Attentional modulation of secondary somatosensory and visual thalamus of mice
Figures
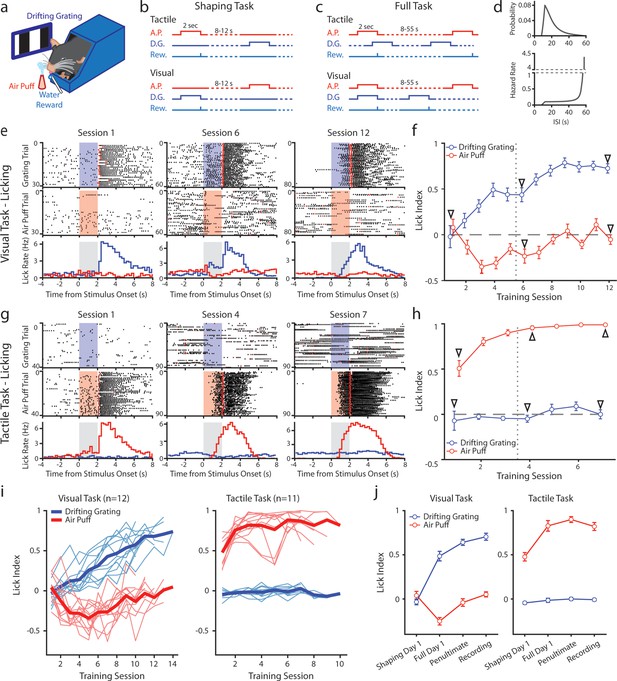
Mice can be conditioned to attend to one sensory modality while ignoring a second modality.
(a) Behavioral setup. Mice were head-fixed and presented with a visual stimulus (a drifting grating on a screen) or a tactile stimulus (an air puff to the distal portion of the whiskers). (b) Schematic for the shaping version of the task, depicting the timing of the air puff (red), the drifting grating (dark blue), and the water reward (light blue). Mice were presented with either stimulus with an inter-stimulus interval of 8–12 s. In the tactile cohort, the air puff was paired with a water reward; in the visual cohort, the drifting grating was paired with the water reward. (c) Schematic for the full version of the task. Stimuli were allowed to overlap so that only the conditioned stimulus predicted the timing of the water reward. (d) Inter-stimulus intervals for each modality in the full task were drawn from an exponential distribution with a linear offset (top). As a result, the hazard rate was largely flat for most stimulus presentations (bottom). (e) Example behavior from a mouse trained on the visual task. Top row: raster plot of licks aligned to the onset of the grating (blue region). Red markers indicate the first lick to occur after a reward was available. Middle row: raster plot of licks aligned to the onset of the air puff (red region). Bottom row: Mean lick rate aligned to the onset of the drifting grating (blue) or the air puff (red). Gray region indicates the stimulation period. Data are from the first session of the shaping task (left column), the first day of the full task (middle column), and the final day of conditioning (right column). (f) Learning curve of the mouse shown in e, showing the lick index (mean ± SEM) for each stimulus on each training day. Vertical dotted line indicates the day the mouse was switched from the shaping task to the full task. Triangles: sessions shown in e. (g, h) Similar to e and f but for an example mouse trained on the tactile task. (i) Learning curves of all visually conditioned mice (left) and tactilely conditioned mice (right). Thin lines: individual mice. Thick lines: mean across all mice. (j) Mean and SEM lick index for all mice on the first day of conditioning (‘shaping day 1’), the first day of the full task (‘full day 1’), the day before neural recordings (‘penultimate’), and the day of recording (‘recording’). The penultimate day and recording day did not significantly differ in lick index for the conditioned stimulus in either conditioning group (visual conditioning p=0.18, n=12 mice, Wilcoxon signed-rank test; tactile conditioning p=0.08, n=11 mice, Wilcoxon signed-rank test).
