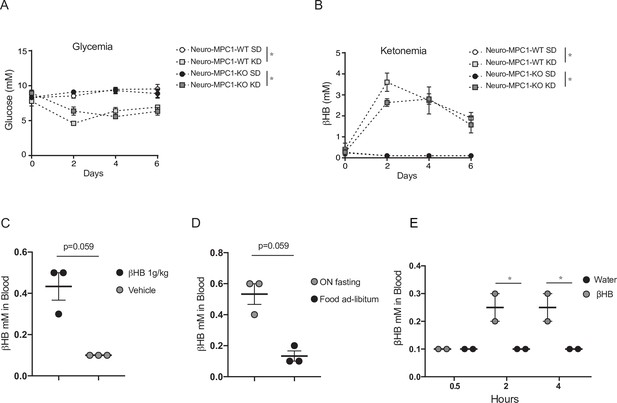
Glycemia and ketonemia measurements in neuro-MPC1-WT and neuro-MPC1-KO mice fed on a ketogenic diet.
(A, B) Glycemia (A) and ketonemia (B) in neuro-MPC1-WT and neuro-MPC1-KO mice maintained on the ketogenic diet (KD) or on a standard diet (SD). N = 4 independent mice for each condition. Two-way ANOVA ((A) F(9,24)=6.629, p = 0.0001, (B) F(9,24)=9.390, p = 0.0001). (C–D) Levels of βHB in the blood from neuro-MPC1-KO mice 15 min following intraperitoneal injection of 1 g/kg of βHB (C) or overnight fasting (D). N = 3 independent mice for each condition. Mann Whitney ((C) Vehicle vs 1 g/kg βHB p = 0.0593; (D) control vs O.N. fasting p = 0.0593). (E) Levels of βHB in the blood from neuro-MPC1-KO mice following addition of βHB 1% in the drinking water for 7 days. N = 2 mice for each condition. One-way ANOVA followed by Holm Sidak’s post hoc test (Water vs βHB 1%, p = 0.0309 for 2 hr and 4 hr).
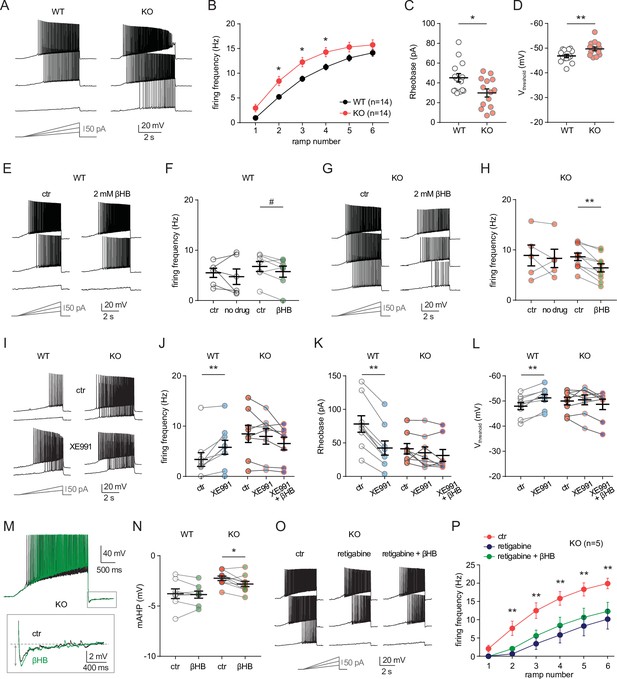
MPC1 deletion increases intrinsic excitability in CA1 pyramidal cells.
