Natural forgetting reversibly modulates engram expression
Figures
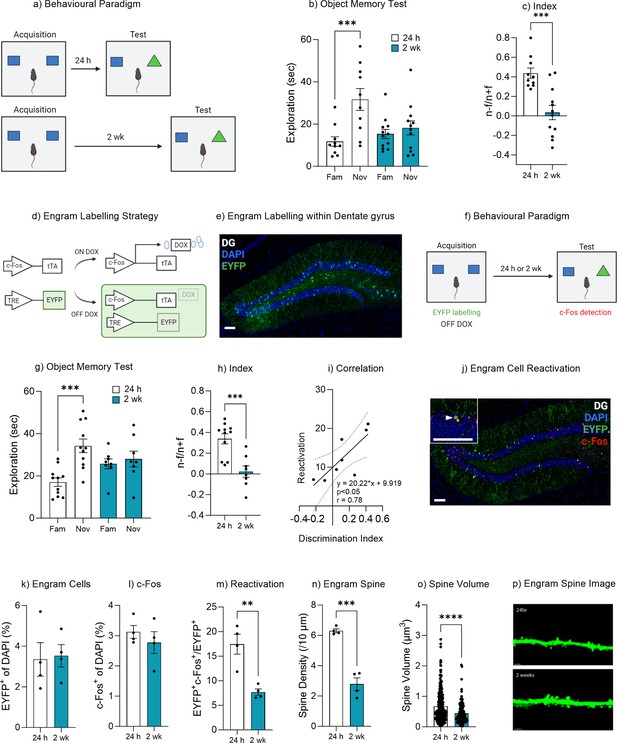
Natural forgetting of an object memory.
(a) Object recognition paradigm. (b) Object memory test for recall at 24 hr or 2 weeks. (c) Discrimination index. (d) Schematic of Fos-tTA engram labeling system. (e) Representative image of engram labeling within the dentate gyrus. (f) Engram labeling of object memory and c-Fos detection following recall. (g) Object memory test for recall at 24 hr or 2 weeks. (h) Discrimination index. (i) Correlation between discrimination index and engram reactivation. (j) Representative image eYFP+ cells, c-Fos+ cells and merged eYFP+ and c-Fos+ for both 24 hr and 2 week test. (k) Engram cells. (l) c-Fos+ cells. (m) Engram reactivation. (n) Engram spine density average per mouse. (o) Engram spine volume average per dendrite. (p) Representative image of engram dendrite for morphological analysis. Bar graphs indicate average values in n=4–11 per group (**p<0.01, ***p<0.001). Data graphed as means ± SEM. Scale bar 100 µm. Panel a was created with BioRender.com. Panel f was created with BioRender.com.
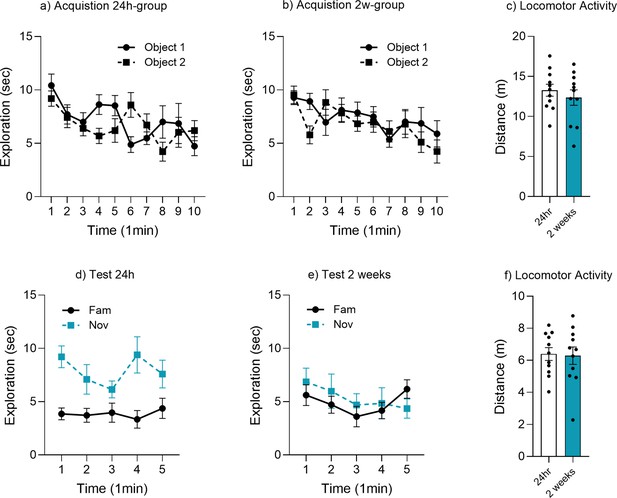
Object memory time bin analysis.
Minute-by-minute analysis of object exploration for (a) acquisition 24 hr group, (b) acquisition 2 week group, (c) locomotor activity acquisition, (d) test at 24 hr, (e) test at 2 weeks, and (f) locomotor activity test.
