Therapeutic deep brain stimulation disrupts movement-related subthalamic nucleus activity in parkinsonian mice
Figures
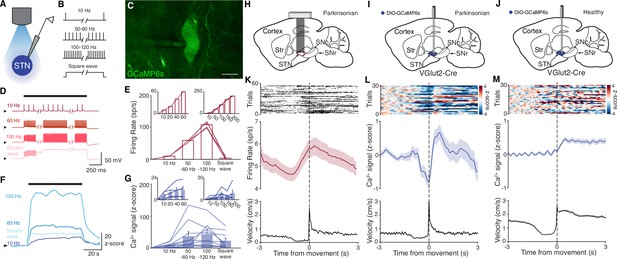
Subthalamic nucleus (STN) GCaMP signals correlate with spiking measured by electrophysiology.
(A–G) Combined ex vivo electrophysiological and calcium imaging recordings in STN neurons. Neurons were patched in the whole-cell current-clamp configuration. (A) Combined whole-cell/calcium imaging recording configuration. (B) Brief intracellular current pulses were provided at different frequencies up to 200 Hz and with prolonged depolarization (‘square wave’). (C) Image of GCaMP-expressing STN neuron (scale bar = 10 μm). (D) Representative STN neuron responses to intracellular current pulses at different frequencies and to prolonged depolarization (‘square wave’). (E) Average firing rate of STN neurons in response to stimulation at a range of frequencies and prolonged depolarization (n=10 cells, N=3 mice for main; n=5 cells, N=1 mouse for each inset). (F) Representative trace of z-scored STN GCaMP signal in response to current-clamp stimulation. (G) Average z-scored STN GCaMP signal in response to intracellular current pulses at a range of frequencies and prolonged depolarization (n=10 cells, N=3 mice for main; n=5 cells, N=1 mouse for each inset). Arrowhead in current-clamp traces and GCaMP traces corresponds to –75 mV and 0 z-score, respectively. Bar plots show mean ± SEM. (H–M) In vivo electrophysiological and GCaMP fiber photometry recordings in STN neurons from freely moving mice, aligned to movement starts. (H) Sagittal schematic showing multielectrode array implant in the STN or parkinsonian mice for single-unit electrophysiology. (I, J) Sagittal schematic showing STN GCaMP and fiber implant for photometry in parkinsonian (I) and healthy (J) mice. (K) Representative STN single-unit firing (top), average firing rate (middle), and average velocity (bottom) aligned to movement starts (n=17 cells, N=3 mice). (L, M) Representative STN fiber photometry signal (top), average fiber photometry signal (middle), and average velocity (bottom) aligned to movement starts in parkinsonian (L, N=8) and healthy (M, N=5) mice. Average firing rate, photometry, and velocity traces show mean ± SEM.
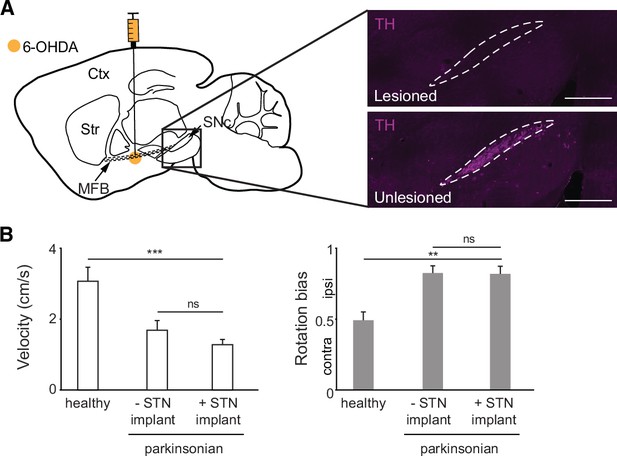
Hemiparkinsonian mice show decreased movement velocity and ipsilesional rotation bias.
(A) Left: Sagittal schematic showing unilateral injection of 6-hydroxydopamine (6-OHDA) to deplete ipsilateral dopamine neurons. Right: Postmortem sagittal section showing depletion of TH (purple) in lesioned hemisphere (dotted lines indicate borders of substantia nigra, pars compacta; scale bar = 500 μm). (B) Comparison of average velocity (left) and rotation bias (right) in healthy mice (N=9 mice), hemiparkinsonian mice without STN implants (N=10 mice), and hemiparkinsonian mice with STN implants (N=9 mice). Statistical significance was determined using a Wilcoxon rank-sum test; **p<.01, ***p < 0.001 (see Supplementary file 1, table 1 for detailed statistics). Bar plots show mean ± SEM.
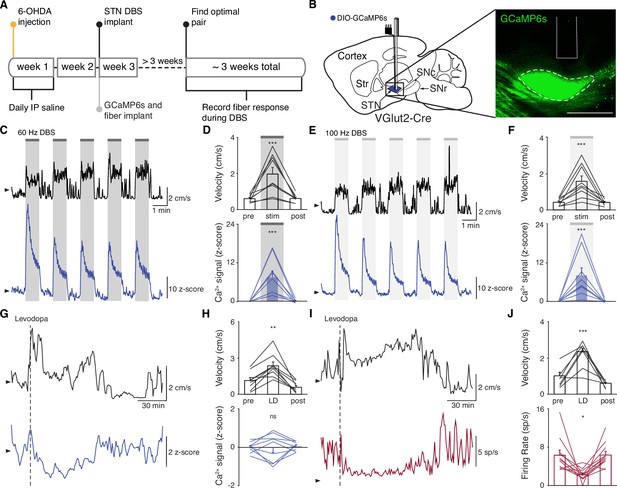
Subthalamic nucleus deep brain stimulation (STN DBS) consistently increases STN activity.
