High-throughput expansion microscopy enables scalable super-resolution imaging
Figures
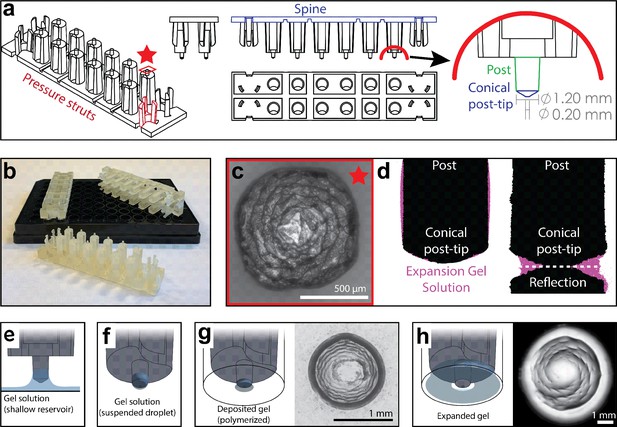
High-throughput expansion microscopy (HiExM) enables gel formation and expansion in a 96-well cell culture plate.
(a) Schematic representation of HiExM devices showing the key features highlighted in color. (b) Example devices used in 96-well cell culture plates. (c) Brightfield image of the conical post-tip shows the pattern of grooves that mediate fluid retention. (d) Fluid retention at the conical post-tip of the device. Silhouettes taken by an optical comparator of the profile of a single post suspended above a surface (left) and in contact with a surface (right) show a fluid droplet interacting with the device. Upon device insertion, the gel solution fills the space under the conical post tip, forming the toroid gel. (e–h) Schematic of HiExM gel deposition and expansion workflow. (e) The device is immersed in a shallow reservoir of gel solution. (f) Upon removal, the tip of each device post retains a small volume of gel solution. (g) Gel solution is deposited by the device into the well centers of the cell culture plate. Brightfield image (right) shows gel geometry and size prior to expansion. Note that gels deposited in HiExM cover ~1.1 mm2 of the well surface to accommodate the expanded gel, and do not include cells outside the gel footprint. (h) Polymerization and expansion are performed with the device in place. Brightfield image (right) shows gel geometry and size after expansion.
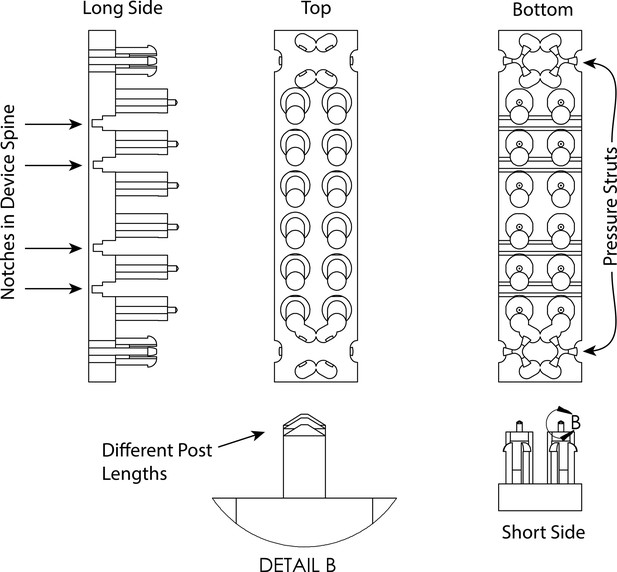
Schematic depiction of high-throughput expansion microscopy (HiExM) device with displayed features.
The device was designed with three different post lengths where the four center posts are the longest, the two pairs of posts on the ends near the pressure struts are the shortest, and the middle posts are an intermediate length. When the device is inserted into the well plate, the center four posts contact the culture surface first. As downward pressure is applied to the ends of the device (by the user pressing down above the pressure struts), the spine of the device bends at the notches such that all posts contact the plate. These design features allow for the reproducible deposition of gel solution droplets and the reproducible formation of toroidal gels within the well constraints.
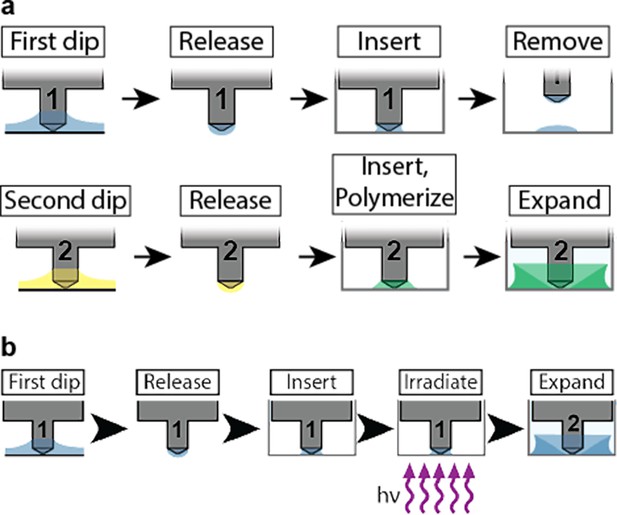
Overall schematic of high-throughput expansion microscopy (HiExM) comparing standard chemistry and photoinitiation.
