The role of V3 neurons in speed-dependent interlimb coordination during locomotion in mice
Figures
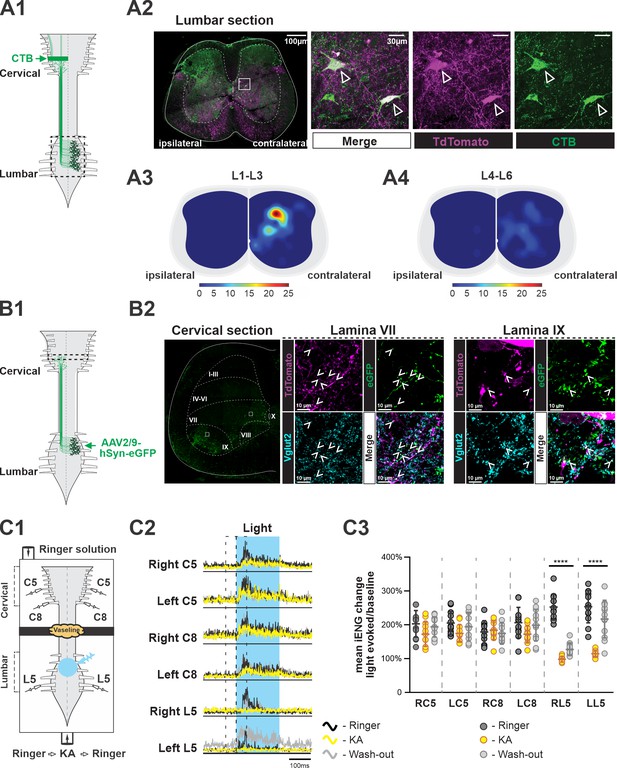
Ascending V3 long propriospinal neurons identified in the lumbar spinal cord.
(A1) Illustration of the experimental strategy to identify lumbar V3 ascending long propriospinal interactions (aLPNs). Cholera-toxin B (CTB; green) injected into the cervical region is picked up at axon terminals and retrogradely transported to cell bodies located in the lumbar region. (A2) Representative image of cross section of the lumbar spinal cord of Sim1tdTom mouse (far left panel). The ‘ipsilateral’ and ‘contralateral’ sides are relative to the injection side in the cervical region. Immunohistochemical staining with different antibodies to illustrate the neurons expressing TdTom (magenta) and CTB (green) (right panels). (A3, A4) Color-coded heat maps of the distribution pattern of V3 aLPNs in the rostral (A3) and caudal (A4) lumbar segments. Scale bars of cell numbers for heat maps are shown below. (B1) Schematic of the experimental strategy to identify lumbar V3 aLPN synapses onto the cervical locomotor region, AAV2/9-hSyn-eGFP is injected into the lumbar region. (B2) Left: a representative image of a cervical hemisection injected with AAV2/9-hSyn-eGFP in the lumbar region. The dashed lines indicate approximate Rexed’s laminae, and white squares indicate the approximate positions of the enlarged images. Middle (lamina VII) and right (lamina IX): representative images of the Vglut2-positive terminals (blue) of lumbar V3 aLPNs (magenta) expressing GFP (green) in cervical locomotor regions. Three random regions (~480 µm × 370 µm) in lamina IX and six regions (~370 µm × 370 µm) in lamina VII region from two cords were sampled. The Vglut2/GFP double-positive puncta (mean of 481.7 ± 47.4 in lamina IX; 143.2 ± 33.4 in lamina VII) and Vglut2/GFP/tdTom triple-positive puncta (12.7 ± 1.7 in lamina IX; 39.5 ± 9.3 in lamina VII) were quantified. (C1) Illustration of an isolated neonatal spinal cord placed in a split bath recording chamber. The suction electrodes for the electroneurogram (ENG) recordings were placed at ventral roots in the lumbar and cervical segments. The chamber is partitioned into two sides with a Vaseline wall (represented by yellow cloud in the figure). The photoactivation (blue light), indicated by the blue transparent circle, is on the ventral side of rostral lumbar, L1–L3, segments. (C2) Averaged traces of rectified ENG recordings at ventral roots of both sides of cervical (C5, C8) and L5 lumbar spinal segments of a P2 Sim1Ai32 mouse, before (Ringer, black), during (kynurenic acid [KA], yellow), and after washout (Washout, gray) of KA application. The time period of photostimulation is indicated by the blue shadowed area. (C3) The changes in integral ENG activity during light stimulation (n = 12) at the period before (dark gray circles), during (yellow circles), and after (light gray circles) KA application. Each circle represents the value of estimated parameter for one trace. Statistics for C3 can be found in Supplementary file 1.
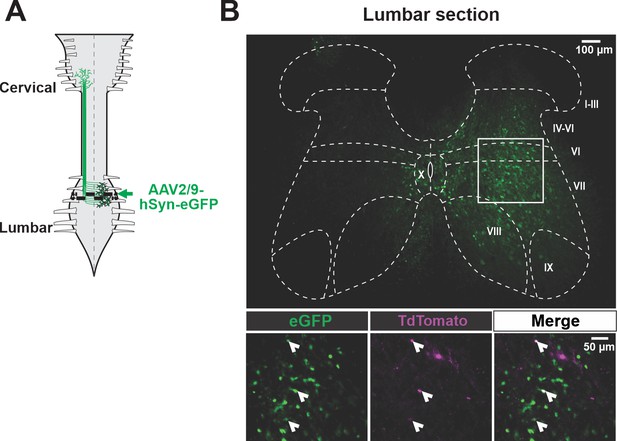
V3 neurons are infected by AAV injection in the lumbar spinal cord.