(A) Experimental timeline. (B) Left: Sagittal schematic showing STN DBS and GCaMP fiber photometry. Right: Postmortem sagittal section showing GCaMP expression and estimated fiber placement in the STN (inset, scale = 500 μm). (C) Representative single-session velocity (black) and STN GCaMP signal (blue) in response to 60 Hz STN DBS. (D) Average velocity (top) and STN GCaMP signal (bottom) before, during, and after 60 Hz STN DBS (N=9 mice). (E) Representative single-session velocity (black) and STN GCaMP signal (blue) in response to 100 Hz STN DBS. (F) Average velocity (top) and STN GCaMP signal (bottom) before, during, and after 100 Hz STN DBS (N=9 mice). (G) Representative single-session velocity (black) and STN GCaMP signal (blue) before and after levodopa injection (dotted line). (H) Average velocity (top) and STN GCaMP signal (bottom) before, during, and after levodopa treatment (N=9 mice). (I) Representative single-session velocity (black) and STN single-unit activity (red) before and after levodopa injection (dotted line). (J) Average velocity (top) and STN single-unit activity (bottom) before, during, and after levodopa treatment (n=11 cells, N=3 mice). Statistical significance was determined using a one-way repeated measures ANOVA with a Tukey HSD post hoc analysis applied to correct for multiple comparisons; *p < 0.05, **p < 0.01, ***p < 0.001 (only comparison between pre and stim/LD shown, see Supplementary file 1, table 1 for detailed statistics). Arrowhead in velocity, GCaMP, and single-unit electrophysiology traces corresponds to 1 cm/s, 0 z-score, and 0 spike/s, respectively. Bar plots show mean ± SEM.
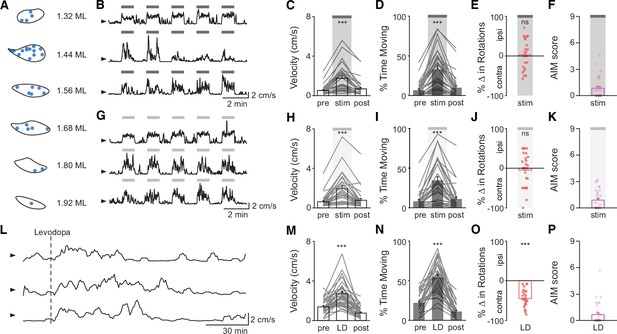
STN DBS and levodopa alleviate some motor signs in parkinsonian mice.
(A) Targeting of subthalamic nucleus deep brain stimulation (STN DBS) electrodes to the STN in 32 mice. (B) Representative single-session velocities in response to 60 Hz STN DBS in three mice. (C) Binned average velocity, (D) percent time moving, (E) change in rotational bias, and (F) dyskinesia in response to 60 Hz STN DBS (N=32 mice). (G) Representative single-session velocities in response to 100 Hz STN DBS in three mice. (H) Binned average velocity, (I) percent time moving, (J) change in rotational bias, and (K) dyskinesia in response to 100 Hz STN DBS (N=31 mice). (L) Representative single-session velocities before and after levodopa injection (dotted line) in three mice. (M) Binned average velocity, (N) percent time moving, (O) change in rotational bias, and (P) dyskinesia in response to levodopa injection (N=30 mice). Dyskinesia was quantified with as the abnormal involuntary movement (AIM) score. Statistical significance was determined using a Wilcoxon signed-rank test (E,I,O); a one-way repeated measures ANOVA with a Tukey HSD post hoc analysis applied to correct for multiple comparisons (M–N); or a Friedman test with a Tukey HSD post hoc analysis applied to correct for multiple comparisons (C,D,H,I); ***p < 0.001 (for ANOVA/Friedman, only comparison between pre and stim/LD shown, see Supplementary file 1, table 1 for detailed statistics). Arrowhead in velocity traces corresponds to 1 cm/s. Bar plots show mean ± SEM.
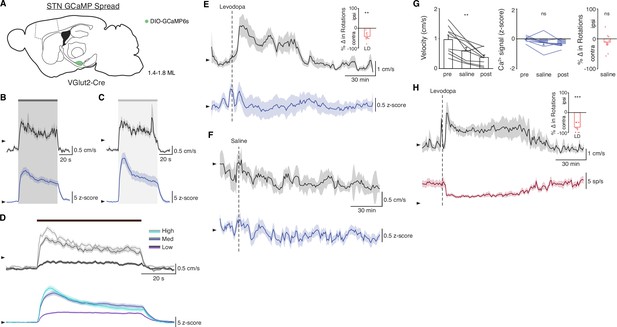
STN DBS increases STN activity in vivo.