(A) Example voltage responses elicited in CA1 pyramidal cells from wild-type (WT) and neuro-MPC1-KO (KO) by injection of current ramps (protocol at the bottom, only three of six ramps displayed). (B) Frequency-current (F–I) relationship of action potential discharges, indicating higher spiking frequency in KO cells (Two-way ANOVA, F(1, 156) 33.43, p < 0.0001). (C) The rheobase was reduced in KO cells (Mann-Whitney test, U = 53.5, p = 0.0406). (D) KO cells exhibited more hyperpolarized threshold potential (unpaired t test, t = 2.856, p = 0.0084). (E) Example traces showing lack of significant changes in WT cell firing after bath application of the ketone body β-hydroxybutyrate (βHB, 2 mM, > 20 min exposure). (F) Average firing frequency elicited by the 3rd ramp of current injections in WT cells in control condition (5 min after whole-cell establishment) and after 20 min of either no drug exposure or βHB application (no drug: paired t test, t = 0.664, p = 0.5362; βHB: paired t test, t = 2.1, p = 0.0804). (G), (H) Example traces and summary graphs indicating significant reduction in KO cell firing after βHB application (no drug: paired t test, t = 0.4691, p = 0.6634; βHB: paired t test, t = 5.339, p = 0.0005). (I) Example traces of cell firing in control and after bath application of XE991 (10 µM) in WT and KO neurons. (J) Average firing frequency elicited by the 2nd ramp of current injections in control and after XE991 application, indicating increased excitability in WT cells (paired t test, t = 3.735, p = 0.0057). XE991 was ineffective in KO cells, in which subsequent application of βHB also failed to modulate excitability (One-way ANOVA, F(1.69, 11.89) = 4.76, p = 0.0347, Holm-Sidak’s multiple comparison p > 0.05). (K) XE991 significantly reduced the rheobase of WT cells (paired t test, t = 11, p < 0.001), but not of KO cells, in which subsequent βHB application was also ineffective (One-way ANOVA, F(1.785, 12.5) = 2.99, p = 0.091). (L) XE991 induced a shift in the threshold potential of WT cells (paired t test, t = 6.001, p = 0.0003), but did not affect KO cells, in which subsequent βHB application was also ineffective (One-way ANOVA, F(1.812, 12.68) = 1.78, p = 0.209). (M) Example traces of KO cell firing elicited by a current ramp (300 pA max amplitude, APs are trimmed) in control and after βHB exposure, with expanded portion at the bottom indicating mAHP measurement. N) Summary graph of mAHP values in WT and KO cells in control and after βHB exposure, indicating significant increase in KO (unpaired t test, t = 2.89, p = 0.0179. (O) Example traces of KO cell firing before and after application of retigabine (10 µM), and subsequent βHB superfusion (2 mM). (P) F-I relationships in KO cells, indicating reduced spiking frequency after retigabine application, with no additional effect of βHB (Two-way repeated measures ANOVA, F(2, 48) = 89.15, p < 0.0001).