(A) For standard expansion microscopy (ExM), the two initiating components (ammonium persulfate, APS and tetramethylethylenediamine, TEMED) were added to cells in wells in a two-step process to control the polymerization rate. In the first step, a droplet of TEMED-containing gel solution is deposited in the well and the device is removed. In the second step, a second identical device delivers a droplet of APS-containing gel solution to the same well with the TEMED-containing droplet to initiate polymerization. The well plate is also kept on ice for 15 min while the APS and TEMED are mixed, followed by heating the well plate at 50 °C for 5 min to initiate rapid polymerization. Additionally, the protocol is performed in a glove bag purged twice with nitrogen. (B) The use of photoinitiation HiExM as described in Methods allows for one-step polymerization. The Irgacure 2959 gel solution is deposited and polymerization is initiated by irradiation with a UVA metal halide lamp.
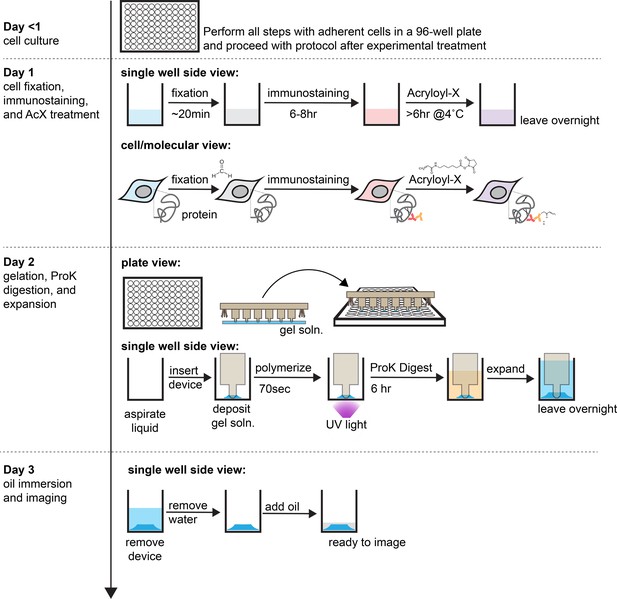
Detailed workflow of the high-throughput expansion microscopy (HiExM) protocol using photoinitiation.
HiExM can be used with cells cultured in commercially available 96-well plates. After conducting an experiment, cells can be fixed and stained as normal, followed by the addition of the anchor molecule Acryloyl-X. The following day, Acyloyl-X is aspirated, washed once with PBS and once with DI water, and then aspirated again to leave cells dry. It is important to ensure that no residual liquid remains in the well. Following aspiration, the device is used to deposit gel droplets on cells. Deposited gels are left for ~90 s followed by a 70- s exposure to UV light to initiate polymerization. Proteinase K solution is then pipetted into the wells and after 6 hr, the plate is submerged in ~4 L of water in a beaker and left suspended over a stir bar overnight. Water is then carefully aspirated from the wells and a layer of mineral oil is applied to each well to prevent evaporation and to stabilize the gel position for imaging. All steps in this procedure are performed at room temperature.
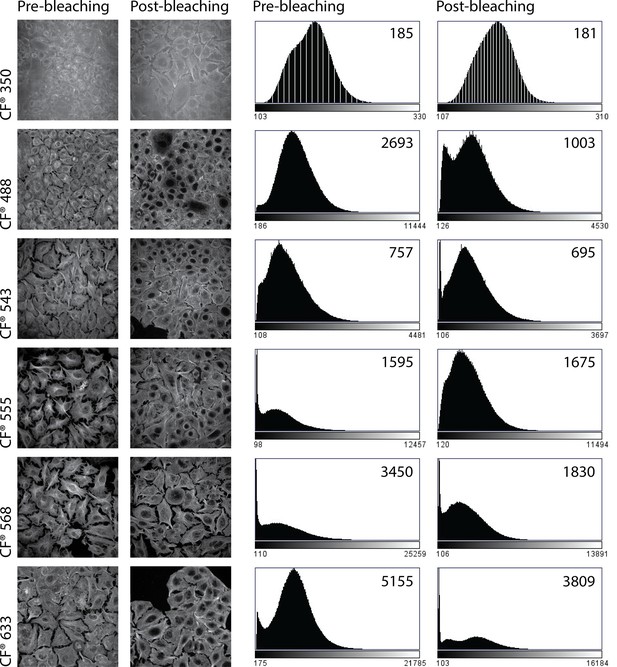
CF conjugated antibodies yield robust signal and resistance to photobleaching in Irgacure high-throughput expansion Microscopy microscopy (HiExM).