(A) Sagittal schematic showing estimated extent of viral GCaMP6s spread in the subthalamic nucleus (STN) of VGlut2-Cre mice (N=9 mice). (B–C) Average movement velocity over time (black) and STN GCaMP signal (blue) following 60 Hz STN deep brain stimulation (DBS) (B, N=9 mice) or 100 Hz STN DBS (C, N=9 mice). (D) Average movement velocity (top) and STN GCaMP signal (bottom) during DBS sessions grouped by stimulation frequency: Low = 5–40 Hz, Med = 60–100 Hz, High = 120–180 Hz stimulation. (E) Average movement velocity over time (black) and STN GCaMP signal (blue) following administration of levodopa (N=9 mice) and average change in rotation bias during levodopa (inset). (F) Average movement velocity over time (black) and STN GCaMP signal (blue) following administration of saline (N=9 mice). (G) Average velocity (left), STN GCaMP signal (middle), and change in rotation bias before, during, and after saline injection (N=9 mice). (H) Average velocity over time (black) and STN single-unit firing rate (red) following administration of levodopa (n=11 cells, N=3 mice) and average change in rotation bias during levodopa (inset). Statistical significance was determined using a Wilcoxon signed-rank test (D,F (right),G) or a one-way repeated measures ANOVA with a Tukey HSD post hoc analysis applied to correct for multiple comparisons (F (left, middle)); **p < 0.01, ***p < 0.001 (for ANOVA, only comparison between pre and saline shown, see Supplementary file 1, table 1 for detailed statistics). Arrowhead in velocity, GCaMP, and single-unit electrophysiology traces corresponds to 1 cm/s, 0 z-score, and 0 spike/s, respectively. Velocity traces, GCaMP traces, single-unit electrophysiology traces, and bar plots show mean ± SEM.
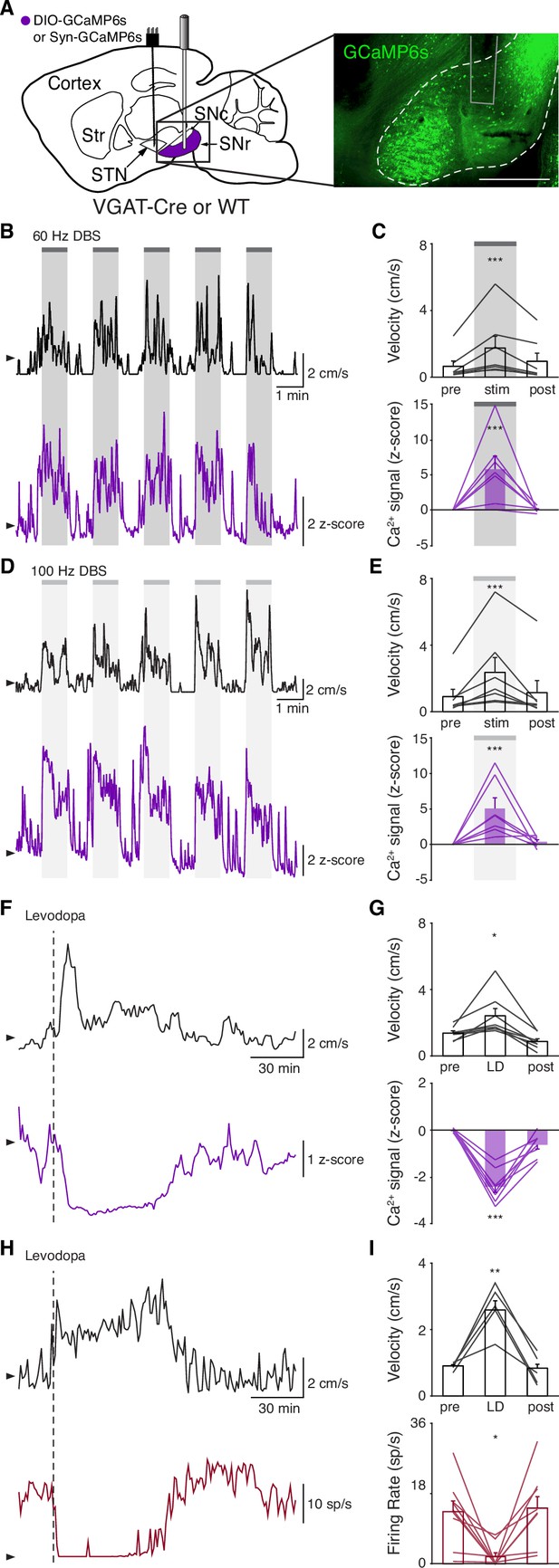
Subthalamic nucleus deep brain stimulation (STN DBS) increases basal ganglia output.
(A) Left: Sagittal schematic showing STN DBS and substantia nigra pars reticulata (SNr) GCaMP fiber photometry. Right: Postmortem sagittal section showing GCaMP expression and estimated fiber placement in the SNr (inset, scale = 500 μm). (B) Representative single-session velocity (black) and SNr GCaMP signal (purple) in response to 60 Hz STN DBS. (C) Average velocity (top) and SNr GCaMP signal (bottom) before, during, and after 60 Hz STN DBS (N=7 mice). (D) Representative single-session velocity (black) and SNr GCaMP signal (purple) in response to 100 Hz STN DBS. (E) Average velocity (top) and SNr GCaMP signal (bottom) before, during, and after 100 Hz STN DBS (N=7 mice). (F) Representative single-session velocity (black) and SNr GCaMP signal (purple) before and after levodopa injection (dotted line). (G) Average velocity (top) and SNr GCaMP signal (bottom) before, during, and after levodopa treatment (N=8 mice). (H) Representative single-session velocity (black) and SNr single-unit activity (red) before and after levodopa injection (dotted line). (I) Average velocity (top) and SNr single-unit activity (bottom) before, during, and after levodopa treatment (n=9 cells, N=3 mice). Statistical significance was determined using a one-way repeated measures ANOVA with a Tukey HSD post hoc analysis applied to correct for multiple comparisons; *p < 0.05, **p < 0.01, ***p < 0.001 (only comparison between pre and stim/LD shown, see Supplementary file 1, table 1 for detailed statistics). Arrowhead in velocity, GCaMP, and single-unit electrophysiology traces corresponds to 1 cm/s, 0 z-score, and 0 spike/s, respectively. Bar plots show mean ± SEM.