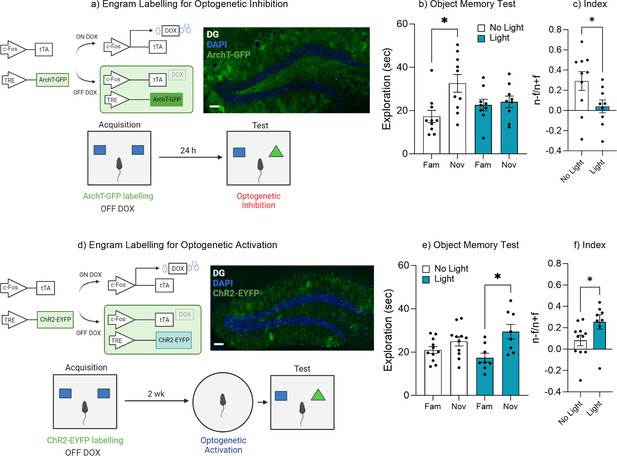
Dentate gyrus engrams are necessary and sufficient for recall of an object memory.
(a) Engram labeling for optogenetic inhibition and behavioral timeline. (b) Object memory test. (c) Discrimination index. (d) Engram labeling for optogenetic activation and behavioral timeline. (e) Object memory test. (f) Discrimination index. Bar graphs indicate average values in n=9–12 per group (*p<0.05). Data graphed as means ± SEM. Scale bar 100 µm. Panel a was created with BioRender.com. Panel d was created with BioRender.com.
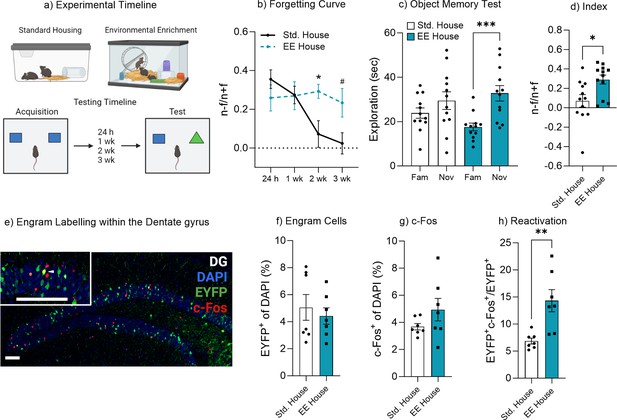
Environmental enrichment reduces the rate of forgetting by increasing engram activation.
(a) Enrichment behavioral paradigm. (b) Natural forgetting curve of an object memory at 24 hr, 1 week, 2 week, and 3 week. (c) Object memory test. (d) Discrimination index. (e) Engram labeling within the dentate gyrus. (f) Engram cells. (g) c-Fos+ cells. (h) Engram reactivation. Bar graphs indicate average values in n=4–12 per group (*p<0.05, ***p<0.001). Data graphed as means ± SEM. Scale bar 100 µm. Panel a was created with BioRender.com.
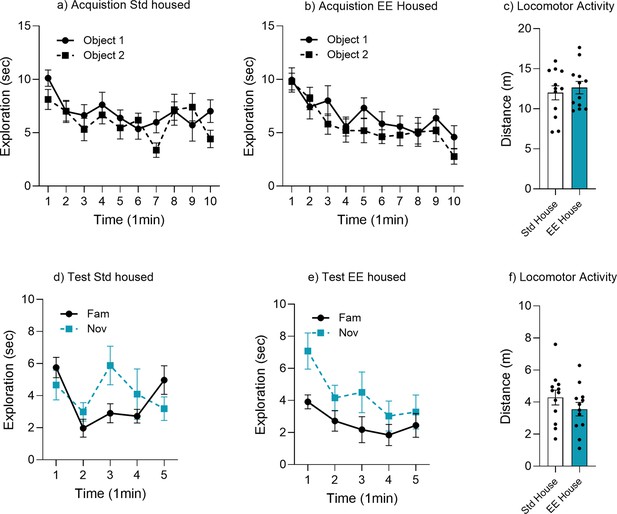
Object memory time bin analysis for environmental enrichment (EE).
Minute-by-minute analysis of object exploration for (a) acquisition standard (Std) housed, (b) acquisition EE housed, (c) locomotor activity acquisition, (d) test Std housed, (e) test EE housed weeks, and (f) locomotor activity test.