Representative images of A549 cells stained with α-Tubulin antibodies and secondary antibodies conjugated to CF dyes at different wavelengths before (left) and after (right) exposure to UV light as described in the HiExM protocol. Stained cells were exposed to UV light in the presence of Irgacure 2959 in 0.1% PBS. Histograms representing the raw pixel values from these images are shown on the right, where the number on each plot represents the peak pixel value for that image. Decreased peak pixel values indicate a loss of signal due to photobleaching.
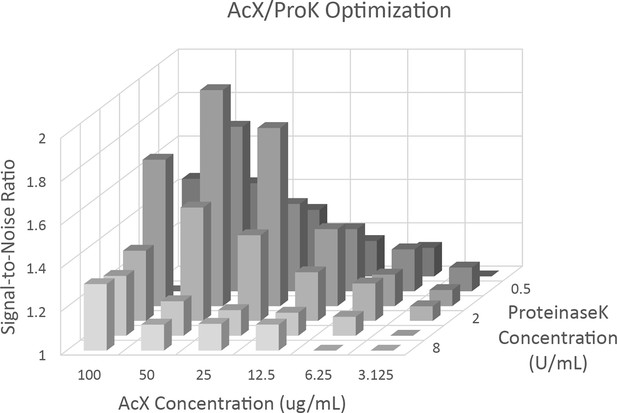
Acryloyl-X (AcX) and ProteinaseK titration.
Signal-to-noise ratio was determined by measuring the average fluorescence intensity in an area of the cell and an area of the background for α-tubulin stained A549 cells from images obtained using the Opera Phenix. Optimum signal-to-noise was found at concentrations of 50 µg/mL AcX and 1 U/mL ProteinaseK.
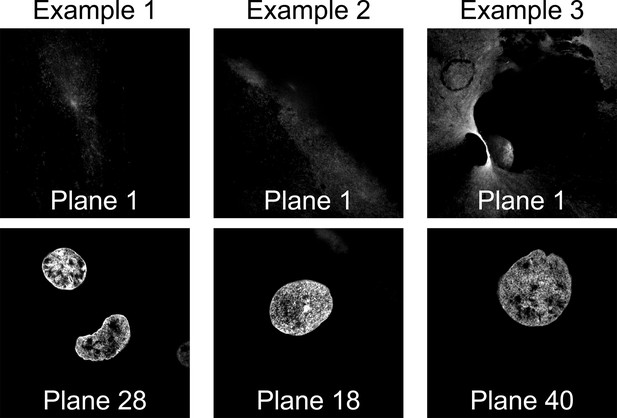
Residual hoechst signal occasionally remains underneath high-throughput expansion microscopy (HiExM) samples.
The lowest image planes (top row) of three example images that show residual hoechst signal. Higher planes from the same images (bottom row) show nuclei within the sample.
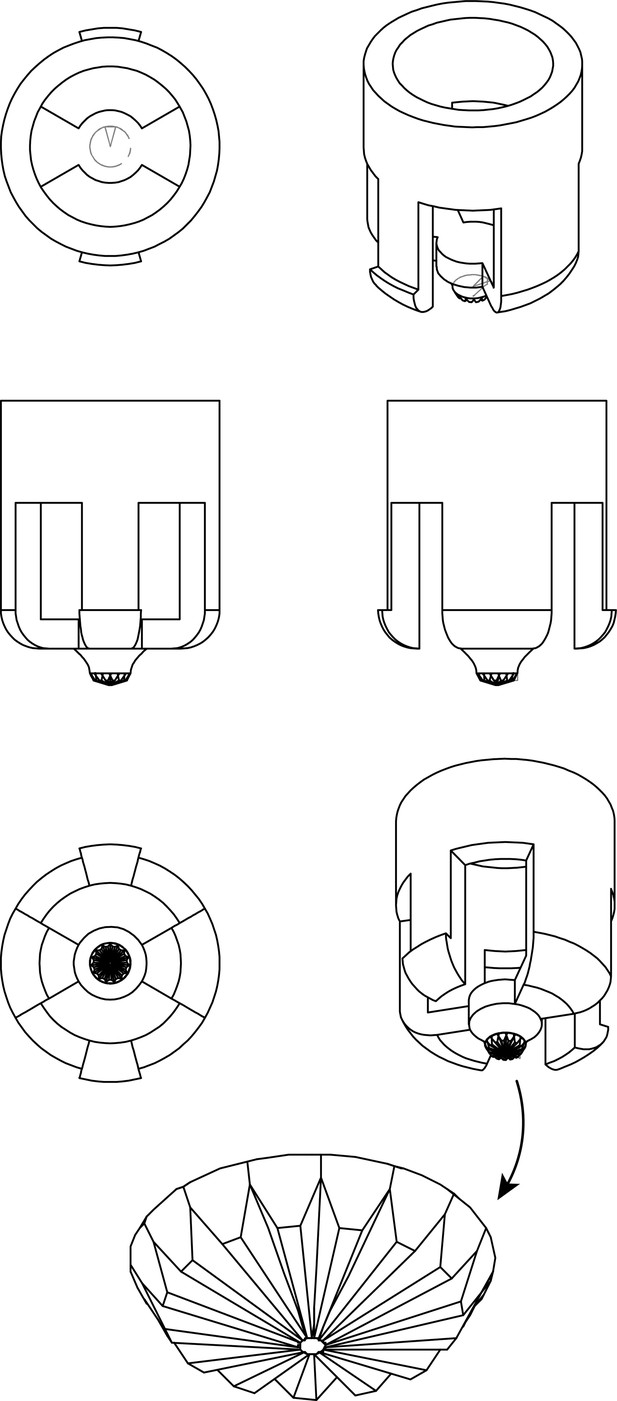
Schematic depiction of the 24-well plate high-throughput expansion microscopy (HiExM) device.
