Foveal vision anticipates defining features of eye movement targets
Figures
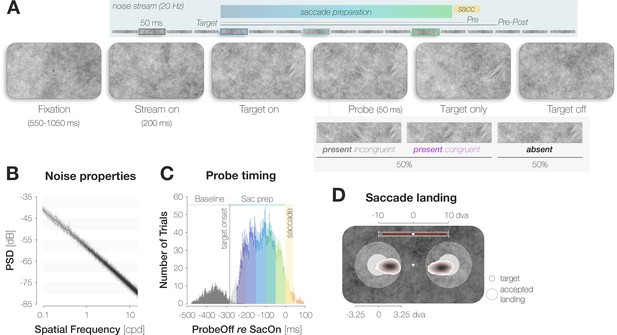
A dynamic noise paradigm probing foveal sensitivity to saccade target features.
(A) Example trial procedure in Experiment 1: The saccade target and foveal probe were embedded in full-screen noise images flickering at a frequency of 20 Hz (image duration of 50ms). After 200ms, the saccade target (an orientation-filtered patch; filtered to either –45° or +45°; 3 dva in diameter) appeared 10 dva to the left or the right of the screen center, cueing the eye movement. On 50% of trials, a probe (a second orientation-filtered patch; filtered to either –45° or +45°) appeared in the screen center either 150ms before target onset (top panel; highlighted element with grey outline), or at an early (dark blue outline), medium (light blue outline) or late (green outline) stage of saccade preparation. The foveal probe was presented for 50ms and could be oriented either congruently or incongruently to the target. On Pre trials, the saccade target disappeared before saccade landing. On Pre-Post trials, it remained visible for a brief duration after landing. Observers reported if they had perceived the probe in the screen center or not (present vs absent). After a ‘present’ response, they reported the probe’s perceived orientation (left for –45° vs right for +45°). (B) Noise properties: Power spectral density (PSD) of the foveal region (3 dva diameter) of all noise images presented in a randomly chosen experimental session. (C) Probe timing: histogram of time intervals between probe offset and saccade onset. Bar heights and error bars indicate the mean and standard error of the mean (SEM; n=7) across observers, respectively. On baseline trials, the probe appeared before target onset (dark grey bars). On all remaining trials, the probe appeared after target onset and therefore during saccade preparation (Sac prep). We assigned saccade preparation trials to five distinct time bins (from dark blue to light green). Trials in which the probe disappeared more than 250ms before saccade onset (light grey), during the saccade (yellow) or after saccade offset (orange) were excluded. The yellow background rectangle illustrates the median saccade duration. (D) Observers were able to select the peripheral stimulus as the saccade target: Bivariate Gaussian Kernel densities of saccade landing coordinates for left- and rightwards saccades. Filled circles indicate the saccade target (rad = 1.5 dva). Transparent circles indicate the accepted landing area (rad = 3.25 dva). The distance between the screen center and the targets was reduced for illustration purposes. Red bars on top indicate median saccade amplitudes based on both the horizontal and vertical component of the saccade. Neither saccade latencies nor saccade endpoints influenced congruency effects (see Materials and methods).
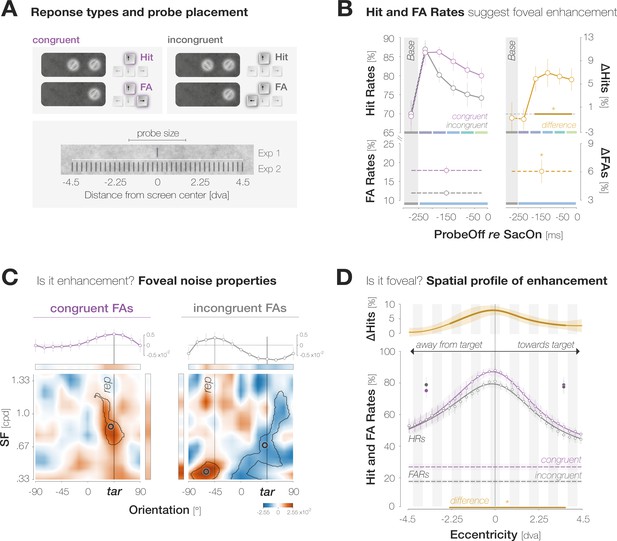
Evidence for predictive foveal enhancement of saccade target features.