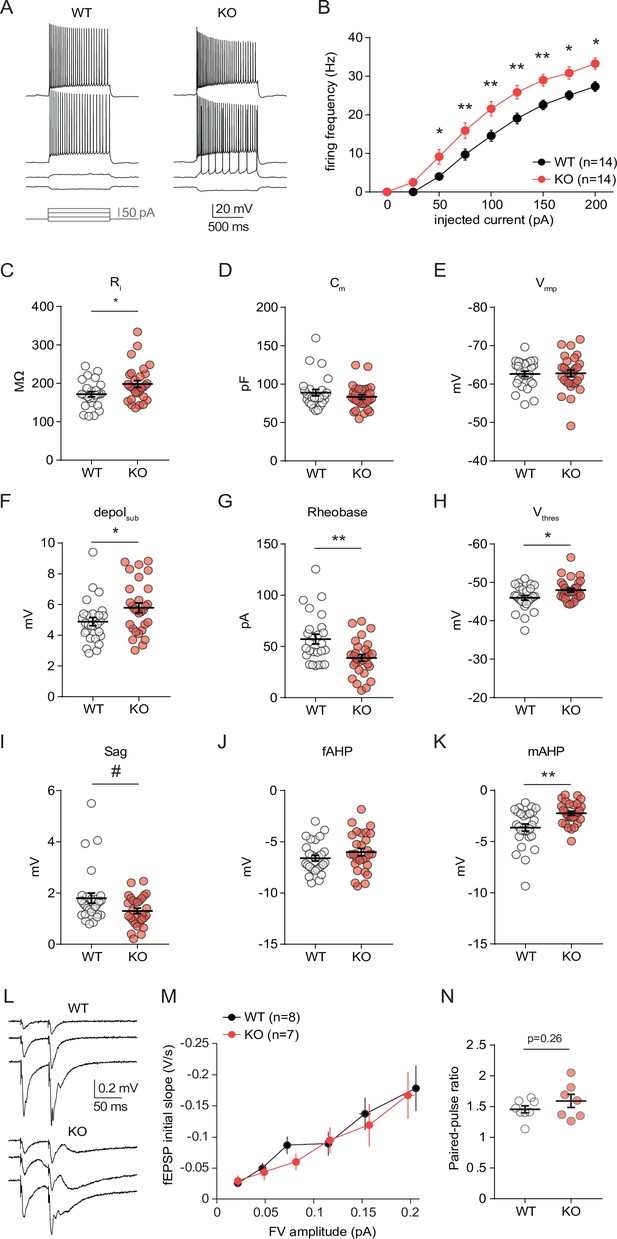
CA1 pyramidal cells from neuro-MPC1-KO mice exhibit altered intrinsic excitability and membrane properties but no changes in glutamatergic transmission.
(A) Example voltage responses elicited in CA1 pyramidal cells from neuro-MPC1-WT (WT) and neuro-MPC1-KO (KO) by injection of current steps (protocol at the bottom, only four stimulations are shown). (B) F-I relationship of action potential discharges evoked by current steps, indicating higher spiking frequency in KO cells (Two-way ANOVA, F(1, 234) = 61.77, p < 0.0001). (C) Input resistance (Ri) (Mann Whitney test, U = 244, p = 0.0247). (D) Membrane capacitance (Cm) (unpaired t test, t = 0.1329, p = 0.895). (E) Resting membrane potential (Vrmp) (Mann Whitney test, U = 302, p = 0.098). (F) Voltage response to a subthreshold current injection of 25 pA (depolsub), confirming higher apparent Ri in KO cells (Mann Whitney test, U = 260, p = 0.0306). (G) Rheobase, calculated as minimal current needed to elicit firing with ramp injections (Mann Whitney test, U = 211, p = 0.0046). (H) Firing threshold (Vthres) (Mann Whitney test, U = 252.5, p = 0.0354). (I) HCN-mediated sag, measured as ΔV between initial and steady state voltage response to a hyperpolarizing current injection of –50 pA (Mann Whitney test, U = 299.5, P = 0.0899). (J) Fast afterhyperpolarization (fAHP) (unpaired t test, t = 0.1315, p = 0.1942). (K) Medium afterhyperpolarization (mAHP), measured as the negative peak of the voltage deflection at the offset of the depolarizing ramp (unpaired t test, t = 3.419, p = 0.0014). In order to compare mAHP after a similar discharge of action potentials, this measure was made using the ramps of maximal amplitude (300 pA), to which WT and KO responded with comparable firing frequencies (Figure 5 B). Data are presented as mean ± SEM. # p < 0.1; * p < 0.05; ** p < 0.01. (L) Example traces representing field excitatory postsynaptic potentials (fEPSP) recorded in CA1 stratum radiatum upon stimulation of the Schaffer collaterals with increased intensity, using a paired-pulse protocol (50ms inter-stimulus interval). (M) Input-output curves depicting fEPSPs slope as a function of the amplitude of the presynaptic volley (FV), showing no significant difference between genotypes (unpaired t test comparing slopes of input-output curves, t = 0.5785, p = 0.5729). (N) Paired-pulse ratio of fEPSPs were no significantly different between genotypes (unpaired t test, t = 1.122, p = 0.29).