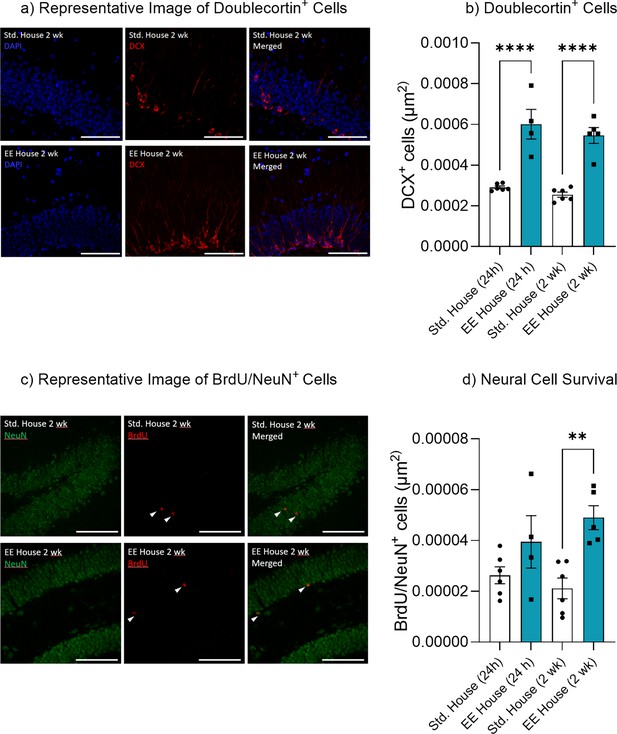
Environmental enrichment increased hippocampal neurogenesis.
(a) Doublecortin+ cells. (b) Representative image of Doublecortin+ cells. (c) Neural cell survival. (d) Representative image of BrdU/NeuN+ cells.
Bar graphs indicate average values in n = 4–6 per group (*p<0.05, **p<0.01, ***p<0.001). Data graphed as means ± SEM. Scale bar 100 µm.
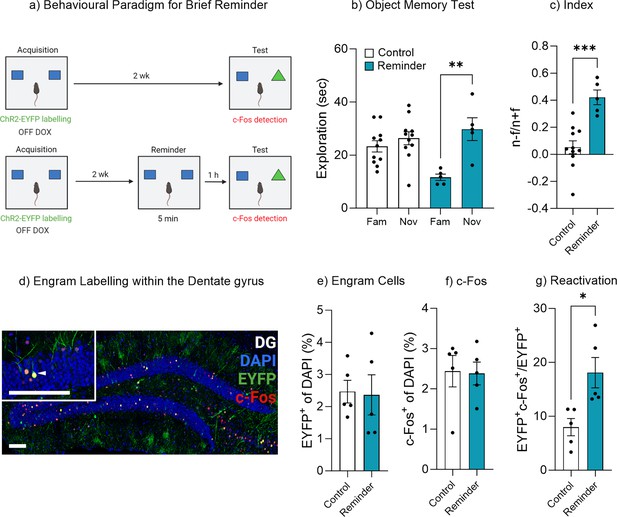
Exposure to the original stimuli facilities memory recall.
(a) Behavioral timeline for a brief reminder exposure. (b) Object memory test. (c) Discrimination index. (d) Engram labeling within the dentate gyrus. (e) Engram cells. (f) c-Fos+ cells. (g) Engram reactivation. Bar graphs indicate average values in n=5–11 per group (*p<0.05, **p<0.01, ***p<0.001). Data graphed as means ± SEM. Scale bars 100 µm. Panel a was created with BioRender.com.
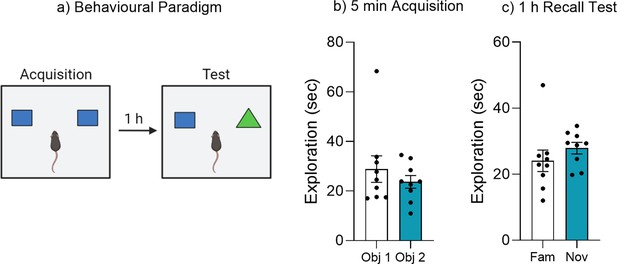
A single brief acquisition does not produce a lasting object memory.