(A) Schematic of the experimental strategy to identify lumbar V3 ascending long propriospinal interactions (aLPNs) synapses onto the cervical locomotor region, laminae VII and IX. AAV2/9-hSyn-eGFP was injected into the rostral lumbar region. (B) Representative image of a cross section of the lumbar spinal cord expressing GFP (green, top panel). Enlarged representative images (bottom) show V3 neurons (magenta) that express eGFP (green) from the lumbar AAV injection.
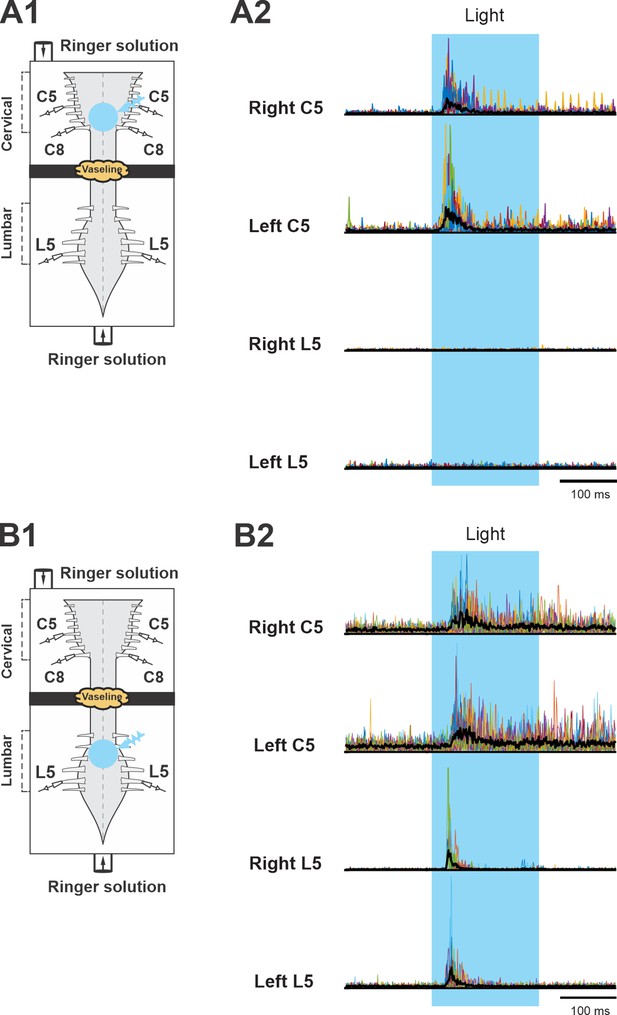
Lumbar and cervical motor activity in response to photoactivation of V3 neurons in the lumbar and cervical region.
(A1, B1) Schematics of the experimental strategy. Isolated neonatal spinal cord with suction electrodes attached to ventral roots is placed in a split bath recording chamber. The chamber is partitioned into two sides with a Vaseline wall (represented by yellow cloud in the figure). The electroneurogram (ENG) activities of left and right cervical or lumbar ventral roots were recorded. The photoactivation (blue light) indicated by the blue circle is on the ventral side of rostral cervical, C5–C7 (A1), or of rostral lumbar, L1–L3 (B1), segments. (A2, B2) Averaged traces of rectified ENG recordings at ventral roots are indicated by thick, black lines. The individual raw recording traces from each light stimulation are indicated by the thin lines in different colors.
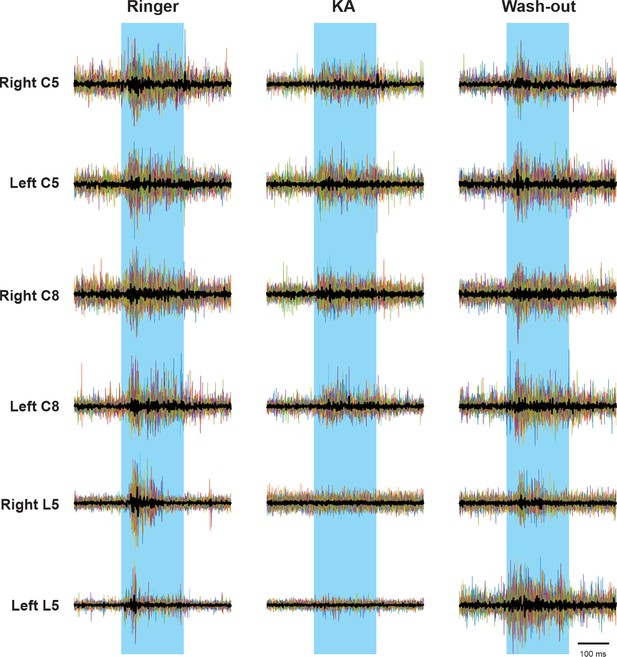
Electroneurogram (ENG) recordings of cervical and lumbar roots responding to the photoactivation of lumbar V3 neurons.
The individual raw traces from each light stimulation are indicated by the thin lines in different colors. Averaged traces of rectified ENG recordings at ventral roots are indicated by thick, black lines.
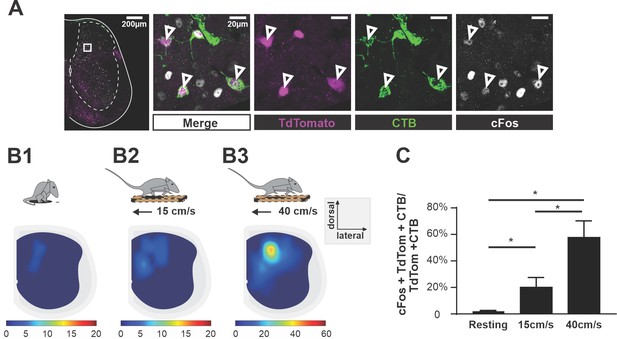
Ascending V3 long propriospinal neurons are recruited during locomotion.