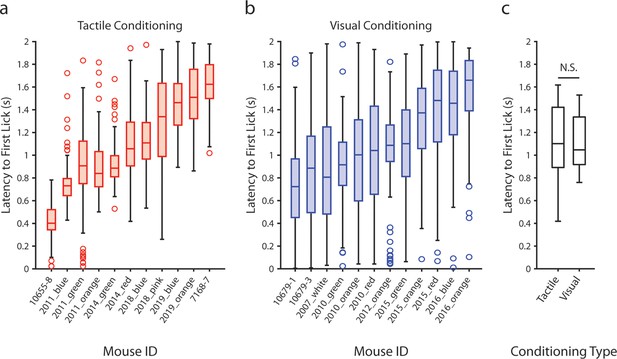
Latency of anticipatory licking does not depend on the modality of the conditioned stimulus.
(a) Latency to the first lick that occurred after the presentation of the air puff in tactilely conditioned mice on the day of recording. Box plots depict the distribution of lick latencies of individual mice for all presentations of the air puff. Only licks that occurred prior to the delivery of the water reward (t ≤ 2 s) were counted. (b) Latency to the first lick after the presentation of the drifting grating in visually conditioned mice on the day of recording. (c) Box plots of the mean lick latency for each mouse as a function of experiment type. Visually and tactilely conditioned mice did not have significantly different lick latencies (tactile conditioning median latency = 1.1 s, n=11 mice; visual conditioning median latency = 1.0 s, n=12 mice; p=0.98, Wilcoxon rank sum test).
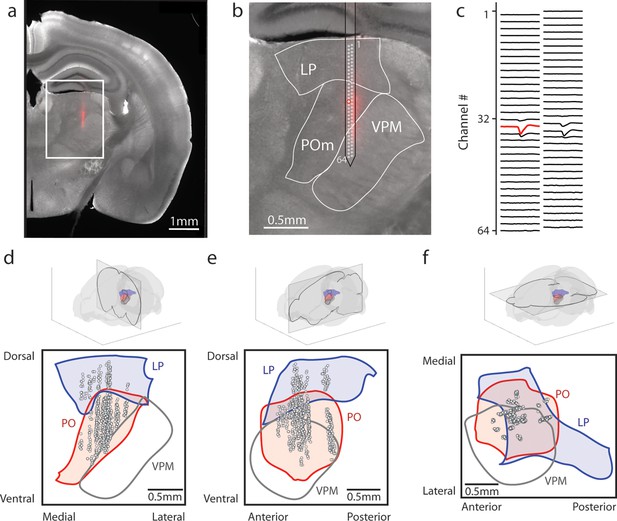
Array recordings were made simultaneously from posterior medial nucleus (POm) and lateral posterior nucleus (LP).
(a) Example coronal section. Red: DiI marking recording track. Gray: endogenous biotin stained with streptavidin Alexa fluor 647. (b) Zoom of the outlined region in a. The borders of LP, POm, and ventral posterior medial nucleus (VPM) are outlined. A 64-channel silicon probe was used to record from LP and POm. (c) Mean waveform of an example POm cell across all probe channels. The channel with the largest mean waveform was identified and used to estimate the position of the cell (red circle in B). (d, e, f) Estimated location of putative cells (gray circles) located in POm (red) and LP (blue) aligned to the Allen reference atlas. Cell locations are jittered along the medial-lateral and anterior-posterior axes for visualization purposes. (d) Coronal projection over the range of cell locations in the anterior-posterior axis. (e) Sagittal projection over the range of cell locations in the medial-lateral axis. (f) Transverse projection over the range of cell locations in the dorsal-ventral axis.