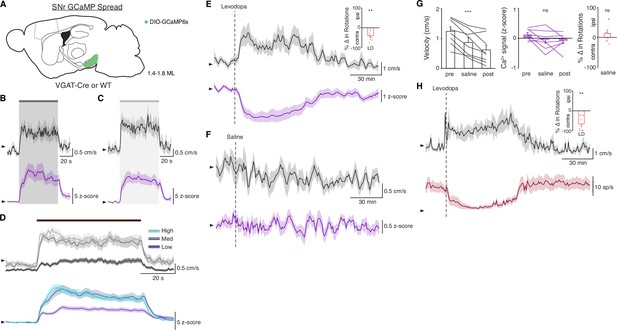
STN DBS evokes a rapid increase in SNr activity.
(A) Sagittal schematic showing estimated extent of viral GCaMP6s spread in the substantia nigra pars reticulata (SNr) of VGAT-Cre or wild-type (WT) mice (N=8 mice). (B–C) Average velocity over time (black) and SNr GCaMP signal (purple) following 60 Hz subthalamic nucleus deep brain stimulation (STN DBS) (B, N=7 mice) or 100 Hz STN DBS (C, N=7 mice). (D) Average movement velocity (top) and SNr GCaMP signal (bottom) during DBS sessions grouped by stimulation frequency: Low = 5–40 Hz, Med = 60–100 Hz, High = 120–180 Hz stimulation. (E) Average velocity over time (black) and SNr GCaMP signal (purple) following administration of levodopa (N=8 mice) and average change in rotation bias during levodopa (inset). (F) Average velocity over time (black) and SNr GCaMP signal (purple) following administration of saline (N=8 mice). (G) Average velocity (left), SNr GCaMP signal (middle), and change in rotation bias before, during, and after saline injection (N=8 mice). (H) Average velocity over time (black) and SNr single-unit firing rate (red) following administration of levodopa (n=9 cells, N=3 mice) and average change in rotation bias during levodopa (inset). Statistical significance was determined using a Wilcoxon signed-rank test (D,F (right),G) or a one-way repeated measures ANOVA with a Tukey HSD post hoc analysis applied to correct for multiple comparisons (F (left, middle)); **p < 0.01, ***p < 0.001 (for ANOVA, only comparison between pre and saline shown, see Supplementary file 1, table 1 for detailed statistics). Arrowhead in velocity, GCaMP, and single-unit electrophysiology traces corresponds to 1 cm/s, 0 z-score, and 0 spike/s, respectively. Velocity traces, GCaMP traces, single-unit electrophysiology traces, and bar plots show mean ± SEM.
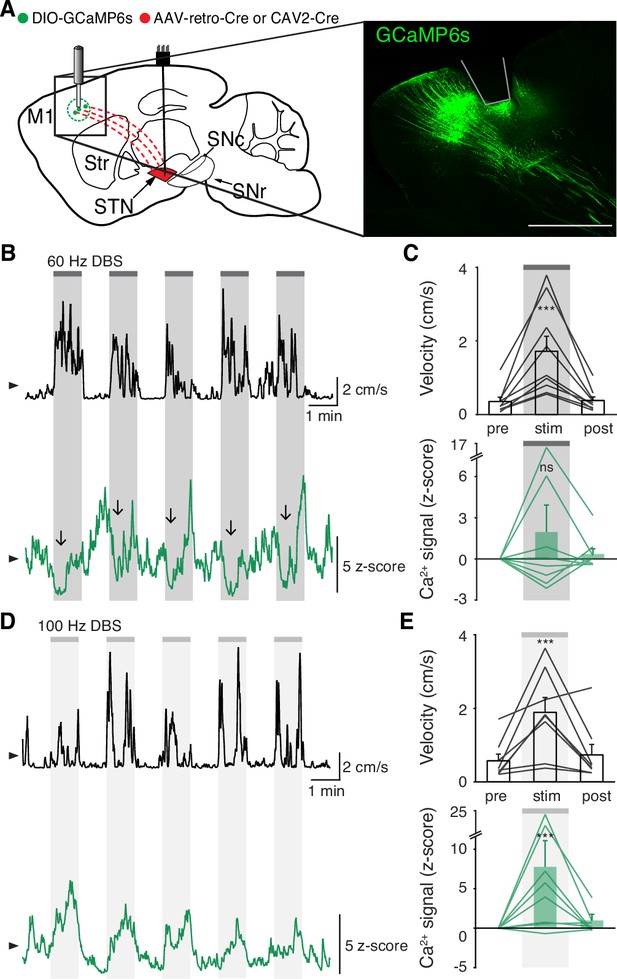
Subthalamic nucleus deep brain stimulation (STN DBS) variably changes hyperdirect M1 neural activity.