(A) Representative image of TdTom+ V3 neurons (magenta), cholera-toxin B (CTB) (green), and c-Fos (white) of a half cross section of the spinal cord from a Sim1Cre/+; Rosa26floxstopTdTom (Sim1tdTom) mouse. Enlarged images showing triple-positive cells. Scale bars: 200 μM and 20 μM. (B1–B3) Color-coded heat maps showing the distribution of c-Fos, CTB, and TdTom triple-positive V3 neurons in a cross section of lumbar spinal cord during resting (B1), 15 cm/s (B2), and 40 cm/s (B3) treadmill locomotion (nresting = 3, n15cm/s=3, n40cm/s = 3). (C) Histogram of the corresponding percentage of triple-positive cells in TdTom/CTB double-positive population in L1–L3 segments. *0.01<p<0.05. Statistics for (C) can be found in Supplementary file 1.
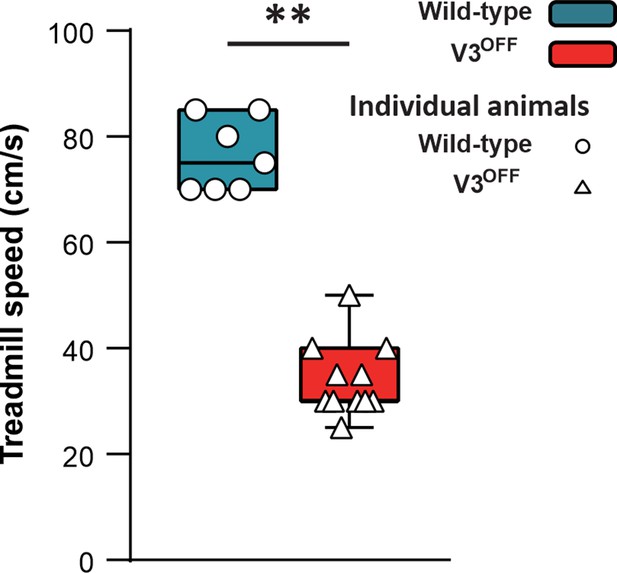
Maximum speeds of wildtype (WT) and V3OFF mice on the treadmill.
The box-and-whisker plots showing the highest speed of the individual WT mice (n = 7) and V3OFF mice (n = 11) on the treadmill. **p<0.01. Statistics can be found in Supplementary file 1.
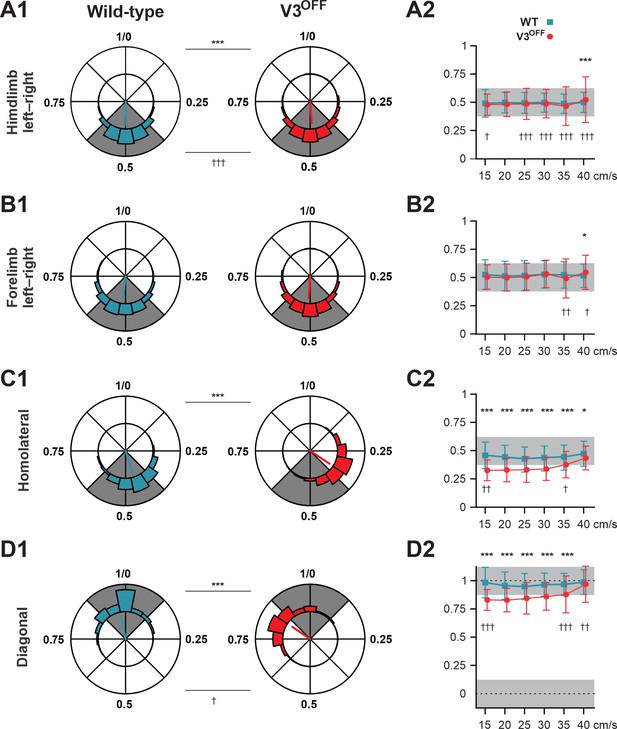
Interlimb coordination in wildtype (WT) and V3OFF mice at different speeds.
(A1–D1) Circular plots of hindlimb (A1) and forelimb (B1) left–right phase differences, homolateral phase differences (C1), and diagonal phase differences (D1) in WT (blue) and V3OFF (red) mice. Except for the forelimb left–right phase differences, the left hindlimbs are used as the reference limb. Each vector, blue line (WT) and red line (V3OFF), in the circular plot, indicates the mean value (direction) and robustness (radial line/length) of the phase difference. The circle is evenly separated into eight fractions. The circular histograms represent the distribution of phase differences of all steps at all tested speeds (WT, n = 1292; V3OFF, n = 1478). (A2–D2) Plots of mean values of coupling phases at individual speeds of V3OFF (red) and WT (blue) mice. *p<0.01; **p<0.001; ***p<0.0001 for comparisons of mean phase differences; †p<0.01; ††p<0.001; †††p<0.0001 for comparisons of the variability (concentration parameter κ) of the phase differences. Statistics for (A1–D1) and (A2–D2) can be found in Supplementary file 1.