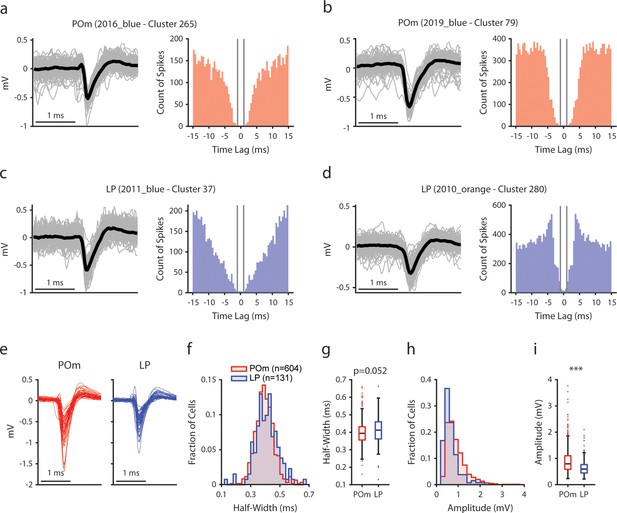
Manual curation of posterior medial nucleus (POm) and lateral posterior nucleus (LP) cells.
(a, b) Example POm cells. Left, Mean spike waveform (black) with 200 randomly sampled individual waveforms (gray). Right, Autocorrelogram, binned at 0.5 ms. Gray lines indicate a time lag of ± 1 ms. (c, d) Example LP cells, as in a and b. (e) Mean waveforms of 50 randomly selected POm cells (red) and 50 randomly selected LP cells (blue). (f) Histogram of waveform half-widths of each recorded POm cell (red, n=604) and LP cell (blue, n=131). (g) Box chart of waveform half-width. Half-widths were not significantly different between LP and POm cells (POm: 0.40 ± 0.07 ms, LP: 0.41 ± 0.08 ms (mean ± std), t-test p=0.052). (h) Histogram of waveform amplitudes from each recorded POm and LP cell. (i) Box chart of waveform amplitudes. POm cells had significantly higher amplitudes than LP cells (POm: 0.91 ± 0.47 mV, LP: 0.67 ± 0.33 mV (mean ± std), t-test p<0.001).
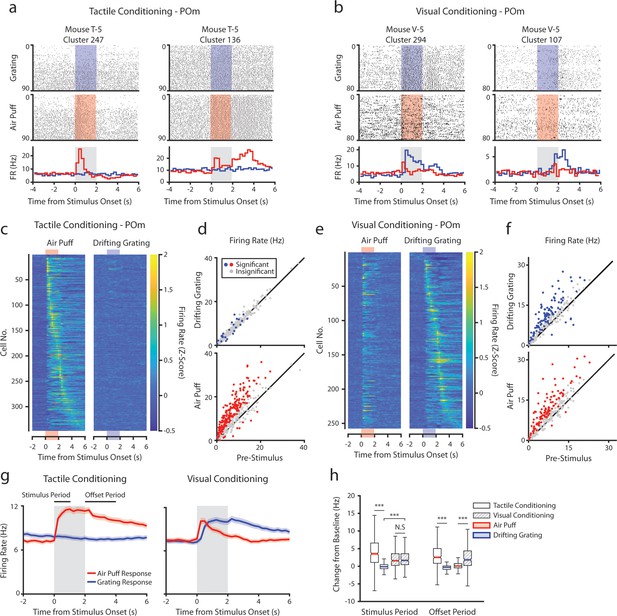
Posterior medial nucleus (POm) responses to tactile and visual stimuli depend on the conditioning paradigm.