(a) Behavioral paradigm for brief reminder trial. (b) Object exploration during 5 min acquisition training. (c) Object exploration during 1 hr recall test. Bar graphs indicate average values in n = 9 per group. Data graphed as means ± SEM. Panel a was created with BioRender.com.
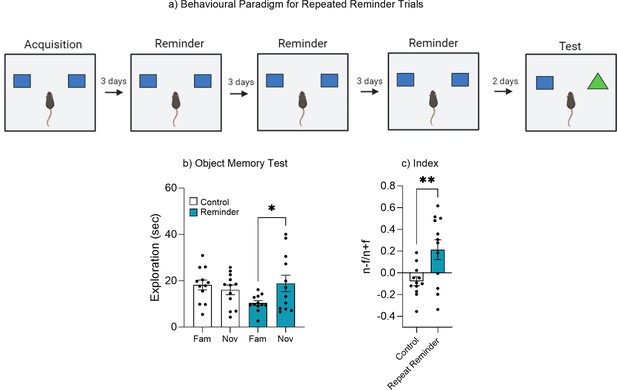
Repeated reminder exposure.
(a) Behavioral paradigm. (b) Object memory test. (c) Discrimination index. Bar graphs indicate average values in n = 12 per group (*p<0.05, **p<0.01). Data graphed as means ± SEM. Panel a was created with BioRender.com.
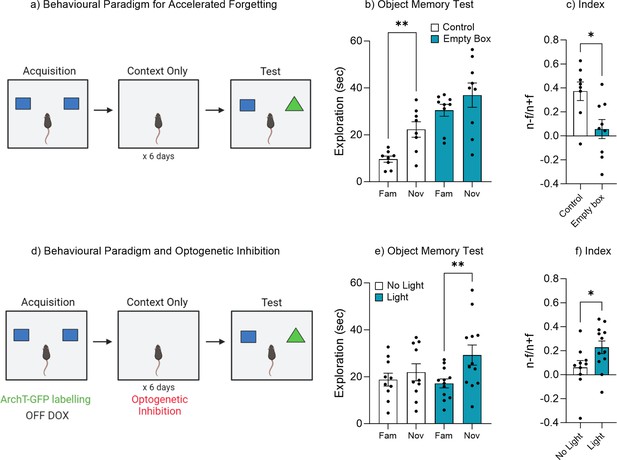
Repeated exposure to the training environment facilitates forgetting.
(a) Behavioral paradigm. (b) Object memory test. (c) Discrimination index. (d) Behavioral paradigm for optogenetic inhibition during repeated context exposure. (e) Object memory test. (f) Discrimination index. Bar graphs indicate average values in n=8–12 per group (*p<0.05, **p<0.01). Data graphed as means ± SEM. Panel a was created with BioRender.com. Panel d was created with BioRender.com.
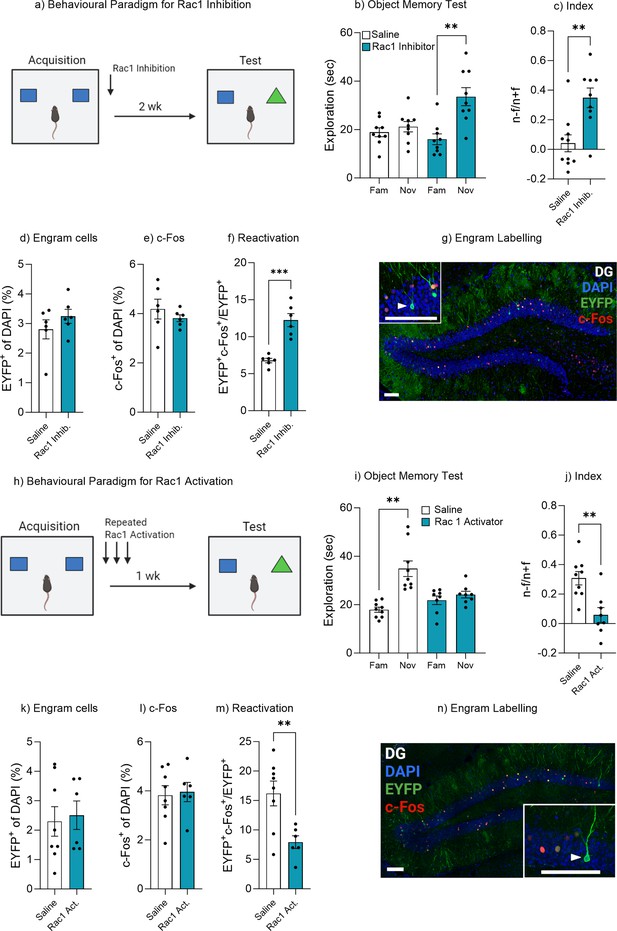
Rac1 modulates forgetting through engram activation.