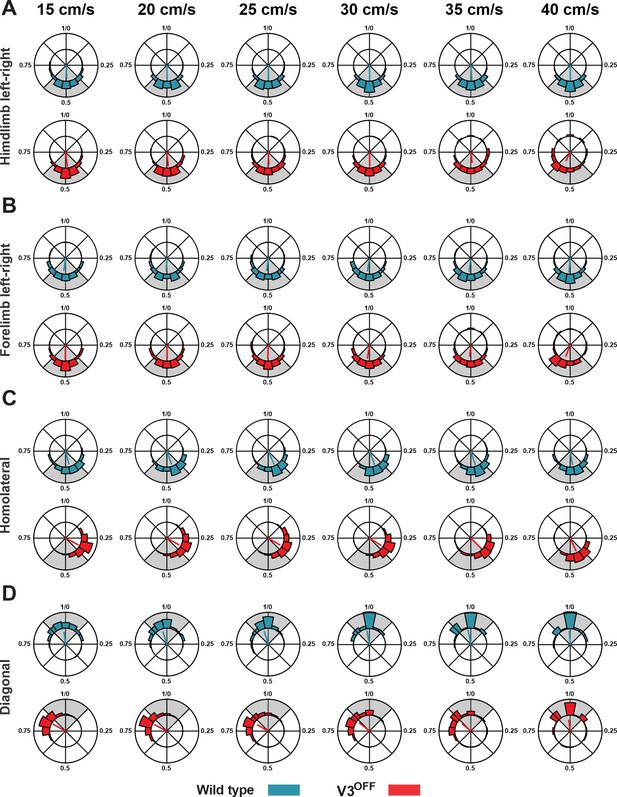
Limb couplings of wildtype (WT) and V3OFF mice at different speeds.
Circular plots of hindlimb (A) and forelimb (B) left–right phase differences, homolateral phase differences (C), and diagonal phase differences (D) in WT (blue) and V3OFF (red) mice at individual treadmill speeds. Except for the forelimb left–right phase differences, the left hindlimbs are used as the reference limb. Each vector, blue line (WT) and red line (V3OFF), in the circular plot, indicates the mean value (direction) and robustness (radial line/length) of the phase differences. The circle is evenly separated into eight fractions. The circular histograms represent the distribution of phase differences of all steps at all tested speeds.
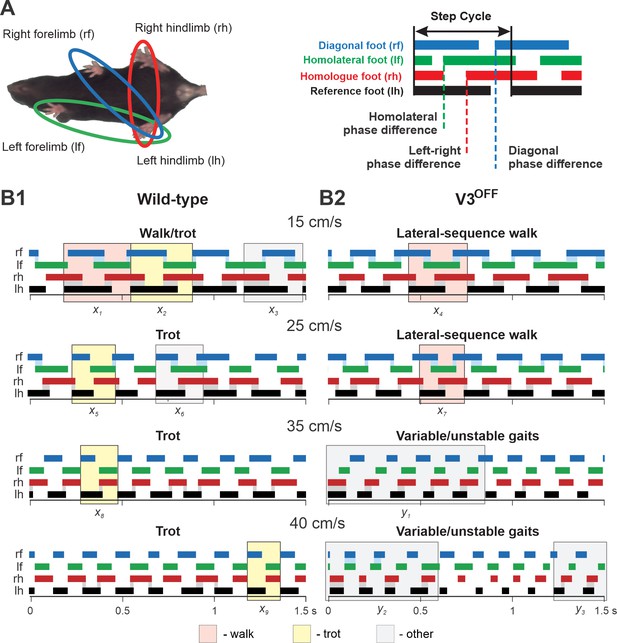
Step patterns of wildtype (WT) and V3OFF mice at different speeds.
(A) Illustration of limb couplings (left). The footprint diagrams of individual limb are represented by color-coded bar graphs (right): the stance phase of each step is shown with solid bar and swing phase is the interval between two bars. The step cycle is measured from the duration between the onset of contacts of two consecutive steps of the same foot. Phase difference between the limbs was calculated as the interval between the start of stance of a specific limb and the reference limb divided by the period or step-cycle duration of the reference limb. (B1, B2) Representative stance phases of 1.5 s episodes of WT (B1) and V3OFF (B2) mice at low (15 cm/s and 25 cm/s) and medium (35 cm/s and 40 cm/s) treadmill speeds. x1–9, exemplary steps; y1–3, exemplary episodes referred to from the text. The shading color indicates the gait (pink, lateral-sequence walk; yellow, trot; light gray, other). Blue and gray shadows highlight periods of overlap between stance phases of the homologue limbs. The black bars show the stance phase of the reference foot (left hindlimb).
Wild-type and V3OFF mice locomotion at 15 cm/s.
Wild-type and V3OFF mice locomotion at 25 cm/s.
Wild-type and V3OFF mice locomotion at 35 cm/s.
Wild-type and V3OFF mice locomotion at 40 cm/s.
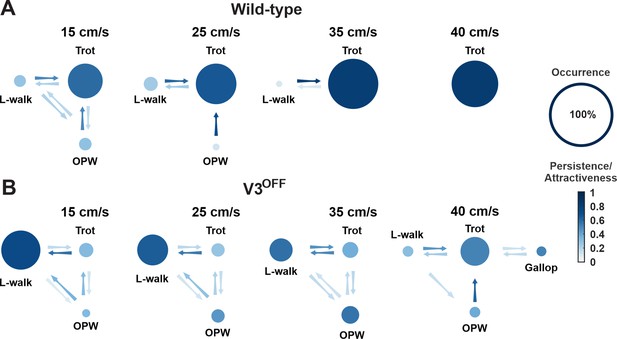
Gait transition diagrams of wildtype (WT) (A) and V3OFF (B) mice at different speeds.