(a) Example cells from a tactilely conditioned mouse (same mouse as in Figure 1g, session 7). Top row: spike raster aligned to the onset of the drifting grating (blue). Middle row: spike raster aligned to the air puff (red region). Bottom row: Mean firing rate over each trial aligned to the drifting grating (blue line) and the air puff (red line). Gray region indicates the timing of both stimuli. (b) Sample cells from a visually conditioned mouse (same mouse as in Figure 1e, session 12). (c) Firing rate of all POm cells from tactilely conditioned mice aligned to either the air puff (left) or the drifting grating (right). Firing rates are binned at 250 ms and cells are sorted by the timing of the peak firing rate when aligned to the air puff. n=347 cells, 11 mice, range = 11–57 cells/mouse, median = 33 cells/mouse. (d) Scatter plot of POm firing rates 1 s pre-stimulus (x-axis) and 1 s during stimulus onset (y-axis) from tactilely conditioned mice. Colored markers: cells with significantly different firing rates pre- and post-stimulus (1-way ANOVA with Holm-Bonferroni correction and post-hoc Wilcoxon signed-rank test). Gray markers: cells without significantly different firing rates. (e) Firing rates of all POm cells from visually conditioned mice aligned to either the air puff (left) or the drifting grating (right). Cells are sorted by the timing of the peak firing rate aligned to the grating. (n=257 cells, 11 mice, range=10–42 cells/mouse, median=19 cells/mouse). (f) Scatter plot of POm firing rates pre- and post-stimulus onset for visually conditioned mice, as in e. (g) Event-triggered average firing rates of all POm cells (mean ± SEM) aligned to either the air puff (red) or the drifting grating (blue) in tactilely conditioned mice (left) and visually conditioned mice (right). Gray region indicates the timing of both stimuli. Rates binned at 250 ms. (h) Box plots of the change in firing rate from baseline for each POm cell during the first second of stimulus onset (‘stimulus period’) and during the first 2 s post-stimulus (‘offset period’). In tactilely conditioned mice, the change from baseline was greater in the air puff stimulus period than the visual stimulus period (two-way ANOVA with post-hoc signed-rank test; conditioning type F=1.98, p=0.16; stimulus type F=153, p<10–33; interaction F=115, p<10–26, signed-rank p<10–45). There was no difference in the change from baseline between stimulus types in visually conditioned mice (p=0.42, signed-rank test). In visually conditioned mice, the drifting grating stimulus response was significantly greater than that of tactilely conditioned mice (rank-sum test, p<10–42). In both conditioning types, firing rates in the offset period were significantly different between stimulus types (two-way ANOVA, conditioning F=0.01, p=0.90; stimulus F=16, p<10–5; interaction F=312, p<10–62; signed-rank p<10–20 for both visually and tactilely conditioned mice).
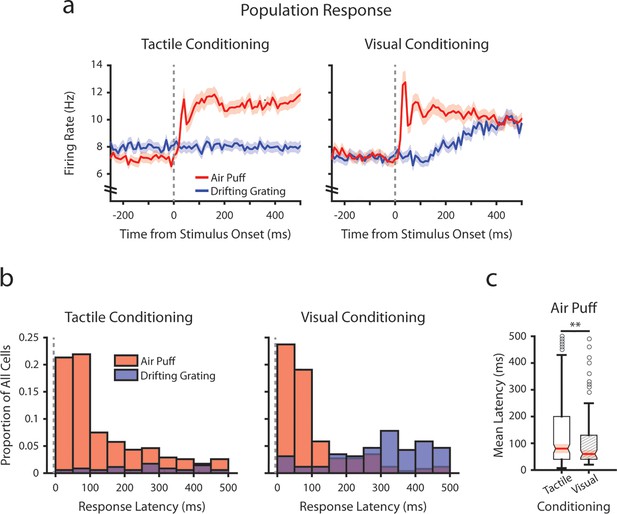
Conditioning alters tactile stimulus response latencies in posterior medial nucleus (POm).