(a) Experimental timeline and drug administration for Rac1 inhibition. (b) Object memory test. (c) Discrimination index. (d) Engram cells. (e) c-Fos+ cells. (f) Engram reactivation. (h) Engram labeling within the dentate gyrus. (i) Experimental timeline and drug administration for Rac1 inhibition. (j) Object memory test. (k) Discrimination index. (l) Engram cells. (m) c-Fos+ cells. (n) Engram reactivation. Bar graphs indicate average values in n=8–9 per group (**p<0.01, ***p<0.001). Data graphed as means ± SEM. Scale bar 100 µm. Panel a was created with BioRender.com. Panel h was created with BioRender.com.
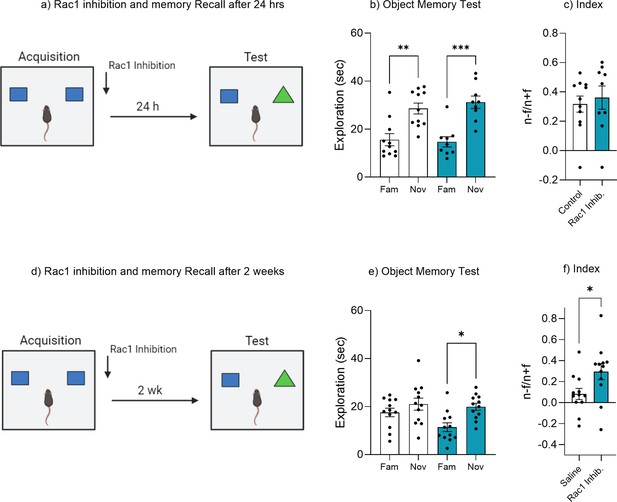
Rac1 inhibition prevents forgetting but does not enhance recognition memory.
(a) Experimental timeline and drug administration for Rac1 inhibition in wild-type mice tested 24 hr recall. (b) Object memory test at 24 hr. (c) Discrimination index. (d) Experimental timeline and drug administration for Rac1 inhibition in wild-type mice tested 2 weeks recall. (e) Object memory test at 2 weeks. (f) Discrimination index. Bar graphs indicate average values in n = 9–12 per group (*p<0.05, **p<0.01, ***p<0.001). Data graphed as means ± SEM. Panel a was created with BioRender.com. Panel d was created with BioRender.com.
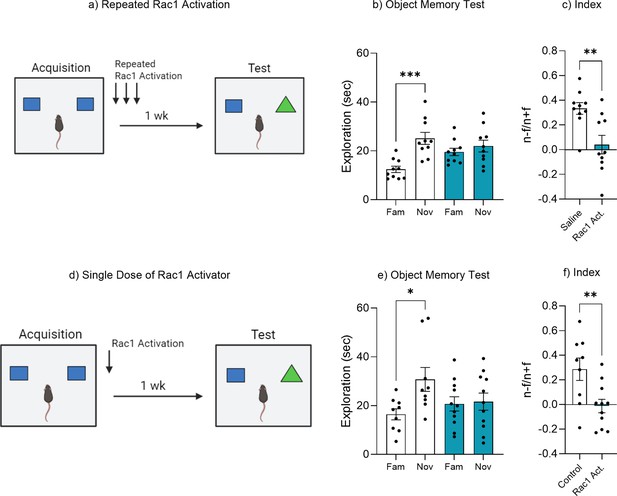
Rac1 activation accelerates forgetting.