The size of circle indicates the relative occurrence of the indicated gait. The full size of the circle meaning 100% occurrence is shown on the far right. The intensity gradient of the color of the circles indicates the persistence of the corresponding gait. The intensity gradient of the color of the arrows indicates the likelihood of a transition.
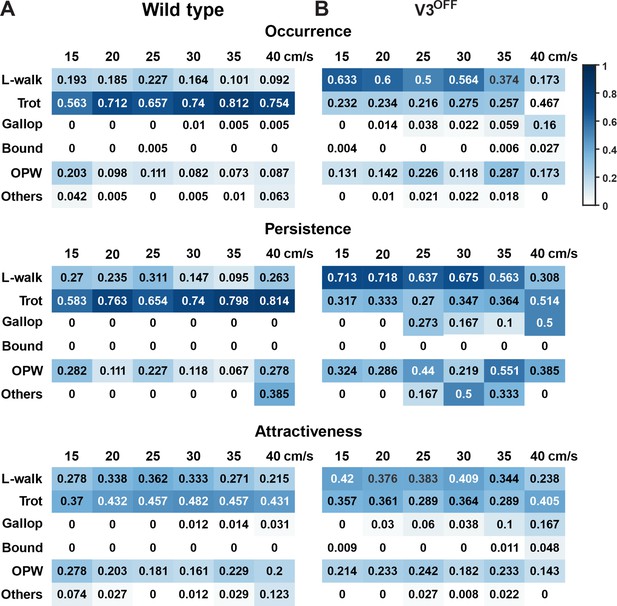
Gait preference of wildtype (WT) and V3OFF mice at individual speeds.
Color-coded matrices of occurrence, persistence, and attractiveness values of each type of gait (row) at each tested speed (column). The matrices in (A) are from WT and (B) are from V3OFF mice. The number in each cell shows the value of the parameter of corresponding gait and speed. L-walk, lateral-sequence walk; OPW, out-of-phase walk.
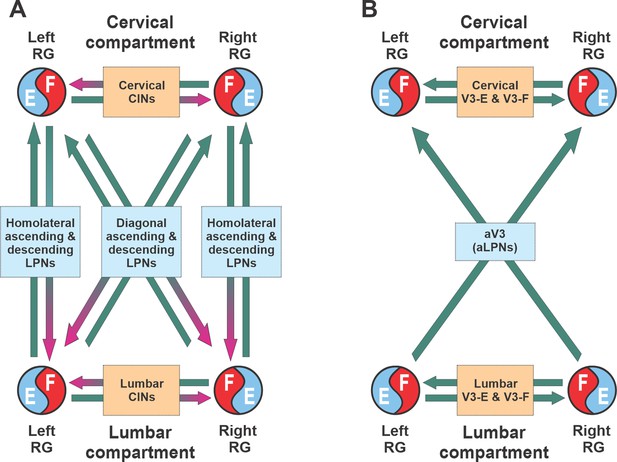
Conceptual model schematic.
(A) The model incorporates two bilateral compartments (cervical and lumbar). Each compartment includes the left and right rhythm generators (RGs) each controlling a single limb and interacting via commissural interneurons (CINs). The cervical and lumbar compartments interact via homolaterally and diagonally projecting, descending and ascending long propriospinal neurons (LPNs). (B) Interactions between four RGs by different populations of V3 interneurons.
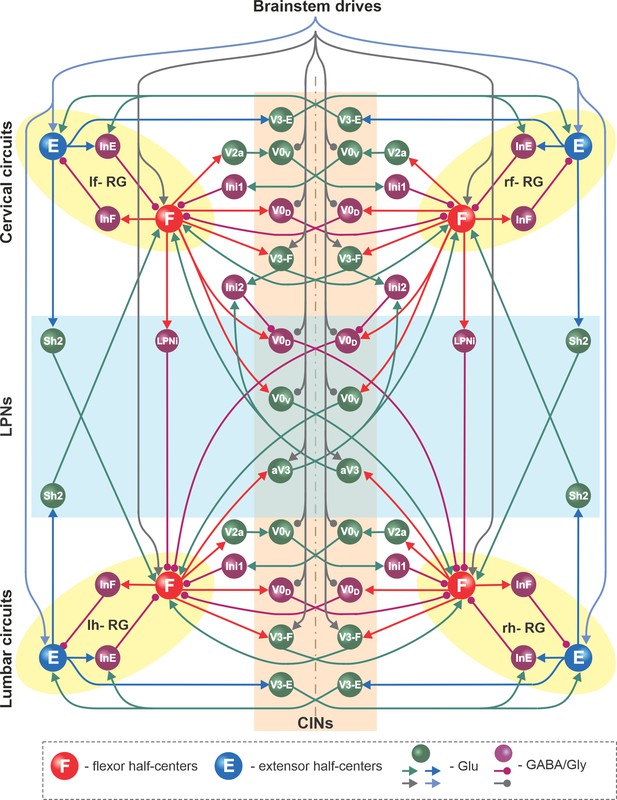
Full model schematic.
Spheres represent neural populations and lines represent synaptic connections. Excitatory (Glu) and inhibitory (GABA/Gly) connections are marked by arrowheads and circles, respectively. RG, rhythm generator; CINs, commissural interneurons; LPNs, long propriospinal neurons.