(a) Event-triggered average firing rates of all POm cells (mean ± SEM) aligned to either the air puff (red) or the drifting grating (blue) in tactilely conditioned mice (left) and visually conditioned mice (right), as in Figure 3g. Dashed line indicates stimulus onset. Firing rates are binned at 10 ms. Note the truncated y-axis. (b) Histogram of the stimulus-response latency, as a portion of total recorded POm cells for each conditioning group. Stimulus-response latency was defined as the first time a cell’s firing rate crossed a confidence interval threshold compared to baseline (Methods). Only cells with response latencies less than 500 ms are represented. Red: stimulus-response latency relative to the air puff onset. Blue: response latency relative to the drifting grating onset. (c) Box plot of POm early air puff response latencies (≤ 500 ms). Response latencies in tactilely conditioned mice were later than those in visually conditioned mice (p=0.003, Wilcoxon rank-sum test; tactile conditioning median = 80 ms, IQR=[40, 200], n=226 cells; visual conditioning median=60 ms, IQR=[40, 130], n=101 cells).
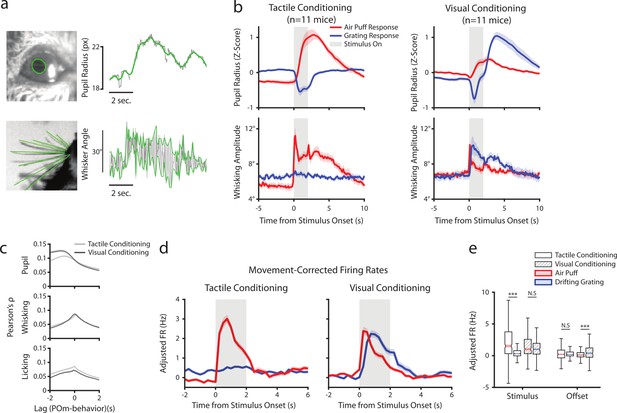
Posterior medial nucleus (POm) correlates with arousal and movement regardless of conditioning.
(a) Pupil and whisker tracking. Top left: Example frame from an eye video with the edge of the pupil highlighted in green. Top Right: A sample trace of pupil radius over time (gray: raw; green: smoothed). Bottom Left: Example frame from a whisker video with whiskers highlighted in green. Bottom Right: Example trace of whisker angle over time (gray) and the most protracted and retracted whisker positions over each whisk cycle (green), which is used to compute whisking amplitude. (b) Whisking amplitude and pupil radius aligned to stimuli in tactilely conditioned mice (n=11 mice, left column) and visually conditioned mice (n=11 mice, right column). Top row: Pupil radius of each mouse aligned to either the air puff (blue) or the drifting grating (red) (Z-scored, mean ± SEM). Bottom row: Whisking amplitude of each mouse aligned to the two stimuli (mean ± SEM). Gray regions indicate stimulus timing. (c) Cross-correlations between POm activity and pupil radius (top), whisking amplitude (middle), and lick rate (bottom). Light gray: Cells from tactilely conditioned mice (mean ± SEM, n=347 cells, 11 mice). Dark gray: Cells from visually conditioned mice (n=257, 11 mice). Negative lags indicate that POm activity precedes the movement, and positive lags indicate that POm activity follows the movement. (d) Movement-corrected firing rates. The firing rate of each cell was fit to a linear model with whisking, licking, pupil radius, and baseline rate as predictors. Model residuals of all cells (mean ± SEM) are aligned to the drifting grating and the air puff, as in B. (e) Box plot of the model residuals of each POm cell during the first second of stimulus onset (‘stimulus period’) and during the first 2 s post-stimulus (‘offset period’) for the same time windows as in 3g,h. Adjusted firing rates in tactilely conditioned mice were significantly higher during the air puff stimulus period than the drifting grating period. (Two-way ANOVA with post-hoc Wilcoxon signed-rank test. Conditioning type F=4.23, p=0.04; stimulus type F=68.2, p<0.001; interaction F=38.5, p<0.001; tactile conditioning Wilcoxon p<0.001; visual conditioning Wilcoxon p=0.09). In visually conditioned mice, adjusted firing rates were higher during the drifting grating offset period than the air puff offset period. (conditioning F=0.003, p=0.95; stimulus F=3.9, p=0.048; interaction F=9.45, p=0.002; tactile conditioning Wilcoxon p=0.94, visual conditioning p<0.001).