(A) Left: Sagittal schematic showing STN DBS and M1-STN GCaMP fiber photometry. Right: Postmortem sagittal section showing GCaMP expression and estimated fiber placement in M1 (inset, scale = 500 μm). (B) Representative single-session velocity (black) and M1-STN GCaMP signal (green) in response to 60 Hz STN DBS. (C) Average velocity (top) and M1-STN GCaMP signal (bottom) before, during, and after 60 Hz STN DBS (N=9 mice). (D) Representative single-session velocity (black) and M1-STN GCaMP signal (green) in response to 100 Hz STN DBS. (E) Average velocity (top) and M1-STN GCaMP signal (bottom) before, during, and after 100 Hz STN DBS (N=8 mice). Statistical significance was determined using a one-way repeated measures ANOVA with a Tukey HSD post hoc analysis applied to correct for multiple comparisons; ***p < 0.001 (only comparison between pre and stim shown, see Supplementary file 1, table 1 for detailed statistics). Arrowhead in velocity and GCaMP traces corresponds to 1 cm/s and 0 z-score, respectively. Bar plots show mean ± SEM.
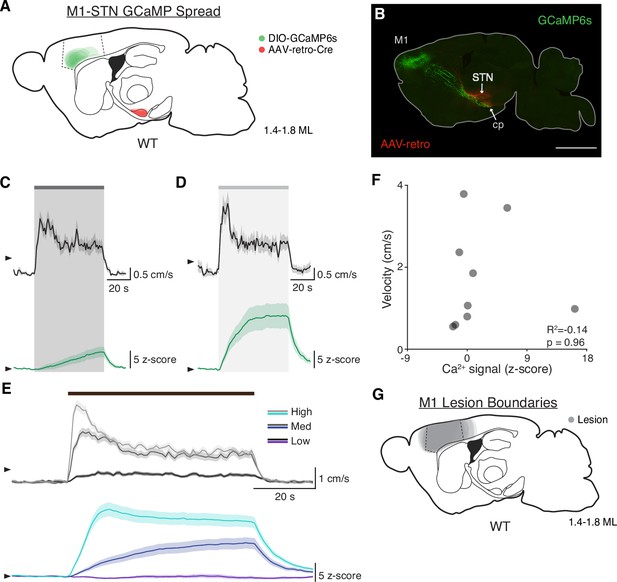
STN DBS drives variable and slow changes in hyperdirect M1 activity.
(A) Sagittal schematic showing estimated extent of viral AAV-retro and GCaMP6s spread in the subthalamic nucleus (STN) and M1, respectively, of wild-type (WT) mice (N=9 mice). (B) Postmortem sagittal section example showing AAV-retro expression in the STN (red), resulting in GCaMP6s expression in M1 neurons (green) projecting to the STN and cerebral peduncle (cp). (C–D) Average velocity over time (black) and M1-STN GCaMP signal (green) following 60 Hz STN DBS (C, N=9 mice) or 100 Hz STN DBS (D, N=8 mice). (E) Average movement velocity (top) and M1-STN GCaMP signal (bottom) during deep brain stimulation (DBS) sessions grouped by stimulation frequency: Low = 5–40 Hz, Med = 60–100 Hz, High = 120–180 Hz stimulation. (F) Scatter plot comparing hyperdirect M1 photometry signal to movement velocity during 60 Hz STN DBS (each dot represents average for one mouse, N=9 mice). (G) Sagittal schematic showing estimated extent of M1 lesions in WT mice (N=11 mice). Statistical significance was determined using a one-way ANOVA (see Supplementary file 1, table 1 for detailed statistics). Arrowhead in velocity traces and GCaMP traces corresponds to 1 cm/s and 0 z-score, respectively. Velocity and GCaMP traces show mean ± SEM.
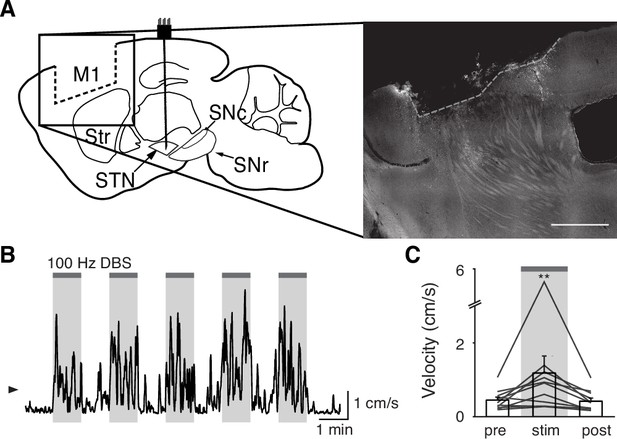
Surgical removal of M1 does not abolish therapeutic benefit of subthalamic nucleus deep brain stimulation (STN DBS).
(A) Left: Sagittal schematic showing STN DBS and M1 surgical lesion. Right: Postmortem sagittal section showing estimated extent of M1 lesion (inset, scale = 500 μm). (B) Representative single-session velocity of an M1-lesioned hemiparkinsonian mouse in response to 100 Hz STN DBS. (C) Average velocity before, during, and after 100 Hz STN DBS in M1-lesioned hemiparkinsonian mice (N=11 mice). Statistical significance was determined using a one-way repeated measures ANOVA with a Tukey HSD post hoc analysis applied to correct for multiple comparisons; **p < 0.01 (only comparison between pre and stim shown, see Supplementary file 1, table 1 for detailed statistics). Arrowhead in velocity trace corresponds to 1 cm/s. Bar plots show mean ± SEM.