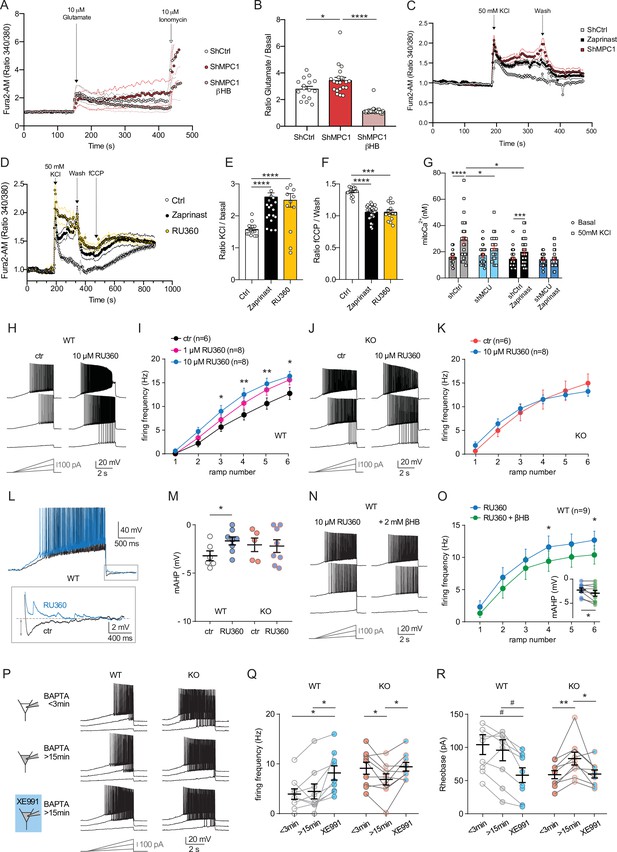
Defect in calcium homeostasis.
(A) Mean fluorescence signal intensity of cortical neurons loaded with Fura2-AM stimulated with 10 μM glutamate (dashed black arrow) prior the addition of ionomycin (red arrow) to reveal the neuronal calcium stock. (B) Graph showing the quantifications of control neurons, MPC-depleted neurons and MPC-depleted neurons+βHB showing an elevated level of cytosolic calcium in MPC-deficient stimulated neurons measured by Fura2-AM. N > 15 neurons per condition from three independent experiments. One-way ANOVA + Holm Sidak’s post-hoc test (shCtrl vs shMPC1 p = 0.0169, shMPC1 vs shMPC1+βHB p = 0.0001). (C) Mean fluorescence signal intensity of cortical neurons loaded with Fura2-AM before and after stimulation with 50 mM KCl (dashed black arrow) for 3 min. Neurons were then washed using a calcium-free medium. (D) Fluorescence signal intensity of control, MPC-deficient, and RU360-treated cortical neurons permeabilized with pluronic acid (0.02%). Neurons were loaded with Fura2-AM, stimulated with KCl 50 mM prior addition of fCCP to reveal the mitochondrial stocks of calcium. (E, F) Quantification of calcium increased upon depolarization (E) ratio of the fluorescence peak after adding KCl to the mean of the 10 first basal measurement) and the amount of mitochondrial calcium released by fCCP (F) ratio of the fluorescence peak after adding fCCP to the lowest point during wash in normal, MPC-deficient neurons and neurons + RU360. N > 13 neurons per condition from three independent experiments. One-way ANOVA + Holm Sidak’s post-hoc test ((E) Ctrl vs Zaprinast p < 0.0001, Ctrl vs RU360 p < 0.0001; (F) Ctrl vs Zaprinast p = 0.0003, Ctrl vs RU360 p < 0.0001). (G) Mitochondrial calcium measurement of AAV-infected cortical neurons expressing mtaequorin. The MCU was inhibited by RNA interference (shMCU), whereas the MPC was inhibited using the MPC inhibitor Zaprinast (5 μM). The bioluminescence values were converved into calcium concentrations according to Bonora et al. Two-way ANOVA + Holm-Sidak post-hoc test (No significant difference could be observed between groups in. basal conditions. shCtrl basal vs shCtrl KCl, p < 0.0001, shMCU basal vs shMCU KCl, p = 0.067, shCtrl+ Zaprinast basal vs shCtrl+ Zaprinast KCl, p = 0.0008, shMCU+ Zaprinast basal vs shMCU+ Zaprinast KCl, p = 0.99, shCtrl KCl vs shMCU KCl, p = 0.012, shCtrl KCl vs