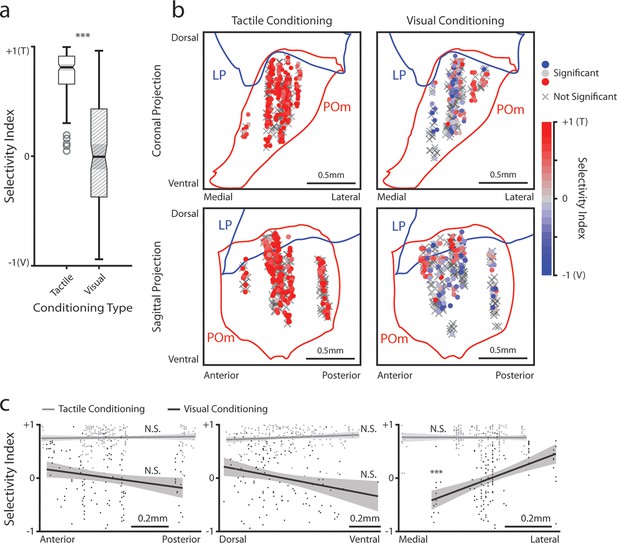
Posterior medial nucleus (POm) responses to tactile and visual stimuli vary based on anatomical location.
(a) Sensory selectivity index for significantly responding POm cells (colored cells in 3d and 3f). Cells with a positive selectivity index more strongly respond to the air puff, and cells with a negative index primarily respond to the drifting grating. Cells from tactilely conditioned mice had a significantly higher selectivity index (Wilcoxon rank sum test, p<0.001; tactile conditioning median = 0.81, IQR=[0.66, 0.91], n=200 cells; visual conditioning median=−0.01, IQR=[–0.37, 0.43], n=120 cells). (b) Position of all POm cells separated by conditioning type. Cell positions are projected on the coronal plane (top row) and sagittal plane (bottom row), as in Figure 2d and e. Significantly responding cells are colored by their selectivity index. Gray Xs indicate unresponsive cells. Positions in the medial-lateral and anterior-posterior axes are jittered for visualization only. (c) Selectivity index of significantly responding cells as a function of their position in POm along the anterior-posterior (left), dorsal-ventral (middle), and medial-lateral axes (right). Light gray: tactile conditioning. Dark gray: visual conditioning. A linear model of selectivity index with experiment type and anatomical position as predictors revealed a significant effect of medial-lateral position and an interaction between position and experiment type. In visually conditioned mice, cells in the lateral portion of POm were more selective to the air puff (model p<10–60, adjusted R2=0.61, conditioning p<10–5, medial-lateral p<10–4, ML-conditioning interaction p<10–4, all other terms p>0.1).
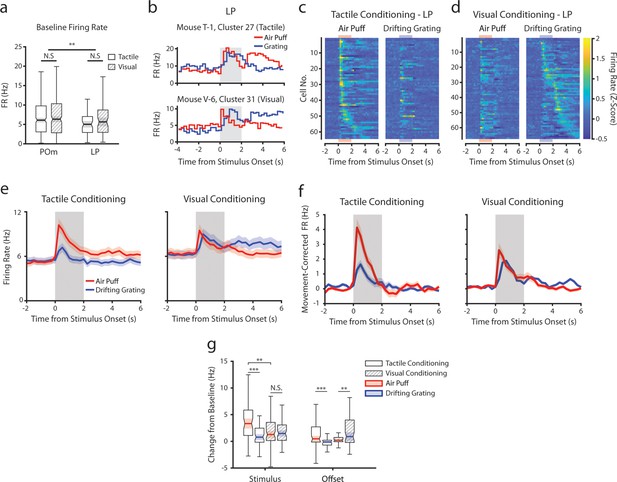
Conditioning also reshapes lateral posterior nucleus (LP) responses.