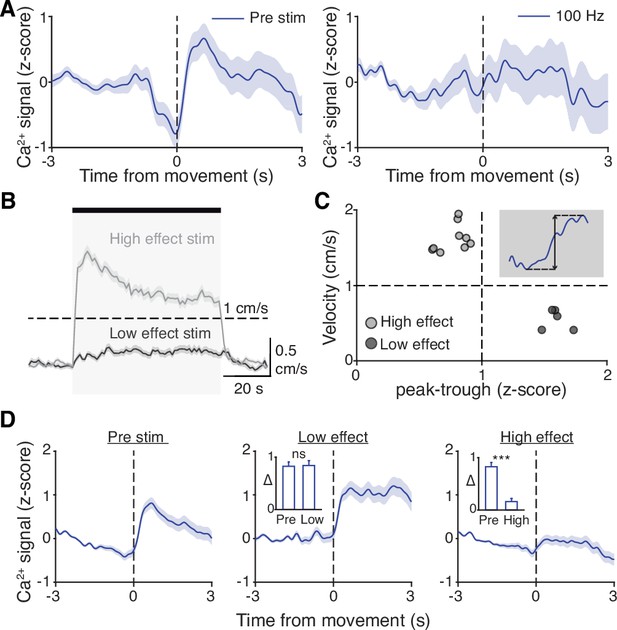
Subthalamic nucleus (STN) movement-related activity is disrupted by therapeutic STN deep brain stimulation (DBS).
(A) Average STN fiber photometry signal aligned to movement starts during pre-stim periods (left) and during 100 Hz STN DBS stimulation (right). (B) Average movement velocity over time during high effect stimulation (light gray, achieves average velocity >1 cm/s) and low effect stimulation (dark gray, achieves average velocity <1 cm/s). (C) Scatter plot comparing peak-trough distance in the average movement-aligned photometry trace (inset) to velocity during high effect (light gray) and low effect (dark gray) stimulation parameters. Each dot represents an average across all mice for each stimulation parameter (N=8 mice). (D) Average STN fiber photometry signal aligned to movement starts during pre-stim periods (left) and during low effect STN DBS stimulation (middle) and high effect STN DBS stimulation (right). Inset bar graphs show difference between average fiber signal in 1 s following movement start and 1 s preceding movement start (see Materials and methods for further details) during pre, low effect, or high effect stim. Statistical significance was determined using a Wilcoxon rank-sum test; ***p < 0.001 (see Supplementary file 1, table 1 for detailed statistics). Bar plots, photometry, and velocity traces show mean ± SEM.
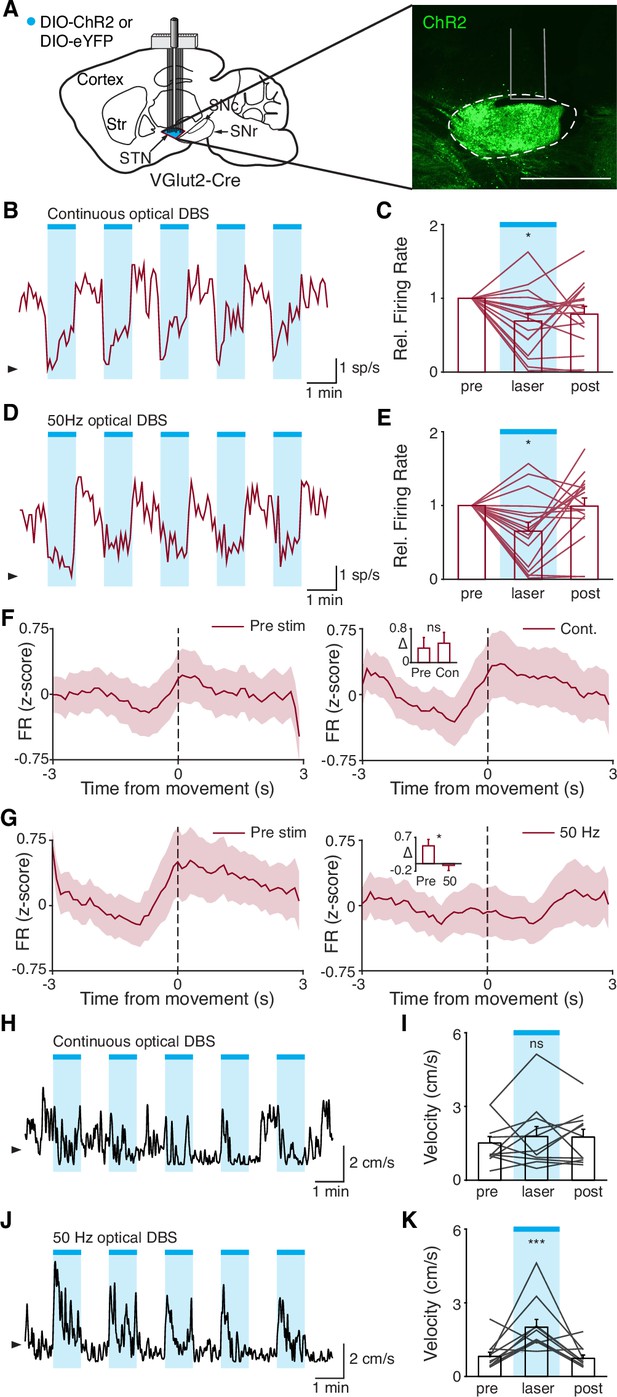
Disruption of movement-related subthalamic nucleus (STN) activity is sufficient to provide therapeutic benefit.