(A)Top: Response types. (In)congruent Hits are probe-present trials in which the probe was oriented (in)congruently to the target and observers reported ‘present’ (up arrow key). (In)congruent FAs are probe-absent trials in which observers reported the presence (up arrow key) of a probe with target-(in)congruent orientation (left, right arrow keys). Bottom: Probe placement. In Experiment 1, we chose a single probe location in the screen center. In Experiment 2, we defined 37 probe locations spaced evenly on a horizontal axis of 9 dva length around the screen center to measure the spatial profile of enhancement. (B) Hit and FA rates in Experiment 1 (y-axis) for different pre-saccadic time bins (x-axis; dark blue to light green rectangles), separated into congruent (purple) and incongruent (gray) responses. The difference between congruencies is plotted in brown. Asterisks indicate p-values ≤ .05 (obtained with bootstrapping, see Materials and methods). (C) Foveal noise properties on congruent and incongruent FA trials. We described the energy of 260 combinations of orientations (x-axis) and spatial frequency (SF; y-axis) in the foveal noise region (see Materials and methods). Noise images corresponding to trials with leftward target orientation were flipped, such that +45° corresponds to the target orientation (‘tar’). The reported orientation (‘rep’) was either +45° (congruent FAs) or –45° (incongruent FAs). Orange regions indicate positive energy values in the standardized energy map (z-score >0) whereas blue regions indicate negative values (z-score <0). Marginal means along the orientation axis (curves and horizontal colored bars) are averages of energy values across all SFs. Marginal means along the SF axis (vertical colored bars) are averages of all SFs within the horizontal boundaries of the cluster around the reported orientation. Open circles indicate the center of mass of identified clusters. (D) Hit and FA rates in Experiment 2 (y-axis) for locations horizontally offset from the fovea (x-axis). Note that FAs cannot be spatially resolved. Data points indicate mean response rates across observers. The plotted curves are average Gaussian function fits to individual-observer means after aligning them to the mean recorded fixation position during saccade preparation (zero on the x-axis). Negative and positive x-axis values indicate probe locations away from and towards the saccade target, respectively. Thick brown lines highlight the significant portion of the spatial difference curve (congruent HRs – incongruent HRs; top panel; obtained with bootstrapping). Additional data points at ±3 dva eccentricity were measured by raising peripheral performance to a foveal level by adaptively increasing probe transparency (last session of Experiment 2). All error bands and bars denote ±1 SEM (n=7 in B and C; n=9 in D).
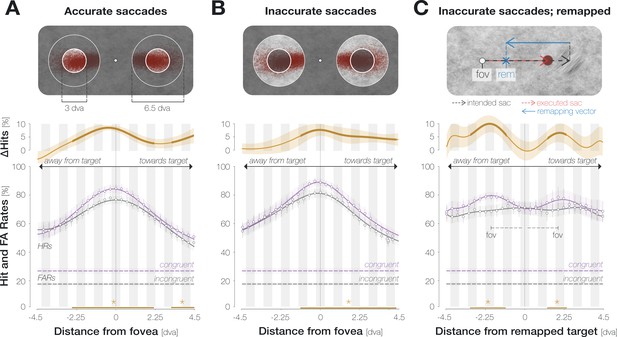
Enhancement is aligned to the center of gaze, not to the remapped target location.
(A) Spatial HR profiles for the subset of trials in which the saccade landed on the target (‘accurate’; see top panel). (B) Spatial profiles for saccades that landed off-target but within the accepted landing area (‘inaccurate’; see top panel). In (A) and (B), zero on the x-axis corresponds to the recorded pre-saccadic center of gaze. The top panels illustrate the endpoints of all included saccades separately for leftwards and rightwards eye movements. (C) Spatial profiles for inaccurate saccades when probe locations were aligned to the remapped target location rather than to the center of gaze. The top panel illustrates a trial in which an observer is fixating on the fixation dot (‘fov’) and planning a saccade to the center of the target stimulus (‘intended sac’; black dashed arrow). The observer executes a hypometric saccade (red arrow), the endpoint of which is illustrated as a red disk. The remapping vector (blue arrow) is obtained by flipping the executed saccade vector and attaching it to the intended saccade endpoint, i.e., to the center of the target (Collins et al., 2009). In all spatial profiles in (C), zero on the x-axis indicates the remapped target location (blue X), that is, the location that the saccade target would occupy upon saccade landing. With this alignment, we observed two peripheral maxima that correspond to the mean center of gaze (‘fov’). All conventions for the spatial profiles follow those of Figure 2D. Remapping-aligned curves for all trials and accurate saccades only are provided in the Supplements (Appendix 1—figure 8).