shCtrl+ Zaprinast KCl, p = 0.011. (H, I). Example traces and F-I relationship in WT cells with standard intracellular solution and with a solution containing the MCU inhibitor RU360 (one or 10 µM), which increased neuronal firing (10 µM: Two-way ANOVA, F(1, 72) = 26.03, p < 0.0001). (J, K) Lack of RU360 (10 µM) effect on neuronal firing in KO cells (Two-way ANOVA, F(1, 72) = 0.03607, p = 0.8499). (L) Example traces of WT cell firing elicited by a current ramp (300 pA max amplitude, APs are trimmed) in control condition and with RU360, with expanded portion at the bottom indicating mAHP measurement. (M) Summary graph of mAHP values in control condition and with RU360, indicating significant reduction in WT (unpaired t test, t = 2.352, p = 0.0392). (N, O) Example traces and F-I relationship in WT cells infused with RU360 (10 µM) and subsequently exposed to βHB (2 mM, > 20 min exposure), which decreased neuronal firing (Two-way ANOVA, F(1, 28) = 17.69, p = 0.0001) and augmented mAHP (inset, paired t test, t = 2.336, p = 0.0477). (P) Scheme of whole-cell recordings with an intracellular solution containing the calcium chelator BAPTA (10 mM), with representative voltage responses in WT and KO neurons. Cell firing was compared between the first 3 min after whole-cell establishment, after 15 min of recordings, and after subsequent bath-application of XE991 (10 µM). (Q) Average firing frequency elicited by the 3rd ramp of current injections, indicating no altered intrinsic excitability in WT cells upon diffusion of BAPTA into the cytoplasm, but increased firing by subsequent bath application of XE991 (One-way ANOVA, F(1.565, 12.52) = 8.29, p = 0.0074, Holm-Sidak’s multiple comparison *p < 0.05). In KO neurons, diffusion of BAPTA induced a significant decrease in cell firing, which was reversed by subsequent bath-application of XE991 (One-way ANOVA, F(1.553, 12.42) = 4.34, p = 0.0449, Holm-Sidak’s multiple comparison *p < 0.05). (R) Changes in firing frequency were accompanied by a tendency toward decreased rheobase in WT (One-way ANOVA, F(1.254, 10.03) = 8.05, p = 0.014, Holm-Sidak’s multiple comparison #p = 0.0512) and by significant changes in rheobase in KO neurons (One-way ANOVA, F(1.5, 12) = 7.974, p = 0.0093, Holm-Sidak’s multiple comparison *p < 0.5, **p < 0.01).
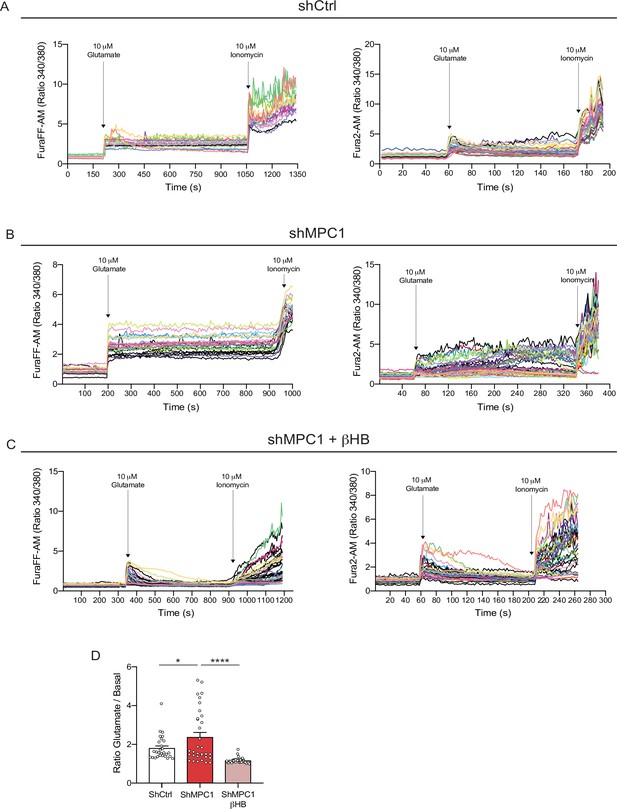
Profiles of calcium imaging in cultured control and MPC1-deficient cortical neurons.