This device was designed to form one gel in a well of a standard 24-well cell culture plate and manufactured with injection molding (Protolabs).
Example of expansion process in high-throughput expansion microscopy (HiExM) with ammonium persulfate (APS)/tetramethylethylenediamine(TEMED) chemistry.
Tubulin-stained A549s were monitored after gel formation with digestion buffer on. This video, taken in real time, shows how cells treated with this protocol tend to ‘stick’ to the cell culture substrate as the gel expands. Cells are torn off the culture substrate by the expanding gel, often leaving behind residual cellular material stuck to the culture substrate.
Example of expansion process in high-throughput expansion microscopy (HiExM) with Irgacure chemistry.
Tubulin-stained A549s were monitored after gel formation with digestion buffer on. This video, taken at 10X speed, shows cells expanding normally in HiExM in the presence of the digestion buffer. The post of the device is close to the bottom-left of the image.
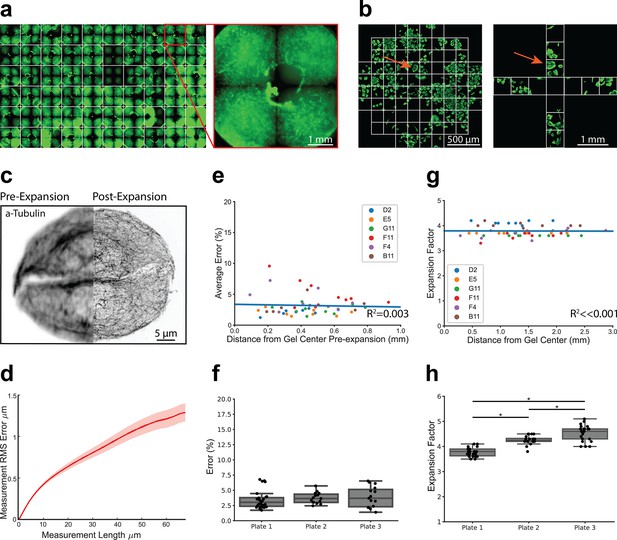
High-throughput expansion microscopy (HiExM) is compatible with nanoscale expansion and automated image acquisition of human cells with minimal distortion.
(a) Expanded gels in a 96-well plate imaged at 5 x with an inset showing an individual gel shown on the right. (b) Imaging fields are shown for pre-expansion and post-expansion samples. Prior to expansion, 61 fields were imaged at 63 x around the center of the gel. After expansion, 12 fields were imaged at 20 x magnification. Max projections for each field were then stitched together and manually inspected to identify fields in the pre-expansion and post-expansion image sets containing matching cells. Arrows show an example of a field representing the same cells before and after expansion. (c) Representative registered fluorescence image of microtubules before (left) and after (right) expansion showing the increase in resolution conferred by expansion. In this case, both pre-expansion and post-expansion images were taken at 63 x magnification. (d) Representative error curve calculated using non-rigid registration of 43 independent fields of view. The shaded region denotes one standard error of the mean. (e) Error is not dependent on the location of a given field of view within the gel. Each data point represents the average percent error (up to 40 μm measurement length post-expansion) for a given field of view as determined by an non-rigid registration (NRR) analysis. Seven to ten fields of view were analyzed across six wells. The distance from the center of each field of view to the center of the well was measured manually in FIJI using the stitched pre-expansion image set. (f) Box plots showing the distribution of average error among gels across three plates (average = 3.63% +/- 1.39). Average error percentages for each well plate: 3.73 +/- 0.879, 3.89 +/- 1.65, and 3.43 +/- 1.44. (g) Expansion factor is not dependent on the location of a given field of view within the gel. Images taken before and after the expansion of the same cell or group of cells were used to measure the ratio of length scales in the two images. (h) Box plots showing the distribution of expansion factors of 58 gels across three plates. Expansion factors ranged from 3.5 to 5.1 x across gels with an average of 4.16 +/- 0.394. Average expansion factors for each well plate showed tight distribution with some variability across plates: 3.78 +/- 0.171, 4.26 +/- 0.171, and 4.53 +/- 0.321. Comparison of the three well plates was performed using one-way ANOVA and post-hoc Tukey HSD test (*p-value<0.05).