(a) Baseline firing rates of all posterior medial nucleus (POm) and LP cells separated by conditioning type. POm cells had higher firing rates than LP cells, and firing rates did not significantly differ by conditioning type (two-way ANOVA, region F=8.0, p=0.0049; conditioning type F=0.44, p=0.51; interaction F=1.44, p=0.23). (b) Example LP cells from a tactilely conditioned mouse (top) and a visually conditioned mouse (bottom). Mean firing rates over each trial are aligned to the drifting grating (blue line) and the air puff (red line). Gray region indicates the timing of both stimuli. (c) Firing rate of all LP cells from tactilely conditioned mice aligned to either the air puff (left) or the drifting grating (right). Cells are sorted by the timing of the peak firing rate when aligned to the air puff. n=64 cells, 7 mice, range = 4–16 cells/mouse, median = 7 cells/mouse. (d) Firing rate of all LP cells from visually conditioned mice aligned to either the air puff (left) or the drifting grating (right). Cells are sorted by the timing of the peak firing rate when aligned to the drifting grating. n=67 cells, 8 mice, range=1–19 cells/mouse, median = 6 cells/mouse. (e) Event-triggered average firing rates of all LP cells (mean ± SEM) aligned to either the air puff (red) or the drifting grating (blue) in tactilely conditioned mice (left) and visually conditioned mice (right). Gray region indicates the timing of both stimuli. (f) Movement-corrected firing rates. The firing rate of each cell was fit to a linear model with whisking, licking, pupil radius, and baseline firing rate as predictors. Model residuals of all cells (mean ± SEM) are aligned to the drifting grating and the air puff, as in panel e. (g) Box plots of the change in firing rate from baseline for each LP cell during the first second of stimulus onset (‘stimulus’ period) and during the first 2 s post-stimulus (‘offset’ period). In tactilely conditioned mice, the change from baseline was greater in the air puff stimulus period than the visual stimulus period (two-way ANOVA with post-hoc signed-rank test; conditioning type F=1.268, p=0.13; stimulus type F=15.7, p≤0.001; interaction F=13.25, p=0.003, signed-rank p<10–4). There was no difference in the change from baseline between stimulus types in visually conditioned mice (p=0.91, signed-rank test). The change from baseline in during the air puff stimulus period was also significantly greater in tactilely conditioned LP cells than in visually conditioned cells (p=0.002, rank-sum test). In both conditioning types, firing rates in the offset period were significantly different between stimulus types (two-way ANOVA, conditioning F=2.1, p=0.15; stimulus F=0.15, p=0.69; interaction F=19.5, p<10–4; signed-rank p<10–4 in tactile conditioning, p=0.007 in visual conditioning).
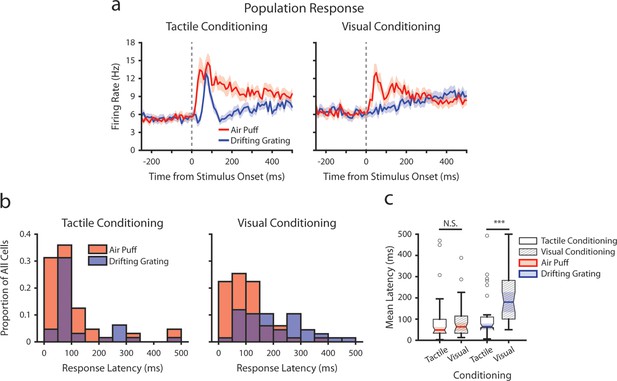
Conditioning alters stimulus response latencies in lateral posterior nucleus (LP).