(A) Fluorescence signal intensity from individual control neurons loaded with the calcium probe FuraFF-AM (left) and Fura2-AM (right) stimulated with 10 μM glutamate prior to the addition of ionomycin to reveal the calcium stock of the neurons. (B) Fluorescence signal intensity from individual shMPC1-treated neurons loaded with the calcium probe FuraFF-AM (left) and Fura2-AM (right) stimulated with 10 μM glutamate prior to the addition of ionomycin to reveal the calcium stock of the neurons. (C) Fluorescence signal intensity from individual shMPC1-treated neurons supplemented with 10 mM βHB loaded with the calcium probe FuraFF-AM (left) and Fura2-AM (right) stimulated with 10 μM glutamate prior the addition of ionomycin to reveal the calcium stock of the neurons. (D) Cytosolic calcium quantification using the FuraFF-AM probe in of control neurons, MPC-depleted neurons and MPC-depleted neurons+βHB. N > 15 neurons per condition from three independent experiments. One-way ANOVA + Holm Sidak’s post hoc test (shCtrl vs shMPC1 p = 0.0286, shMPC1 vs shMPC1+βHB p = 0.0001).
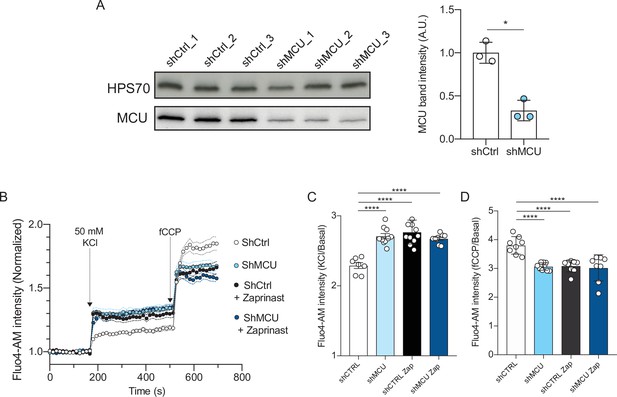
Depletion of the mitochondrial calcium uniporter phenocopies MPC1-inhibition.
(A) Western blotting of lysates prepared from cortical neurons expressing shCtrl or shMCU for 7 days.Band intensities for MCU were expressed relative to the intensity of the loading control HSP70 band (n = 3; Mann Whitney test p = 0.049). (B–D) Fluorescence signal intensity of control, MCU-depleted neurons, and Zaprinast treated neurons. Fluorescence signal intensity of control (shCtrl), MCU-depleted neurons (shMCU), and Zaprinast treated neurons. The MPC inhibitor Zaprinast was used at 5 μM. Neurons were loaded with Fluo-4AM, stimulated with KCl 50 mM prior to addition of fCCP to release mitochondrial calcium. N > 8 wells from three independent experiments. Two-way ANOVA, F(150, 2050) = 14.70, p < 0.0001. (C, D) Quantification of cytosolic calcium upon neuronal stimulation before (C), KCl/basal and after fCCP treatment (D), fCCP/basal. N > 8 wells from three independent experiments. One-way ANOVA + Holm Sidak test (All condition p < 0.0001).
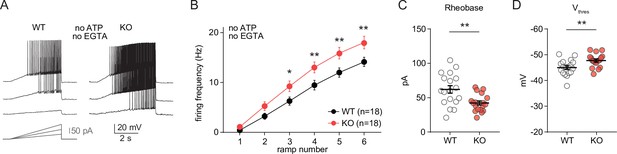
Alterations in excitability of CA1 pyramidal cells from neuro-MPC1-KO mice are preserved under recording conditions that minimize cell dialysis and interference with intracellular ATP levels.