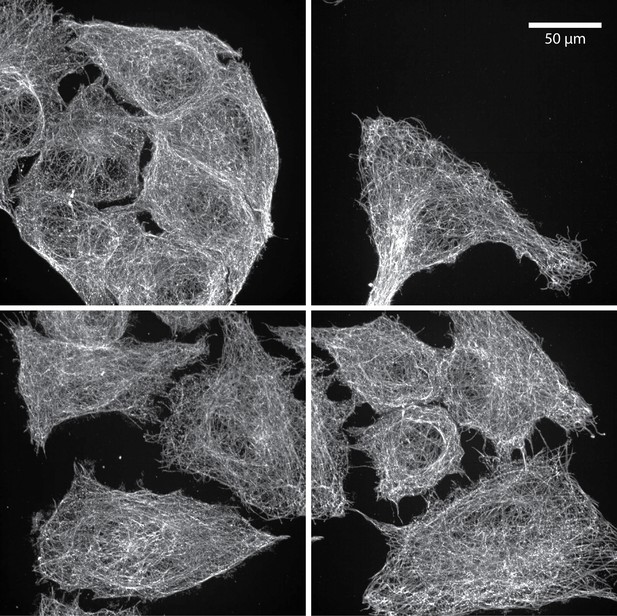
Representative images post-expansion showing nanoscale resolution in high-throughput expansion microscopy (HiExM).
A549 cells were stained for α-Tubulin and imaged on an Opera Phenix High Content Screening System (PerkinElmer) using a 63 x objective (1.15 na) as described in Figure 2. Scale bar represents the real scale of the images, not corrected for expansion.
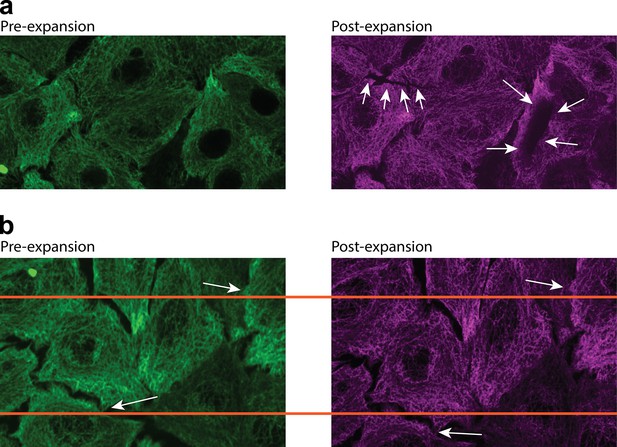
Infrequent higher expansion error in high-throughput expansion microscopy (HiExM) is due to abnormal stretching and tearing of the gel.
(A) Arrows in the image on the right point to occasional artifacts arising during the expansion process. These rare artifacts generally occur closer to the center of the gel. (B) Arrows point to matched features in the pre-expansion and post-expansion images. These images were aligned using TurboReg as previously described (Gustafsson, 2000). A comparison of the two images shows that the expanded sample was stretched anisotropically over length scales on the order of the size of the cell.
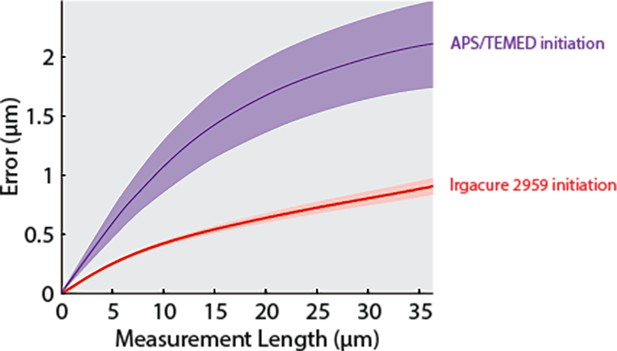
Comparison of expansion error measurements between standard expansion microscopy (ExM) chemistry and Irgacure 2959 photoinitiation.
Consensus error curves were generated as described in Figure 2 for ammonium persulfate (APS)/tetramethylethylenediamine (TEMED) gels (left) as compared to Irgacure 2959 gels (right). These data demonstrate that photoinitiation results in robust expansion and less error compared to the APS/TEMED chemistry in our high-throughput expansion microscopy (HiExM) method.
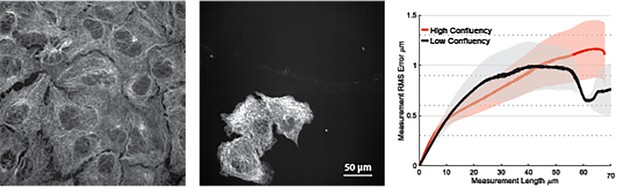
High-throughput expansion microscopy (HiExM) performs robustly on cells plated at low and high confluency.
Example images of high (left) and low (center) confluent cultures of A549s. Consensus error curves (right) were calculated as described in Figure 2, and show minimal error within ~0.2 um (n=4 fields of view for each condition).