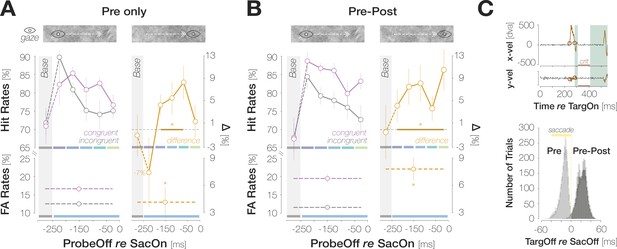
Post-saccadic target foveation boosts congruency.
(A) Foveal Hit Rates (top row) and FA Rates (bottom row) for the subset of trials in which the saccade target had disappeared during saccadic flight (‘Pre-only’). (B) Foveal Hit Rates (top row) and FA Rates (bottom row) for the subset of trials in which the saccade target had remained visible briefly after saccade landing (right column; ‘Pre-Post’). Conventions in (A) and (B) are as in Figure 2B. (C) Top: Illustration of saccade inclusion criteria based on horizontal and vertical eye velocities (x vel; y vel) recorded in an example trial. Orange outlines highlight offline-detected saccadic events. To ensure that foveal input remained stable on the retina after landing, we excluded trials in which a second saccade occurred in a critical time window (‘crit’) between 25 and 100ms after response saccade offset. We employed a conservative estimate of saccade offset and excluded post-saccadic oscillations from the saccadic profile. We therefore introduced a short time window of 25ms after response saccade offset during which saccadic activity did not lead to trial exclusions. Bottom: Histogram of time intervals between target offset and saccade offset. On Pre trials, the target disappeared ~14.1ms (median) before saccade landing. On Pre-Post trials, the target remained visible for ~22.3ms (median) after saccade landing.
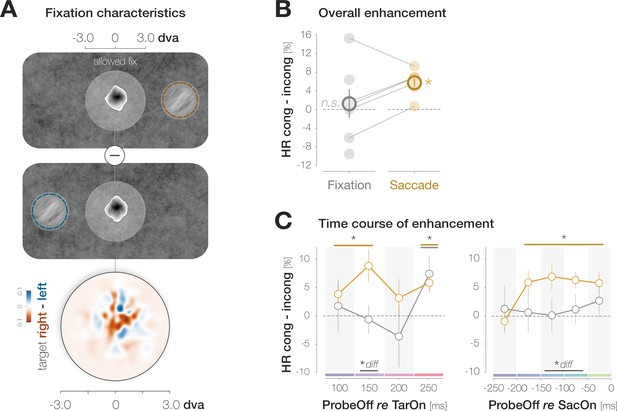
Saccade preparation accelerates foveal enhancement.
(A) Observers’ mean fixation densities when the target was presented to the right (first panel; orange) or the left (second panel; blue) of the screen center. The difference plot (third panel) reveals no systematic biases in gaze direction. The distance between the allowed fixation area around the screen center (light disk) and the target is reduced for illustration purposes. (B) Mean enhancement across all target-probe presentation intervals for the fixation (gray symbols) and saccade experiment (Experiment 1; orange symbols). Saccade data were collected in Experiment 1. Filled disks correspond to individual observers. Lines connect the data points of observers who participated in both the saccade and fixation experiment (n=5). (C) Time course of enhancement locked to target onset (left) or saccade onset (right). As in (B) orange symbols correspond to saccade data while gray symbols correspond to fixation data. The orange curve in the second panel is identical to the difference curve plotted in Figure 2B. Across panels, error bars denote ±1 SEM (n=7 for saccade and fixation data, respectively). Horizontal lines and asterisks above the data points highlight comparisons to zero with a p ≤ 0.05 for the fixation (gray) and saccade (orange) condition (obtained with bootstrapping). Horizontal lines and asterisks below the data points highlight bins in which the saccade and fixation condition differed significantly (‘diff’).