(A) Example voltage responses elicited in CA1 pyramidal cells from wild-type (WT) and MPC1-CamKII-KO (KO) by injection of current ramps (protocol at the bottom, only three of six ramps are displayed). Whole-cell recordings were performed with narrow pipettes (9–10 MΩ) filled with a K-Gluconate-based solution lacking ATP and EGTA. (B) F-I relationship of action potential discharges evoked by current ramps, indicating higher spiking frequency in KO cells (Two-way ANOVA, F(1, 204) = 29.01, p < 0.0001). (C) The rheobase was reduced in KO cells (unpaired t test, t = 3.16, p = 0.004). (D) KO cells exhibited a more hyperpolarized threshold potential (unpaired t test, t = 2.975, p = 0.0054). Data were obtained from two animals per genotype, and are presented as mean ± SEM. Data points represent single observations.
Videos
Spontaneous calcium activity in neuro-MPC1-WT hippocampal slices following PTZ addition.
Spontaneous calcium activity in neuro-MPC1-KO hippocampal slices following PTZ addition.
Tables
Reagent type (species) or resource | Designation | Source or reference | Identifiers | Additional information |
---|---|---|---|---|
Strain, strain background (Mouse, M. musculus) | CamkIIaCreERT2 mice | Jackson laboratory | RRID:IMSR_JAX:012362 | |
Strain, strain background (Mouse, M. musculus) | Mpc1flox/flox mice | gift from Professor Eric Taylor | NA | |
Strain, strain background (Mouse, M. musculus) | Ai14 reporter mice | gift from Professor Ivan Rodriguez | NA | |
Strain, strain background (Mouse, M. musculus) | GfapCreERT2 | gift from Professor Nicolas Toni | NA | |
Strain, strain background (Mouse, M. musculus) | Neuro-MPC1-KO (CamkIIaCreERT2 MPCflox/flox mice) | This article | NA | See materials and methods (p 18,19) |
Strain, strain background (Mouse, M. musculus) | Astro-MPC1-KO(GfapCreERT2 Mpcflox/flox mice) | This article | NA | See materials and methods (p 18,19) |
Cell line (Homo sapiens) | HEK293T | ATCC | RRID: CVCL_0045 | |
Biological sample (Mus musculus) | Cultures of primary cortical neurons | This article, Fauré et al., 2006 | See materials and methods (p 20) | |
Biological sample (Mus musculus) | Organotypic cultures of hippocampus | This article, Marissal et al., 2018 | See Appendix 1 | |
Biological sample (Mus musculus) | Acute hippocampal slices | This article | NA | See materials and methods (p 19,20) |
Recombinant DNA reagent | PLKO.1_shMCU | Sigma Aldrich (NM_001033259) | TRCN0000251263 | |
Recombinant DNA reagent | PLKO.1_shMPC1 | Sigma Aldrich (NM_016098) | TRCN0000005485TRCN0000005487 | |
Recombinant DNA reagent | PLKO.1_shCTRL | Sigma Aldrich (SHC016) | ||
Recombinant DNA reagent | AAV-GCaMP6s | University of Pennsylvania Vector Core | ||
Recombinant DNA reagent | Ade-mtaequorin | Gift from Rosario Rizzuto lab, Tosatto et al., 2016 | ||
Antibody | anti-MPC1 (rabbit) | Sigma Aldrich(HPA045119) | RRID:AB_10960421 | 1/2000 (WB)1/1000 (IF)1/250(IHC) |
Antibody | anti-MPC2 (Mouse) | Millipore (MABS1914) | MABS1914 | 1/500 (WB) |