(a) Experimental timeline and drug administration for repeated Rac1 activation in wild-type mice. (b) Object memory test. (c) Discrimination index. (d) Experimental timeline and drug administration for single dose of Rac1 activator in wild-type mice. (e) Object memory test. (f) Discrimination index. Bar graphs indicate average values in n = 9–11 per group (*p<0.05, **p<0.01, ***p<0.001). Data graphed as means ± SEM. Panel a was created with BioRender.com. Panel d was created with BioRender.com.
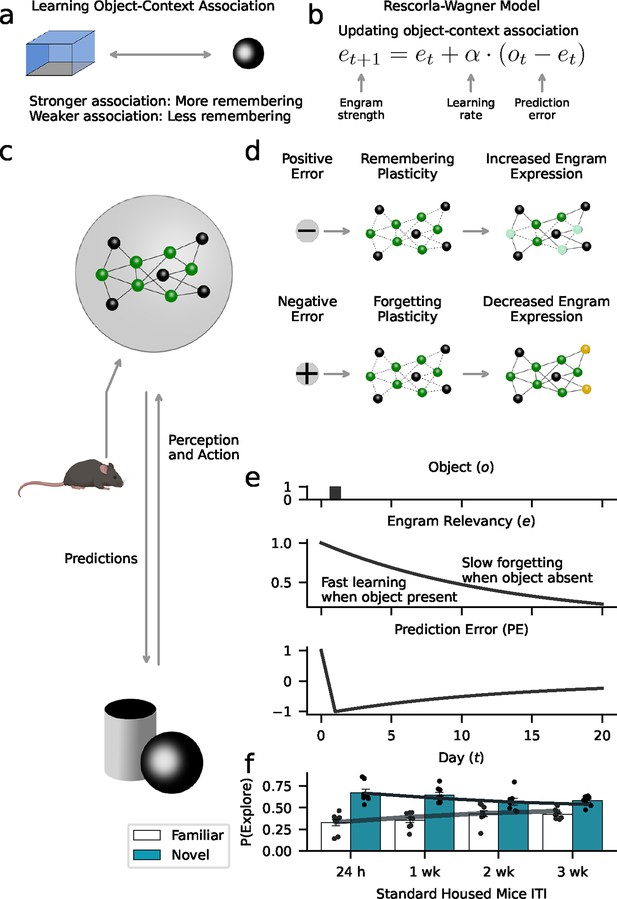
Forgetting as adaptive learning.
Our model assumes that animals create and update memory engrams to flexibly adjust their behavior to their environment. (a) We used a Rescorla-Wagner model that learns object-context associations in the object-based memory task. The key assumption is that stronger associations lead to higher memory performance, while weaker associations lead to more forgetting. (b) The Rescorla-Wagner model updated the strength of object-context associations (‘engram strength’) as a function of the prediction error (difference between experienced object and engram strength) and the learning rates governing the influence of the prediction error. (c) Based on learned representations, animals constantly predict what happens in the environment (e.g. the occurrence of objects), and if predictions are violated (prediction errors), engrams are updated to improve the accuracy of future predictions. Here, established engram cells are shown in green; non-engram cells in gray. (d) Positive prediction errors signaling the occurrence of an unexpected event (e.g. new object) induce a learning process that increases the probability of remembering. This might rely on the recruitment of new engram cells (shown in yellow). In contrast, negative prediction errors signaling the absence of an expected event (e.g. predicted object did not appear) induce forgetting. This might rely on ‘forgetting’ plasticity reducing access to engrams (light green cells). (e) Our model formalizes this perspective based on the notion of ‘engram relevancy’, i.e., the strength of the object-context association. Higher engram relevancy makes it more likely that an engram is behaviorally expressed, e.g., through exploration behavior. The presentation of a novel object (upper panel) leads to a high engram relevancy (middle panel) in response to a positive prediction error (lower panel). The absence of an expected object decreases engram relevancy through negative prediction errors. (f) Model simulations corroborate the behavioral effects of our data (Figure 3a). Gray lines and bars show the average exploration probability for the familiar and novel object according to the model; markers show simulated mice.