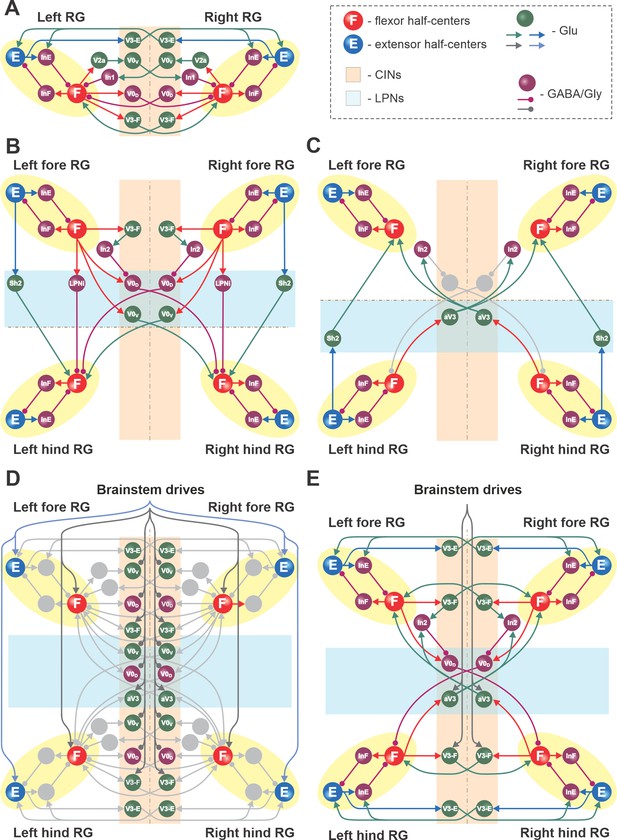
Connections within the spinal cord in the model.
(A) Connections between the left and right rhythm generators (RGs) within each compartment (see text for details). (B) Cervical-to-lumbar connections via descending long propriospinal neurons (dLPNs). (C) Lumbar-to-cervical connections via ascending long propriospinal neurons (aLPNs). (D) Brainstem drive to the extensor and flexor half-centers (E and F), commissural interneurons (CINs), and long propriospinal neurons (LPNs). (E) Local and long propriospinal connections in the model mediated by V3 interneurons. Neural populations are shown by spheres. Excitatory and inhibitory connections between populations are shown by arrowheads and circles, respectively.
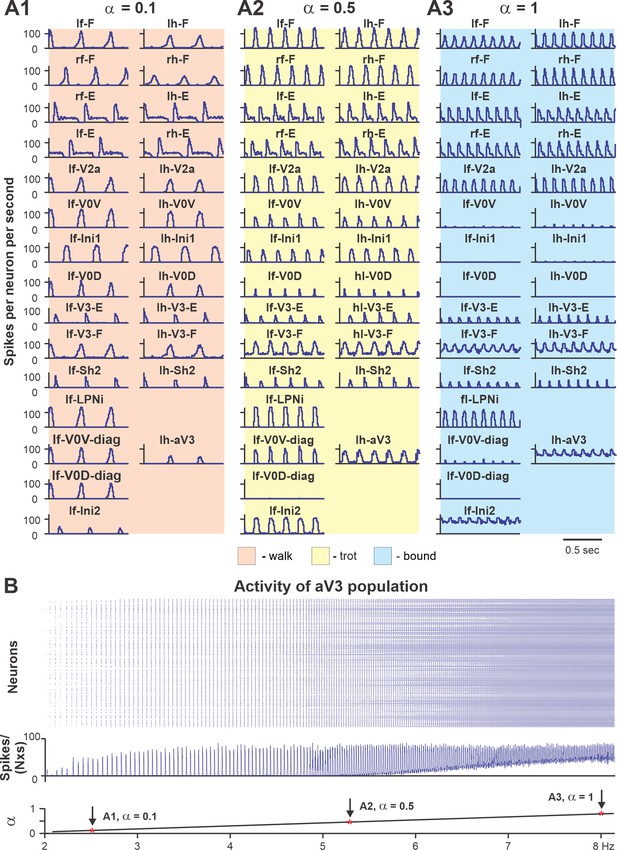
Performance of the intact model for three values of brainstem drive.
(A1–A3) Model performance for three sequentially increased values of parameter α ( = {0.1; 0.5; 1}) that controls brainstem drive to the network. In all panels, activities of flexor and extensor (F and E) half-centers and only left cervical (fore, f-) and lumbar (hind, h-) interneuron populations are shown. Integrated activities of all populations are shown as average histograms of neuron activity [spikes/(N × s), where N is a number of neurons in population; bin = 10 ms]. (B) Raster plot (upper panel) of activity of the left lumbar aV3 population when the brainstem drive (lower panel) was increased from 0.1 to 1. Note increased recruitment of aV3 neurons with the increased drive. rf, right fore; lf, left fore; rh, right hind; lh, left hind.
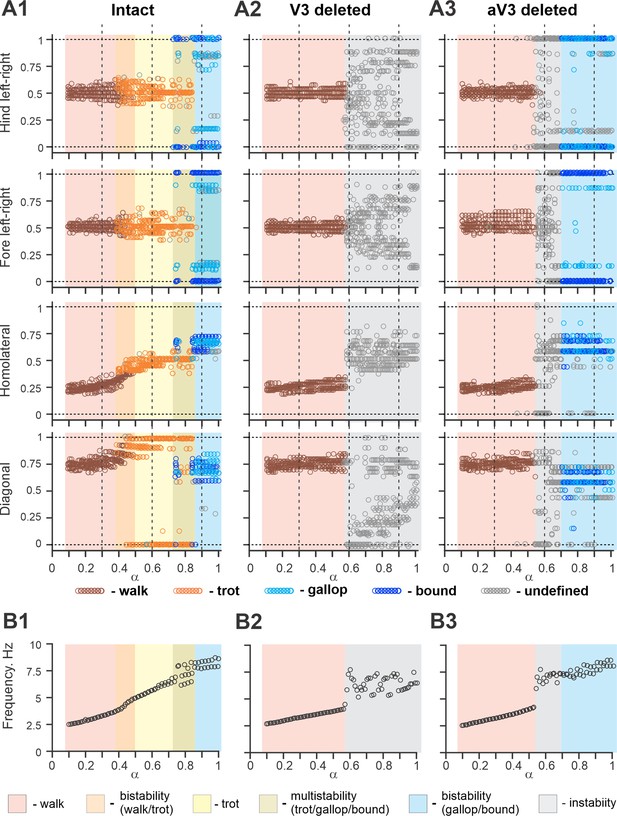
Bifurcation diagrams of the intact model, and after removal of all V3 or only propriospinal aV3 neurons.