(A) Left: Sagittal schematic showing viral injection and 16-channel optrode implantation in the STN. Right: Postmortem sagittal section showing ChR2 expression in the STN (inset, scale = 500 μm). (B) Representative STN single-unit firing in response to continuous optical stimulation, given in 1 min epochs (blue bars). (C) Average z-scored firing rate of STN single units before, during, and after continuous optical stimulation (n=17 neurons, N=3 mice). (D) Representative STN single-unit firing in response to 50 Hz optical stimulation, given in 1 min epochs (blue bars). (E) Average z-scored firing rate of STN single units before, during, and after 50 Hz optical stimulation (n=17 neurons, N=3 mice). (F) Average z-scored firing rate of STN single units aligned to movement starts before (left) and during (right) continuous optical stimulation. Inset: average change in firing rate around movement starts (see Materials and methods for further details) before (pre) or during (con) constant optical stimulation (n=17 neurons, N=3 mice). (G) Average z-scored firing rate of STN single units aligned to movement starts before (left) and during (right) 50 Hz optical stimulation. Inset: average change in firing rate around movement starts before (pre) and during (50) 50 Hz optical stim (n=17 neurons, N=3 mice). (H) Representative single-session movement velocity in response to continuous optical stimulation, given in 1 min epochs. (I) Average velocity before, during, and after continuous optical stimulation (N=11 mice). (J) Representative single-session movement velocity in response to 50 Hz optical stimulation, given in 1 min epochs. (K) Average velocity before, during, and after 50 Hz optical stimulation (N=11 mice). Statistical significance was determined using a Wilcoxon rank-sum test (F–G) or a one-way repeated measures ANOVA with a Tukey HSD post hoc analysis applied to correct for multiple comparisons (C,E,I,K); *p<.05, ***p < 0.001 (for ANOVA, only comparison between pre and laser shown, see Supplementary file 1, table 1 for detailed statistics). Arrowhead in firing rate and velocity traces corresponds to 0 spike/s and 1 cm/s, respectively. Bar plots and average firing rate traces show mean ± SEM.
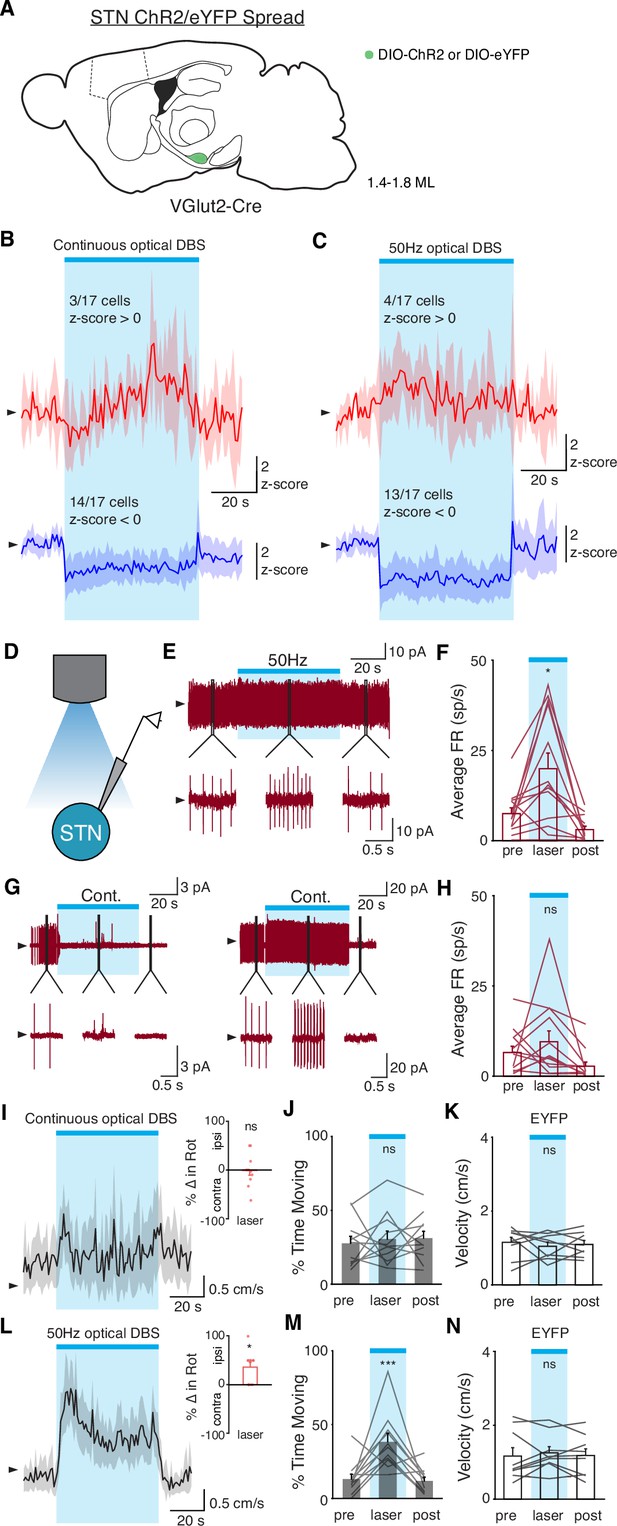
In vivo and ex vivo optical STN stimulation produces distinct changes in firing rate.