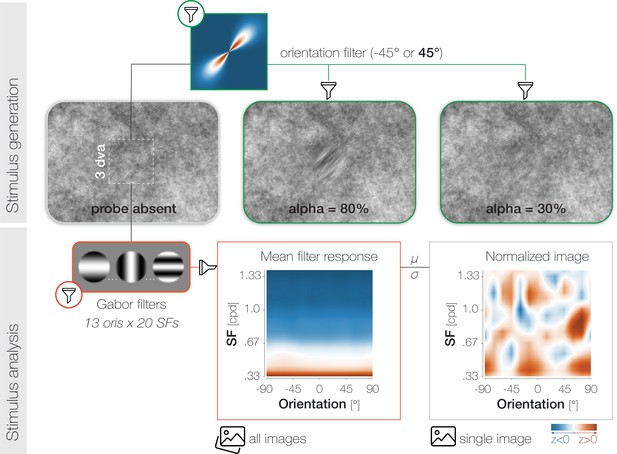
Stimulus generation (top) and analysis (bottom).
To generate probe and target patches, we applied smooth orientation filters (green lines) to the relevant regions of the respective noise image. The 2D Fourier transform of a 45° filter is plotted for illustration. To adjust task difficulty for individual observers and different experimental purposes, we varied the opacity α (1-transparency) of the probe. High α-values increase visibility (see α=80%) while low α-values decrease visibility (see α=30%). Note that the calibration of the displaying device (in our case the projector) and the constant stimulus flicker in our experiment influence the visibility of the probe stimulus and should be considered when judging the displayed examples. To analyze the properties of the foveal noise window on probe absent trials, we computed its dot product with 260 Gabor filters with varying orientation (ori)*spatial frequency (SF) characteristics (yellow outlines). The resulting mean filter response across all images shows high energy for low-SF information (orange) and low energy for higher SFs (blue). To account for the asymmetry in power across SFs, we normalized every image by the mean (μ) and standard deviation (σ) of the set of images presented in a given experimental session for a given observer.
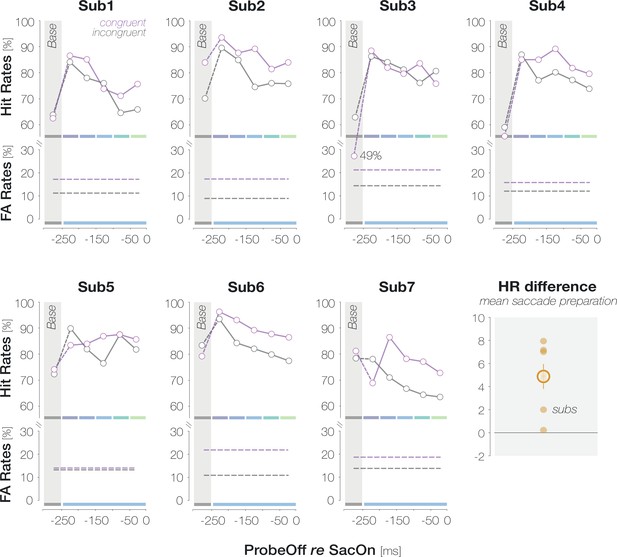
Congruency effects in HRs and FARs are fairly consistent across observers.
Individual observer plots follow the conventions of Figure 2B. The last panel summarizes the congruency effect in HRs. Each dot corresponds to the mean enhancement (HRcong-incong) across saccade preparation (all bins except baseline). The orange circle indicates the mean across observers. The error bar denotes ±1 SEM (n=7).
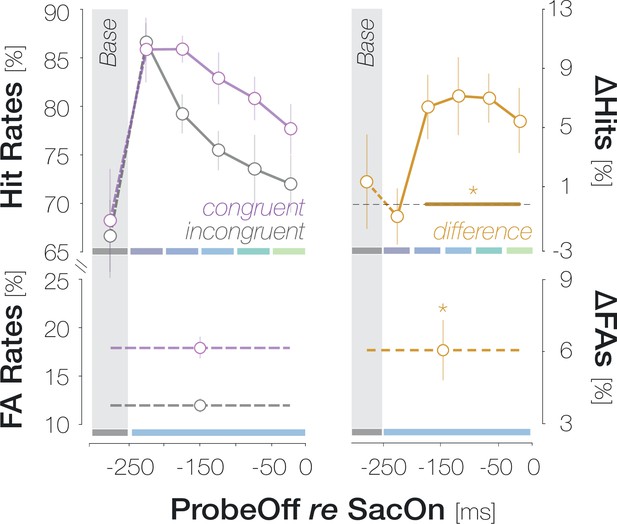
Taking the accuracy of the orientation report on Hit trials into account does not change the nature of findings.