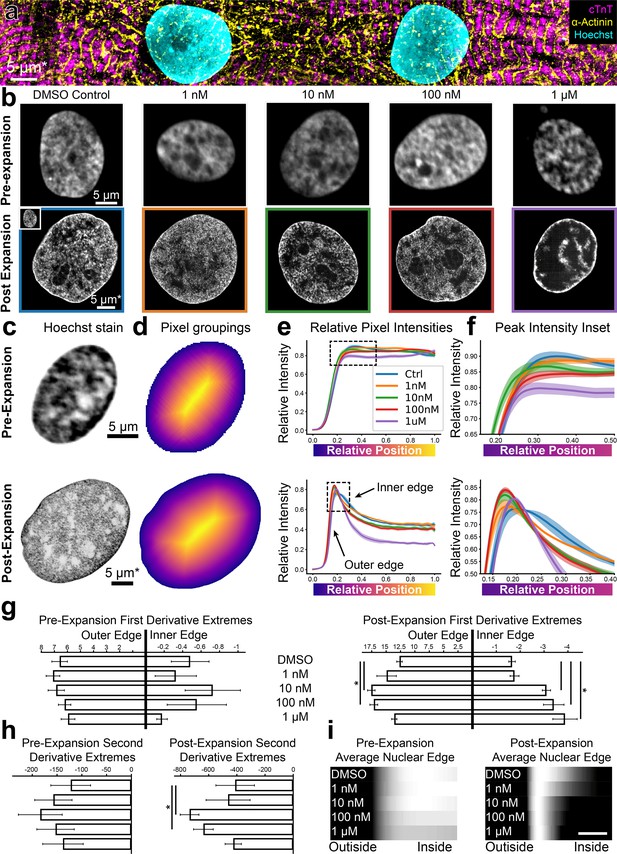
High-throughput expansion microscopy (HiExM) shows altered nuclear morphology of cardiomyocytes treated with low doses of doxorubicin.
(a) hiPSC-CMs imaged post-expansion in HiExM. (b) Example images of human induced pluripotent stem cell derived cardiomyocyte (hiPSC-CM) nuclei after dox treatment for 24 hr before (top) and after (bottom) expansion. (c) Example images of hiPSC-CM nuclei taken before (top) and after (bottom) expansion. (d) Heatmaps of nuclei in (c) showing pixel groupings for subsequent analysis. Heatmaps were generated from the image mask after dilation, such that the outer edge represents a contour just beyond the nuclear periphery. (e) Relative Hoechst intensity plotted as a function of pixel position relative to the edge of the dilated mask in pre- (top) and post- (bottom) expansion nuclei. Colored curves represent dox concentrations or DMSO (ctrl). Shaded regions represent SEM for n=56, 71, 64, 92, and 62 nuclei in the pre-expansion images and n=4 replicates in the expanded case (118, 111, 110, 113, and 77 nuclei analyzed for DMSO control, 1 nM, 10 nM, 100 nM, and 1 µM Dox, respectively). (f) Insets of plots in (e) highlighting the nuclear periphery. (g) Rate-of-change analysis of curves for pre- (left) and post- (right) expansion images. Outer edge values were obtained by determining the maximum values of the derivatives for each condition, and inner edge values were obtained by determining the minimum values of the derivatives within the domain 0–0.4. (h) Curvature analysis for pre- (left) and post- (right) expansion data. Values represent the minima of the second derivative for curves within the domain 0–0.4. (i) Average Hoechst intensities are plotted as gradients for each dox concentration. Scale bar represents a 10 percent distance from the edge of the nucleus to the center. Error bars represent SEM as in (e). * denotes significance from an independent two-sample t-test (p<0.05). *Scale bar represents biological scale assuming 4 x expansion.
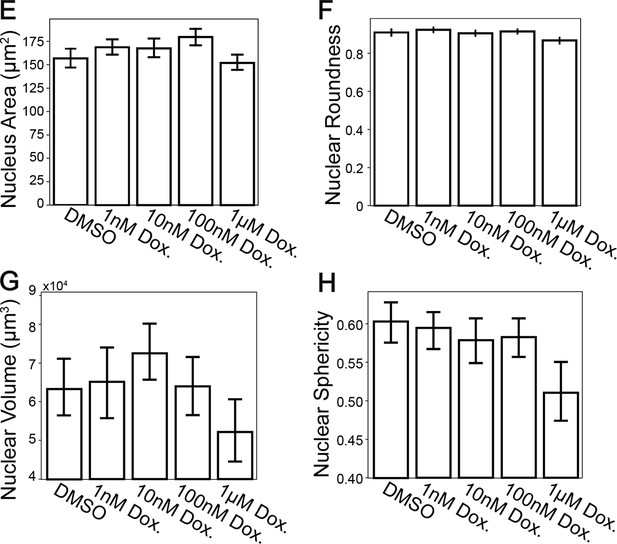
Nuclear volume/area analysis of Doxorubicin-treated cardiomyocytes before and after expansion.