Bifurcation diagrams reflecting changes in the normalized phase differences between oscillations in the left and right hind (lumbar) rhythm generators (RGs), left and right fore (cervical) RGs, homolateral, and diagonal RGs with a progressive increase in brainstem drive () from 0.1 to 1 with a step of 0.01 (see for details ‘Modeling methods’ and Danner et al., 2017). (A1) Intact model. (A2) The result of removal of all V3 neurons (V3OFF simulation). (A3) Only the diagonal aV3 are removed. Parameter α that represents the brainstem drive was used as bifurcation parameters. Each diagram shows phase differences calculated for five locomotor periods when α was fixed. The vertical dashed lines correspond to three values of α = {0.3; 0.6; 0.9} for which complimentary circular plot diagrams are built showing phase differences between activities of the left hind E population (lh-E) and other relative populations for the intact model and after deletion of all V3 or aV3 neurons. (B1–B3) Average oscillation frequency corresponding to simulations shown in (A1–A3).
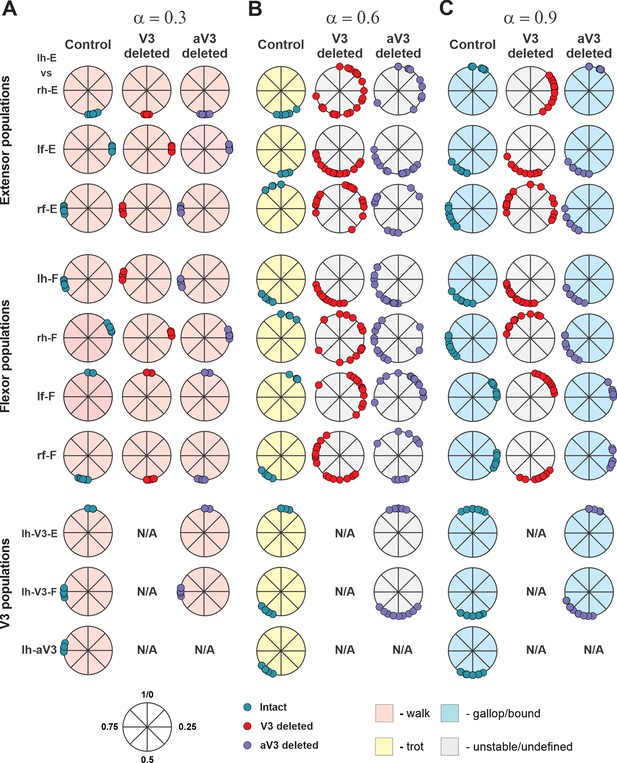
Phase differences for intact, V3- and aV3-deleted model for three different values of α.
Phase differences between activities of the left hind E population and other rhythm generator (RG) (rh-E, lf-E, rf-E, lh-F, lf-F, rh-F, and rf-F) and left V3 (lh-V3-E, lh-V3-F, and lh-aV3) populations for the intact model and after deletion of all V3 or aV3 neurons are shown as circular plot diagrams for all steps for three values of α = {0.3; 0.6; 0.9} for 5 s of simulation time. rf, right fore; lf, left fore; rh, right hind; lh, left hind.
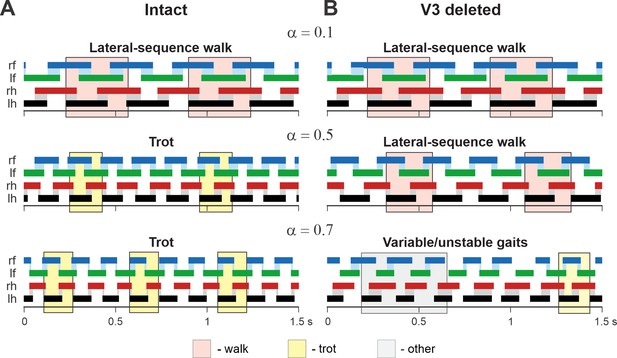
Examples of the step diagrams for the intact model (A) and after all V3 neurons were deleted (B).
For each rhythm generator (RG), the lengths of the corresponding extensor phases are shown for 1.5 s of the simulation time for three values of α = {0.1; 0.5; 0.7}. At α = 0.1, both intact and V3-deleted models demonstrate stable lateral-sequence walking gait. At α = 0.5, the intact model exhibits a trot, and the V3-deleted model demonstrates a lateral-sequence walk. At α = 0.7, the intact model has a stable trot while the V3-deleted model demonstrates unstable behavior. The solid color bars show the extensor phases of all four RGs: rf, right fore; lf, left fore; rh, right hind; lh, left hind. The black bars show the reference RG (lh). Blue and gray shadows highlight periods of overlap between extensor phases of the homologue RGs. The shading color indicates the gait (pink, lateral-sequence walk; yellow, trot; light gray, other).