All conventions follow those of Figure 2B.
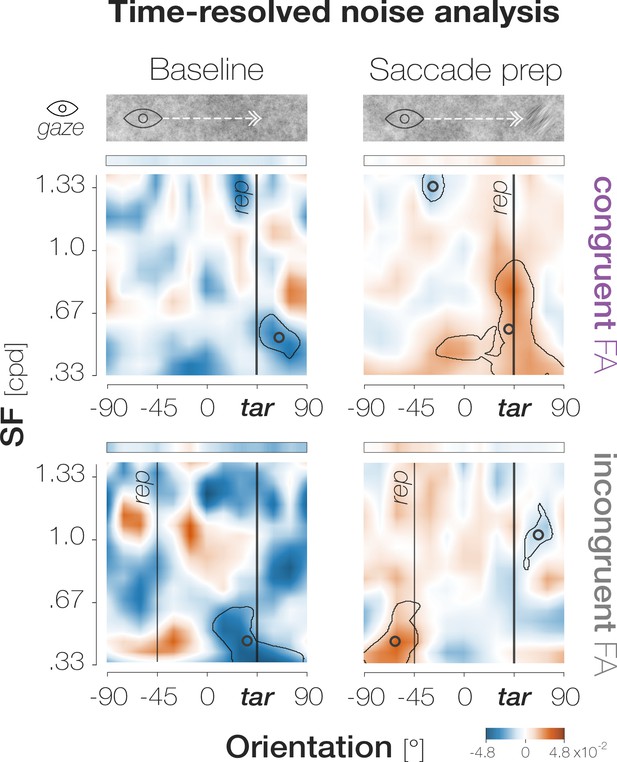
Higher-SF clusters around the target orientation manifest exclusively during saccade preparation.
On every trial, we separated all presented noise images into those that had appeared before saccade target onset (‘Baseline’; left column) and after saccade preparation had been initiated by the appearance of the target (‘Saccade prep’; right column). For both congruent (top row) and incongruent (bottom row) FAs, clusters that include target-like orientations in a higher SF range are observable exclusively during saccade preparation (orange cluster indicating high energy for congruent FAs, blue cluster indicating low energy for incongruent FAs). See main text for further explanations. All conventions follow those of Figure 2C.
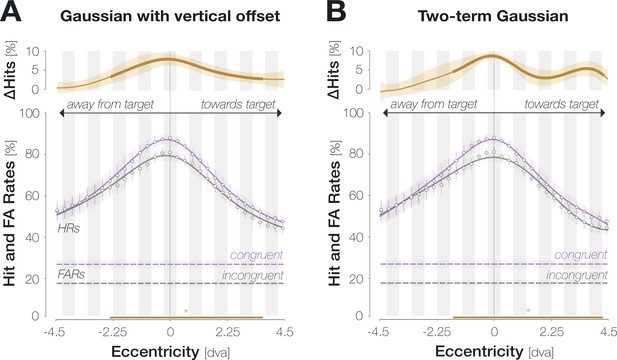
Gaussian functions with vertical offsets (A) and two-term Gaussian functions (B) yield comparable fits to spatial HR profiles.
Conventions as in Figure 2D.
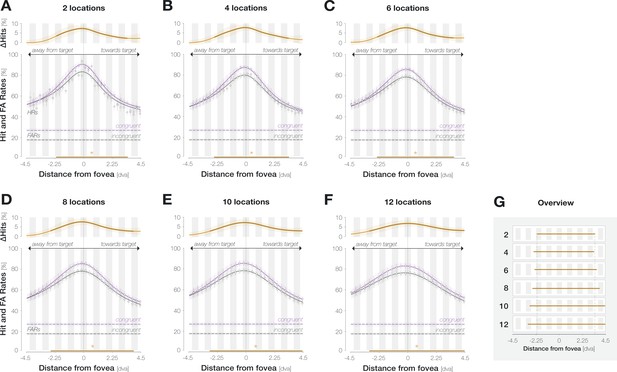
Spatial profiles for different widths of the moving boxcar window (i.e., different numbers of locations combined at each iteration).