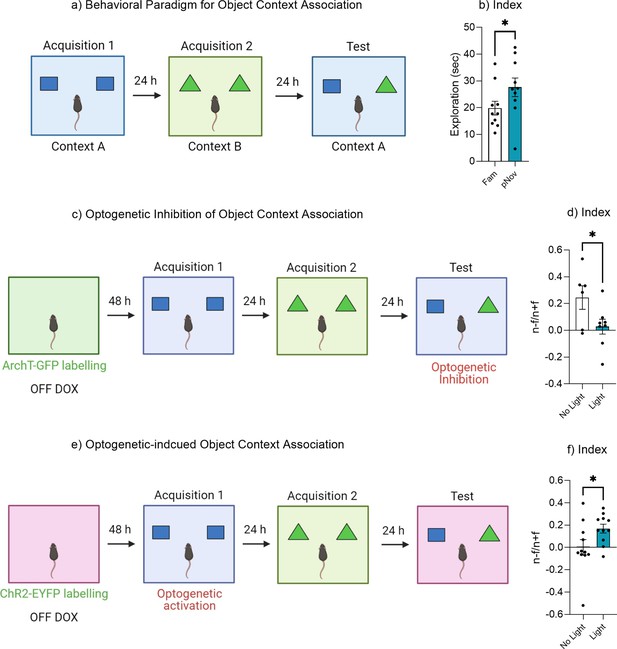
Dentate gyrus engram modulates object context associations.
(a) Behavioral paradigm for object-context associations. (b) Discrimination index. (c) Experimental timeline for optogenetic inhibition of object-context association. (d) Discrimination index. (e) Experimental timeline for optogenetic-induced object-context association. (f) Discrimination index. Bar graphs indicate average values in n = 6–11 per group (*p<0.05). Data graphed as means ± SEM. Panel a was created with BioRender.com. Panel c was created with BioRender.com. Panel e was created with BioRender.com.
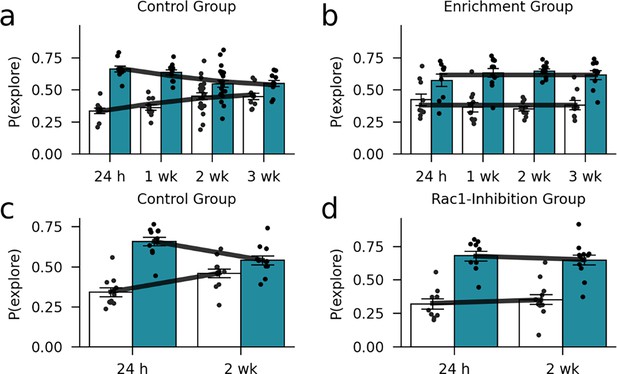
In-sample model validation.
A comparison between the forgetting data and the forgetting curve predicted by the model suggests that the model captures the data accurately. (a) Control group (standard housing condition) of the environmental enrichment experiment. (b) Enrichment group of the environmental enrichment experiment. (c) Control group of the Rac1-inhibition experiment. (d) Rac1-inhibition group of the Rac1-inhibition experiment. Markers and bars show exploration probabilities of the mice. Lines show the average exploration probability for the familiar and novel object according to the model.
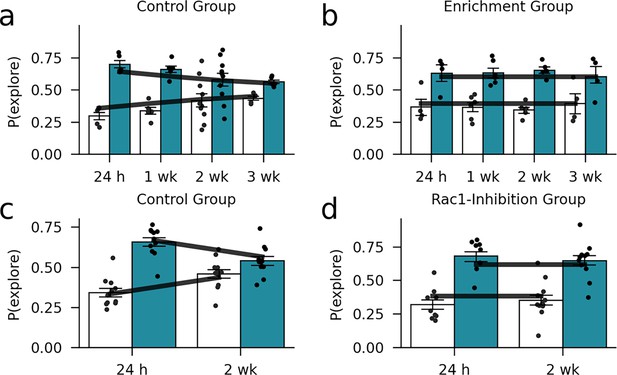
Out-of-sample model validation.
We examined the model fit using a simple cross-validation procedure. For the environmental enrichment data set, we estimated the free parameters based on half of the subjects. Then, we compared the predicted exploration behavior (dark lines) to the data of the other half of the subjects. (a) Control group. (b) Enrichment group (markers and bars show exploration probabilities of the mice). Furthermore, we compared the predicted exploration probabilities conditional on the parameter estimates from the environmental enrichment experiment to the observed exploration probabilities in the Rac1-inhibition experiment. (c) Control group. (d) Rac1-inhibition group.