(a) Event-triggered average firing rates of all LP cells (mean ± SEM) aligned to either the air puff (red) or the drifting grating (blue) in tactilely conditioned mice (left) and visually conditioned mice (right), as in 7e. Dashed line indicates stimulus onset. Firing rates are binned at 10 ms. (b) Histogram of the stimulus-response latency, as a portion of total recorded LP cells for each conditioning group. Stimulus-response latency was defined as the first time a cell’s firing rate crossed a confidence interval threshold compared to baseline (Methods). Only cells with response latencies less than 500 ms are represented. Red: response latency relative to the air puff onset. Blue: response latency relative to the drifting grating onset. (c) Box plot of LP response latencies to the air puff (red) and the drifting grating (blue) in tactilely conditioned mice (unshaded) and visually conditioned (shaded) mice. Drifting grating response latencies were significantly different between conditioning groups (Drifting grating p=0.001, Wilcoxon rank-sum test; tactile conditioning median = 65 ms, IQR=[60, 110], n=32 cells; visual conditioning median = 180 ms, IQR=[100, 280], n=37 cells. Air puff p=0.29, Wilcoxon rank-sum test; tactile conditioning median=55 ms, IQR=[40, 105], n=52; visual conditioning median=70 ms, IQR=[40, 120], n=46).
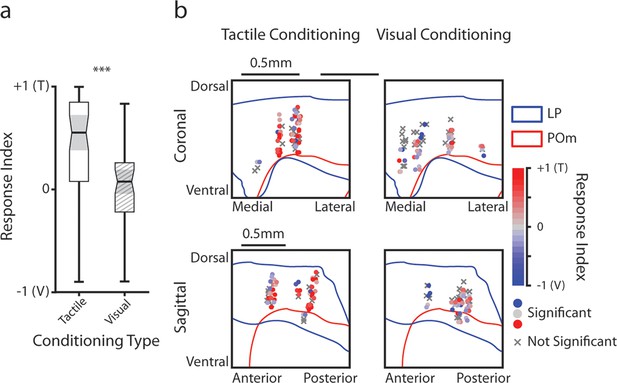
Lateral posterior nucleus (LP) responses to tactile and visual stimuli as a function of anatomical location.
(a) Stimulus selectivity index for significantly responding LP cells. Cells with a positive selectivity index primarily respond to the air puff and cells with a negative index primarily respond to the drifting grating. Cells from tactilely conditioned mice had a significantly higher selectivity index (Wilcoxon rank sum test, p<0.001, tactile conditioning median=0.55, IQR=[0.08, 0.85] n=50 cells; visual conditioning median = 0.08, IQR=[–0.22, 0.26], n=41 cells). (b) Position of all LP cells separated by conditioning type. Cell positions are projected on the coronal plane (top row) and sagittal plane (bottom row), as in Figure 2d and e. Significantly responding cells are colored by their selectivity index. Gray Xs indicate unresponsive cells. Positions in the medial-lateral and anterior-posterior axes are jittered for visualization only.
Tables
Reagent type (species) or resource | Designation | Source or reference | Identifiers | Additional information |
---|---|---|---|---|
Software, algorithms | MATLAB | MathWorks, Inc. | v2022b | |
Software, algorithms | Kilosort | https://github.com/MouseLand/Kilosort (Pennington et al., 2024) | v3 | |
Software, algorithms | Phy | https://github.com/cortex-lab/phy | v2.01b | |
Software, algorithms | Arduino IDE | https://www.arduino.cc/ | v1.8.x | |
Software, algorithms | PsychoPy | https://github.com/psychopy/psychopy/releases/tag/2020.1.1 (Peirce, 2020) | v2020.1.1 | |
Software, algorithms | FIJI | https://imagej.net/software/fiji | N/A | |
Software, algorithms | SHARP-Track | https://github.com/cortex-lab/allenCCF | N/A | |
Chemical compound, drug | DiI (1,1’-Dioctadecyl-3,3,3′,3′-tetramethylindocarbocyanine perchlorate) | Sigma-Aldrich | CAS# 41085-99-8 | |
Chemical compound, drug | Streptavidin, Alexa 647 Conjugate | ThermoFisher | Cat# S21374 |