(A) Sagittal schematic showing estimated extent of viral ChR2 or eYFP spread in the subthalamic nucleus (STN) of VGlut2-Cre mice (N=20 mice). (B–C) Average in vivo firing rate of STN single units over time in response to continuous (B) or 50 Hz (C) optical stimulation (n=17 cells, N=3 mice), subdivided into neurons in which firing rate increased to greater than 0 z-score (top traces, red) and neurons in which firing rate decreased to less than 0 z-score (bottom traces, blue). (D) Recording configuration for ex vivo recordings of STN neurons in the cell-attached configuration. (E) Representative STN neuron before, during, and after 50 Hz optical stimulation. 1 s portions of the sweep are shown below. (F) Average firing rate before, during, and after 50 Hz optical stimulation (n=13 cells, N=3 mice). (G) Two STN neurons before, during, and after constant optical stimulation, demonstrating the variable response of neurons. 1 s portions of the sweep are shown below. (H) Average firing rate before, during, and after constant optical stimulation (n=13 cells, N=3 mice). (I) Average movement velocity and change in rotation bias (inset) in response to constant optical stimulation in ChR2 mice (N=11 mice). (J) Average percent time moving before, during, and after constant optical stimulation in ChR2 mice (N=11 mice). (K) Average movement velocity before, during, and after constant optical stimulation in mice injected with eYFP (N=9 mice). (L) Average movement velocity and change in rotation bias (inset) in response to 50 Hz optical stimulation in ChR2 mice (N=11 mice). (M) Average percent time moving before, during, and after 50 Hz optical stimulation in ChR2 mice (N=11 mice). (N) Average movement velocity before, during, and after 50 Hz optical stimulation in mice injected with eYFP (N=9 mice). Statistical significance was determined using a one-way repeated measures ANOVA with a Tukey HSD post hoc analysis applied to correct for multiple comparisons; *p < 0.05 (only comparison between pre and laser shown, see Supplementary file 1, table 1 for detailed statistics). Arrowhead in firing rate, cell-attached, and velocity traces corresponds to 0 z-score, 0 pA, and 1 cm/s, respectively. Firing rate traces, velocity traces, and bar plots show mean ± SEM.
Tables
Reagent type (species) or resource | Designation | Source or reference | Identifiers | Additional information |
---|---|---|---|---|
Genetic reagent (Mus musculus, C57Bl/6) | VGluT2-Cre | JAX | RRID IMSR_JAX:028863 | Hemizygous |
Genetic reagent (Mus musculus, C57Bl/6) | VGAT-Cre | JAX | RRID IMSR_JAX:016962 | Hemizygous |
Strain, strain background (Mus musculus, C57Bl/6) | C57Bl/6J | JAX | RRID IMSR_JAX:000664 | Wild-type |
Genetic reagent (AAV) | AAV1-Syn-Flex-GCaMP6s-WPRE-SV40 | Addgene | RRID:Addgene_100845 | Diluted 1:8 in normal saline |
Genetic reagent (AAV) | AAV2retro-Cre-mCherry | Addgene | RRID:Addgene_55632 | Undiluted |
Genetic reagent (CAV) | CAV-Cre | Montpellier | CAV-Cre | Undiluted |
Genetic reagent (AAV) | AAV5-DIO-ChR2-eYFP | Addgene | RRID:Addgene_20298 | Diluted 1:2 in normal saline |
Genetic reagent (AAV) | AAV5-DIO-eYFP | UNC Vector Core | No. order # available | Undiluted |
Other | 16-Channel 7 mm fixed electrode array | Innovative Neurophysiology | No. catalog # | Tungsten microwire array |
Other | Master8 | AMPI | RRID:SCR_018889 | Stimulus pattern generator |
Antibody | Anti-tyrosine hydroxylase (rabbit polyclonal) | Pel-Freez | Order # P40101-150 RRID:AB_2617184 | lot #: AJ0818B; (1:1000) dilution |
Antibody | Anti-tyrosine hydroxylase (chicken polyclonal) | Millipore | Order # AB9702 RRID:AB_570923 | lot #: 3152195; (1:1000) dilution |
Software, algorithm | Plexon Offline Sorter | Plexon | RRID:SCR_000012 | |
Software, algorithm | Ethovision | Noldus | RRID:SCR_000441 | |
Software, algorithm | IgorPro | Wavemetrics | RRID:SCR_000325 | With MafPC: https://www.xufriedman.org/mafpc |
Software, algorithm | Matlab | Mathworks | RRID:SCR_001622 | v.2019a |
Additional files
-
Supplementary file 1
(a) Full statistical results for the indicated figures. rmANOVA = one-way repeated measures analysis of variance with post hoc Tukey multiple comparisons test. WRS = Wilcoxon rank-sum test (Mann-Whitney U test). FT = Friedman’s test with post hoc Tukey multiple comparisons test. WSR = Wilcoxon signed-rank test. (b) Stimulation parameters used in assessing differences between low-, medium-, and high-frequency stimulation. (c) Stimulation parameters used in assessing differences between high and low effect stimulation.
- https://cdn.elifesciences.org/articles/75253/elife-75253-supp1-v2.docx
-
Transparent reporting form
- https://cdn.elifesciences.org/articles/75253/elife-75253-transrepform1-v2.docx