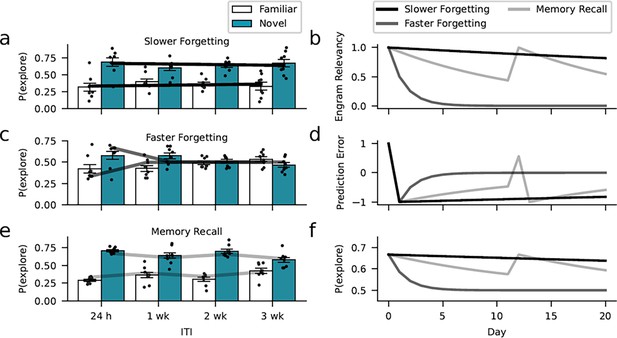
Learning model captures the dynamics of forgetting.
Environmental, optogenetic, and pharmacological manipulations might modulate the speed of forgetting by altering key parameters of our model. Simulations with different learning-rate parameters explain the forgetting dynamics of the different experimental conditions. (a) The enrichment and Rac1-inhibition conditions were successfully captured using a low learning rate (0.01, similar to the empirical estimates). (c) In contrast, assuming a larger learning rate (0.5), we could capture faster forgetting as observed in the Rac1 activator and context-only conditions. (e) Moreover, improved memory performance after reminder cues can be explained by assuming that these interventions induce a positive prediction error boosting object relevancy. Here, we assumed a learning rate of 0.07 (based on the empirical estimate). (b) Development of engram relevancy and (d) prediction errors across conditions. (f) Probability of exploring the novel object plotted separately for each condition.
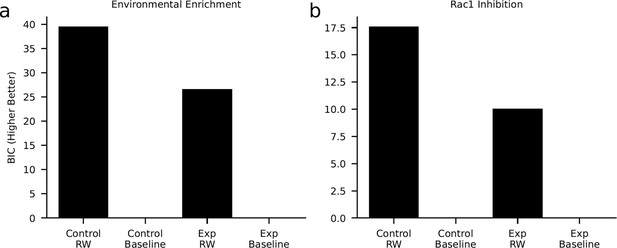
Model comparison.
We tested if our computational model (Rescorla-Wagner [‘RW’]) describes the data better than a control model (‘Baseline’) that explores each object with a probability of 0.5. The model comparison was based on a comparison of the cumulated Bayesian information criterion (BIC). Here, higher values indicate a better model fit. In both the environmental enrichment experiment (a, experimental and control condition) and the Rac1-inhibition experiment (b, experimental and control condition), the model comparison favored the RW-learning model. These results show that our model explained the data above chance level.
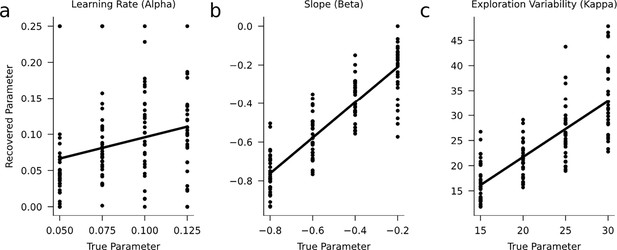
Parameter recovery.
We performed a parameter-recovery study to examine whether the free parameters of our learning model could be estimated accurately. Similar to the enrichment experiment, we assumed n=12 mice per inter-test interval (24 hr, 1 week, 2 weeks, 3 weeks). The recovery study indicates that the (a) learning-rate, (b) slope, and (c) exploration-variability parameters can sufficiently be estimated given the limited amount of data for model fitting. We validated that the variability of the parameters, especially of the learning rate, is lower when more subjects are included (e.g. n=50; not shown).
Additional files
-
Supplementary file 1
Parameter estimates.
Control group (Cont) and experimental group (Exp).
- https://cdn.elifesciences.org/articles/92860/elife-92860-supp1-v1.docx
-
MDAR checklist
- https://cdn.elifesciences.org/articles/92860/elife-92860-mdarchecklist1-v1.docx