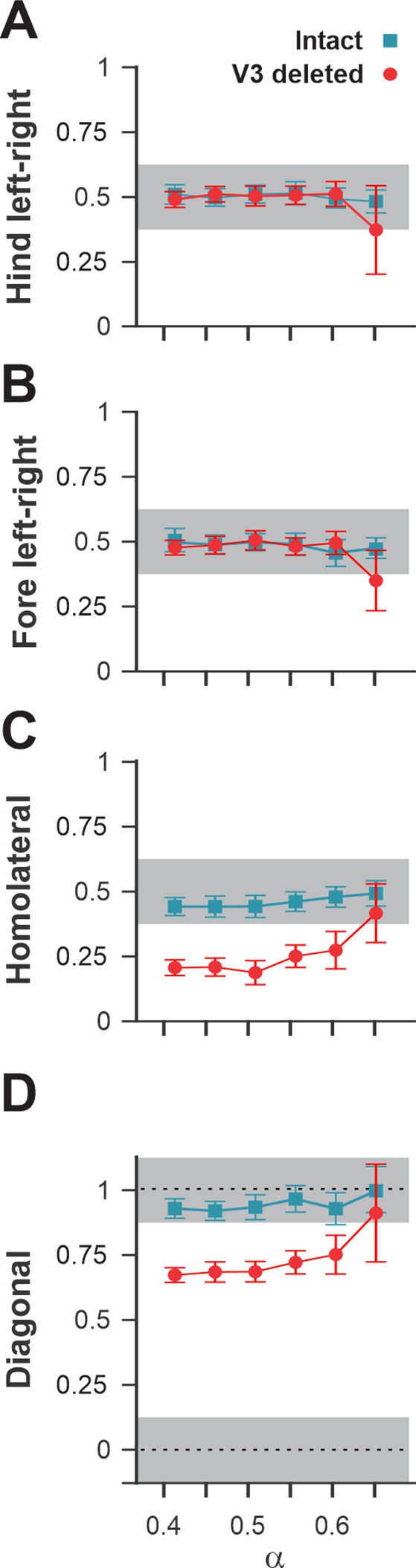
Interlimb coordination in the intact model and after removal of all V3 neurons.
Interlimb coordination in the intact (blue) and V3-deleted (red) models for six values of the brainstem drive is shown for left–right hind (A), left–right fore (B), homolateral (C), and diagonal (D) normalized phase differences. For each value of , phase differences were averaged over 10–20 consecutive oscillation periods. In (A), (C), and (D), the extensor phase of the left hind rhythm generator (RG) is chosen as the reference. Note the homolateral and diagonal phase difference shift toward walking in V3-deleted model compared to trotting gate in the intact case.
Tables
Brainstem drive parameters.
Target population | ||
---|---|---|
Excitatory drives | ||
RG-E | 0.0 | 0.5 |
RG-F (f) | 0.15 | 0 |
RG-F (h) | 0.3 | 0 |
V3-F | 1 | 0 |
aV3 | 1 | 0 |
Inhibitory drives | ||
V0V | -8 | 0 |
V0D | –10 | 0 |
Diagonal V0V | -3 | 0 |
Diagonal V0D | -3 | 0 |
-
f, forelimb; h, hindlimb; RG, rhythm generator.
Connection weights.
Source | Target () |
---|---|
Within cervical and lumbar circuits | |
i-F | i-F (0.0125, P = 0.1); i-InF (0.3, P = 0.1), i-V0D (0.3, P = 0.1), i-V2a (0.3, P = 0.1), i-V3-F (0.3, P = 0.1) |
i-E | i-E (0.0125, P = 0.1); i-InE (0.2, P = 0.1), i-V3-E (0.2, P = 0.05), i-Sh2 (0.1, P = 0.1) |
i-InF | i-E (–0.6, P = 0.1) |
i-InE | i-F (–0.07, P = 0.1) |
i-V2a | i-V0V (0.4, P = 0.1) |
i- V0V | c-Ini1 (0.4, P = 0.1) |
i-Ini1 | i-F (–2, P = 0.1) |
i-V0D | c-F (–1, P = 0.1) |
i-V3-E | c-E (0.01, P = 0.05), c-InE (0.2, P = 0.05) |
i-V3-F | c-F (0.03, P = 0.1), i-Ini2 (0.05, P = 0.1) |
Within cervical circuits | |
i-F | i-LPNi (0.4, P = 0.1), diagonal i-V0D (0.25, P = 0.1), diagonal i-V0V (0.25, P = 0.1) |
Within lumbar circuits | |
i-F | i-aV3 (0.1, P = 0.1) |
Between cervical and lumbar circuits | |
i-Sh2 (f) | ih-F (0.02, P = 0.1) |
i-Sh2 (h) | if-F (0.05, P = 0.1) |
i-LPNi (f) | ih-F (–0.2, P = 0.1) |
diagonal i-V0D (f) | ch-F (–1.2, P = 0.1) |
diagonal if-V0V (f) | ch-F (0.1, P = 0.1) |
i-aV3 | cf-F (0.12, P = 0.1), c-Ini2 (0.5, P = 0.1) |
-
i, ipsilateral; c, contralateral; f, forelimb; h, hindlimb.
Additional files
-
Supplementary file 1
Statistical results.
- https://cdn.elifesciences.org/articles/73424/elife-73424-supp1-v1.docx
-
Transparent reporting form
- https://cdn.elifesciences.org/articles/73424/elife-73424-transrepform1-v1.docx