Antibody | anti-bIII-tubulin (Mouse) | Biolegend(801201) | RRID:AB_2313773 | 1/5000 (WB)1/1000 (IF) |
Antibody | anti-MCU (Rabbit) | Sigma Aldrich (HPA 016480) | RRID:AB_2071893 | 1/5000 (WB) |
Antibody | anti-HSP70 (Mouse) | Millipore (MABS1955) | MABS1955 | 1/1000 (WB) |
Antibody | Anti-TOMM20 (Rabbit) | Abcam (Ab186735) | RRID:AB_2889972 | 1/2000 (IF) |
Antibody | anti-synaptophysin (Mouse) | Abcam (ab8049) | RRID:AB_2198854 | 1/2000 (WB) |
Antibody | anti-Tyrosine Hydroxylase (Rabbit) | Millipore (AB152) | RRID:AB_390204 | 1/2000 (WB) |
Antibody | anti-CamKIIa (Goat) | Abcam (ab87597) | RRID:AB_2040677 | 1/5000 (WB) |
Antibody | anti-GFAP (Mouse) | Sigma Aldrich (G3893) | RRID:AB_477010 | 1/5000 (WB) |
Antibody | anti-VDAC (Goat) | Santa Cruz (sc-8829) | RRID:AB_2214801 | 1/500 (WB) |
Antibody | anti-bActin (Mouse) | Sigma Aldrich (a3854) | RRID:AB_262011 | 1/50000 (WB) |
Antibody | goat anti-mouse Alexa Fluor488 | Life technologies (A32723) | RRID:AB_2633275 | 1/2000 |
Antibody | goat anti-rabbit Alexa Fluor594 | Life technologies (A11037) | RRID:AB_2534095 | 1/2000 |
Antibody | anti-IgG-Mouse-HRP | Dako (P0447) | RRID:AB_2617137 | 1/10000 |
Antibody | anti-IgG-Rabbit-HRP | Dako (P0217) | RRID:AB_2728719 | 1/10000 |
Antibody | anti-IgG-Goat-HRP | Santa Cruz (sc-2304) | RRID:AB_641158 | 1/10000 |
Commercial assay or kit | CellTiter Glo | Promega | G9241 | |
Commercial assay or kit | DeadEnd Colorimetric TUNEL System | Promega | G7130 | |
Commercial assay or kit | GlucoMen Lx Plus kit | Menarini diagnostics | ||
Other | Ketogenic diet | Provimi Kliba AG | XL75:XP10 | |
Chemical compound, drug | FuraFF | Thermo Fisher Scientific | F14181 | |
Chemical compound, drug | Fura2-AM | Thermo Fisher Scientific | F1221 | |
Chemical compound, drug | Fluo4-AM | Thermo Fisher Scientific | F14201 | |
Chemical compound, drug | 2-NDBG (2-(N-(7-Nitrobenz-2-oxa-1,3-diazol-4-yl)Amino)–2-Deoxyglucose) | Thermo Fisher Scientific | N13195 | |
Chemical compound, drug | Pentylenetetrazol (PTZ) | Sigma Aldrich | P6500 | |
Chemical compound, drug | Kainic Acid | Sigma Aldrich | K0250 | |
Chemical compound, drug | Tamoxifen | Sigma Aldrich | 85,256 | |
Software, algorithm | Prism7 | Graphpad version 7.0 a, April 2, 2016. | RRID:SCR_002798 | |
Other | Digidata1550A digitizer | Molecular Devices | ||
Other | Digital Lynx SX | Neuralynx, USA | ||
Other | Seahorse XF 24 extracellular flux analyzer | Seahorse Biosciences | ||
Other | Cytation 3TM | Biotek Instrument Inc | ||
Other | IX71 Olympus microscope | Olympus | ||
Other | Confocal LSM780 microscope | Zeiss |
Additional files
-
Source data 1
Uncropped western blot corresponding to Figure 1—figure supplement 1A, Figure 1—figure supplement 1B, Figure 2C, Figure 3—figure supplement 1E, Figure 6—figure supplement 2A.
- https://cdn.elifesciences.org/articles/72595/elife-72595-data1-v2.pptx
-
Transparent reporting form
- https://cdn.elifesciences.org/articles/72595/elife-72595-transrepform1-v2.docx