Nuclear area (top left) and roundness (top right) were measured in pre-expanded cardiomyocytes. Nuclear volume (bottom left) and sphericity (bottom right) were measurable in expanded cardiomyocytes due to the increased axial resolution of high-throughput expansion microscopy (HiExM). These measurements were performed in Harmony (Perkin Elmer). Error bars represent SEM and sample sizes are the same as in Figure 3 for both unexpanded and expanded cells. * denotes significance from an ANOVA (*p<0.05, **p<0.005, ***p<0.0005).
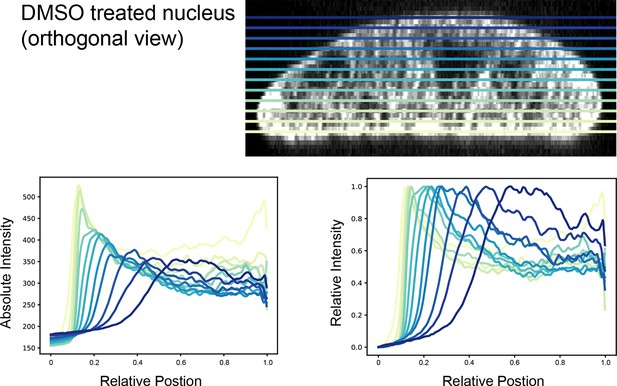
Nuclear edge analysis at different optical slices of Doxorubicin-treated cardiomyocytes.
Defining the optical plane that intersects the nucleus at a right angle in the z-axis is necessary for nuclear edge analysis. Orthogonal view of a DMSO-treated nucleus. Colored lines represent optical slices corresponding to the analysis results below the image (top). Absolute signal intensity as a function of relative position at 12 different z-slices using the ‘ring-scan’ analysis (bottom left). Relative signal intensity as a function of relative position at the same 12 z-slices using the same analysis (bottom right).
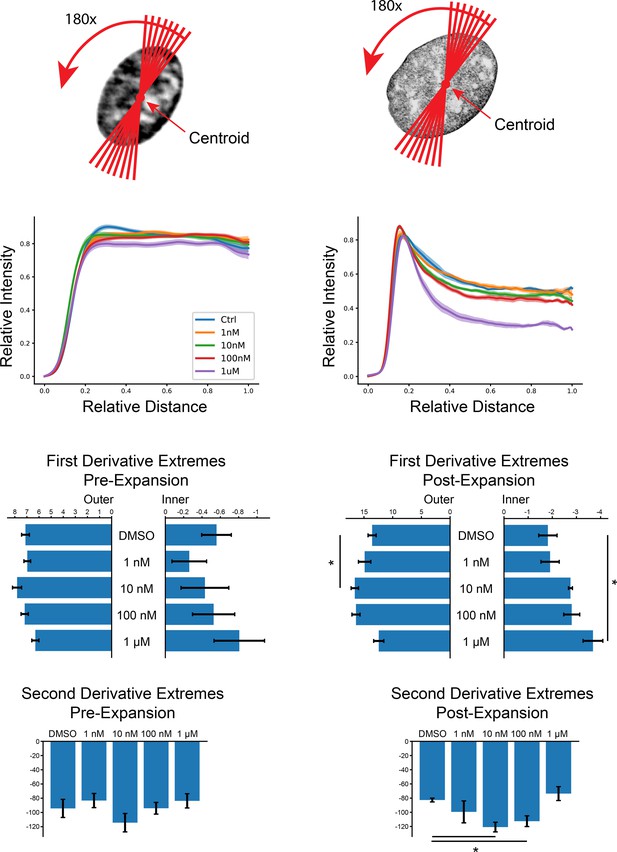
Line-scan analysis of Doxorubicin-treated cardiomyocytes.
An orthogonal line-scan analysis shows similar results in Figure 3. Briefly, the centroid of the nucleus was found and 180 lines were drawn through the image at one-degree increments. The pixel intensities from these scans were then interpolated (linear) to normalize their lengths to 500. The scans were then cut in half, the second half of the scans were mirrored, and the two halves were averaged to give a representation of Hoechst intensity as a function of distance from the center of the nucleus. Shaded region represents SEM as in Figure 3e. First and second derivatives were calculated as in Figure 3g and h. Error bars represent SEM, asterisks denote significance from an independent two-sample t-test (p<0.05).