As the overview in (G) illustrates, the measured width of enhancement increases with the width of the moving window (row numbers). We used a window width of seven locations for the main analysis in Figure 2D and a width of nine locations when separating trials by saccade landing accuracy in Figure 3.
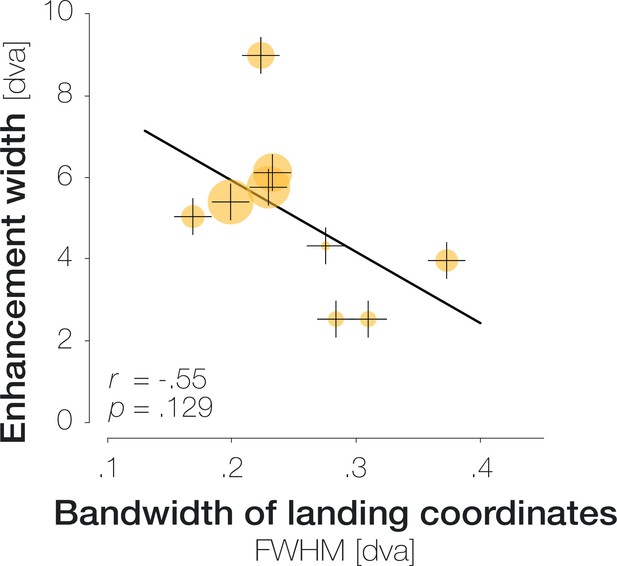
Relation between the imprecision of saccade endpoints (x-axis) and the width of the enhanced region (y-axis) in Experiment 2.
Saccadic imprecision was defined as the bandwidth of the horizontal Gaussian kernel fitted to all saccade endpoints of a specific observer. X-axis values represent the kernel’s full width at half maximum (FWHM) in dva. Enhancement width was defined as the maximum number of consecutive locations with significant enhancement. Orange disks correspond to individual observers. The size of each disk expresses the mean enhancement per significant data point (from 3.2% to 10.9%). The black line indicates a least-squares linear fit to the data.
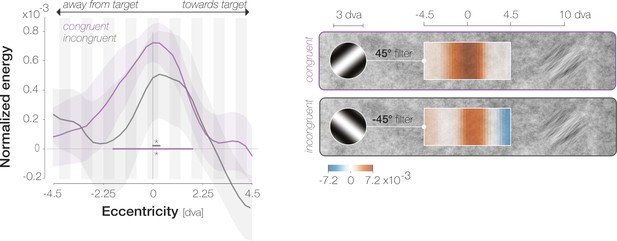
FAs are triggered by foveal orientation information.
Left: Normalized energy around the reported orientation (+45° for congruent FAs, purple; –45° for incongruent FAs, gray) across spatial locations. Significance indicators highlight the spatial region in which the curve with corresponding color differs from zero. All further conventions follow those of Figure 2D. Right: Spatial profiles overlaid on the filtered region (drawn to scale with respect to the saccade target). Filters illustrate the relevant orientation in an example SF.
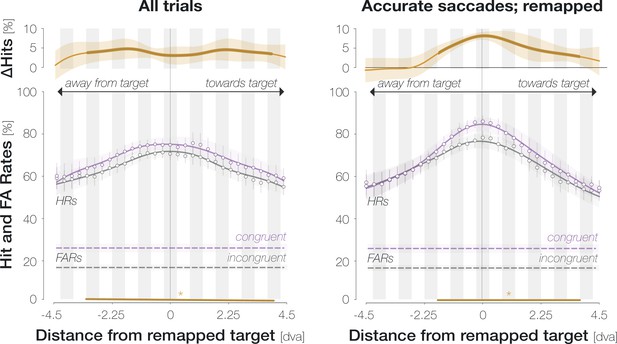
Spatial HR profiles aligned to the remapped location for all trials (left) and for the subset of accurate saccades that landed on the target (right).
All conventions are as in Figure 2D.
Videos
FAs are primarily triggered by foveal orientation information.
Filter responses of noise images underlying incongruent (A; the non-target orientation was reported) and congruent (B; the target orientation was reported) FAs at different spatial locations. The axis on top indicates the spatial range of filter locations (from –4.5–4.5 dva). The moving slider highlights the filter location corresponding to the currently displayed energy map. All further conventions are as in Figure 2C. For both FA types, the energy around the reported orientation is particularly high in and around the foveal region.