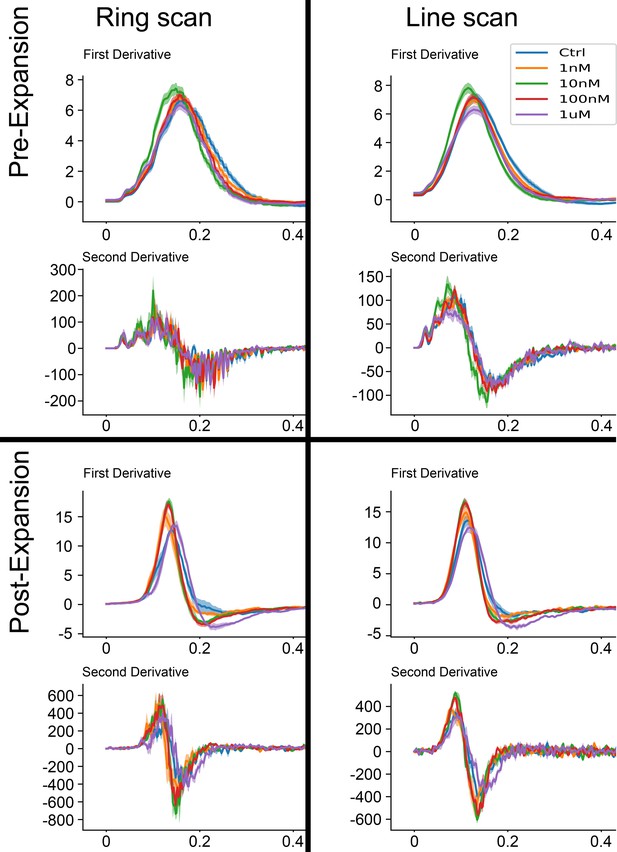
Comparison of derivative curves from Figure 3 and Figure 3—figure supplement 3.
Curves on the left are described in Figure 3 (ring scan), and on the right are described in Figure 3—figure supplement 3 (line-scan). Top eight curves represent pre-expansion, bottom eight curves represent post-expansion analyses.
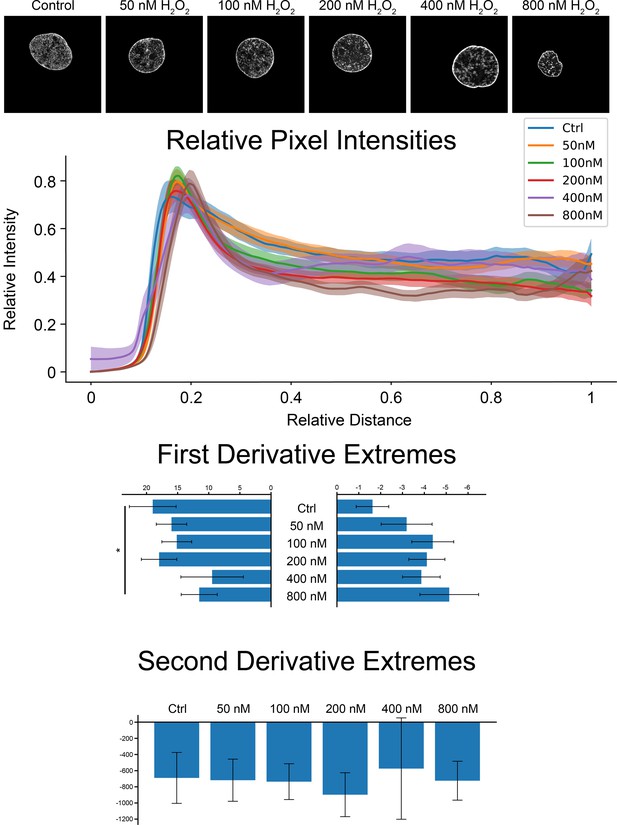
Nuclear edge analysis of H2O2-treated cardiomyocytes.
Example images of cardiomyocyte nuclei across different conditions and associated curves (top). Analyses were carried out as in Figure 3. First derivative inflection-point-slope extremes (middle) show a significant reduction at the outer side only between the control and the highest concentration of hydrogen peroxide. Second derivative minima (bottom) show no significant differences between conditions.
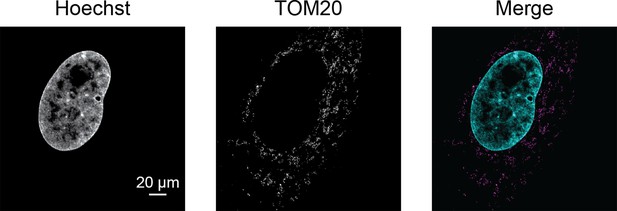
Example of a cell expanded in high-throughput expansion microscopy (HiExM) using Photo-expansion microscopy (ExM) gel chemistry.
Photo-ExM does not require an anoxic environment for gel deposition and polymerization, improving the ease of use of HiExM. Mitochondria were stained with an Alexa 647 conjugated secondary antibody, demonstrating that HiExM is compatible with additional fluorophores when combined with Photo-ExM.
Animation of nuclear periphery analysis employed in Figure 3.
This animation depicts an analysis of an example nucleus, where each frame is a single iteration of the analysis. The red ring overlay indicates the current group of pixels being averaged. The mean intensity of these pixels is plotted on the y-axis in the graph on the right, with the x-axis representing the progression of iterations from the outer edge of the nucleus to its center.