Restoring vestibular function during natural self-motion: Progress and challenges
Figures
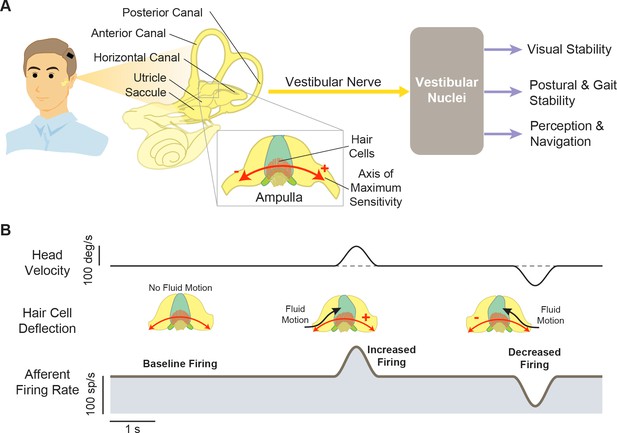
Structures and functions of the vestibular system.
(A) Three orthogonal semicircular canals (anterior, posterior, and horizontal) provide angular head motion signals, and two otolith organs (utricle and saccule) provide linear acceleration signals. These signals travel via afferent fibers to the vestibular nuclei, where they contribute to pathways involved in visual stability, postural stability, and perception and navigation. Inset: Within the ampulla of each semicircular canal, the hair cells are arranged such that their axis of maximum sensitivity is aligned with the fluid motion direction. (B) At rest, afferents fire at a baseline rate (left). During head motion, this baseline is then modulated up (middle) or down (right) to encode head motion in two opposing directions.
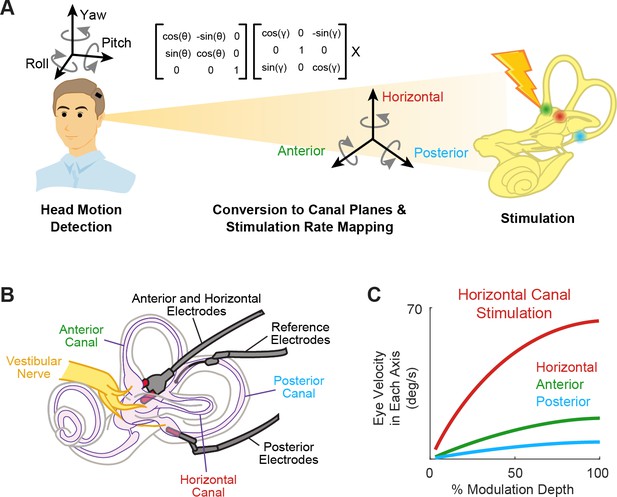
Schematics of the basic functioning of vestibular prostheses.
(A) To restore rotational vestibular inputs to the brain, vestibular prostheses detect head rotations in three dimensions, transform them onto each canal axis, and map each movement into pulsatile electrical stimulation of each associated branch of vestibular nerve. (B) The nerve bundle of each semicircular canals is targeted by an electrode array, allowing for canal-specific stimulation. Adapted from Figure 1 from Chow et al., 2021. (C) Eye movement velocity (in each canal axis) evoked by stimulation of the horizontal canal electrode. Adapted from Figure 6 from Boutros et al., 2019a.
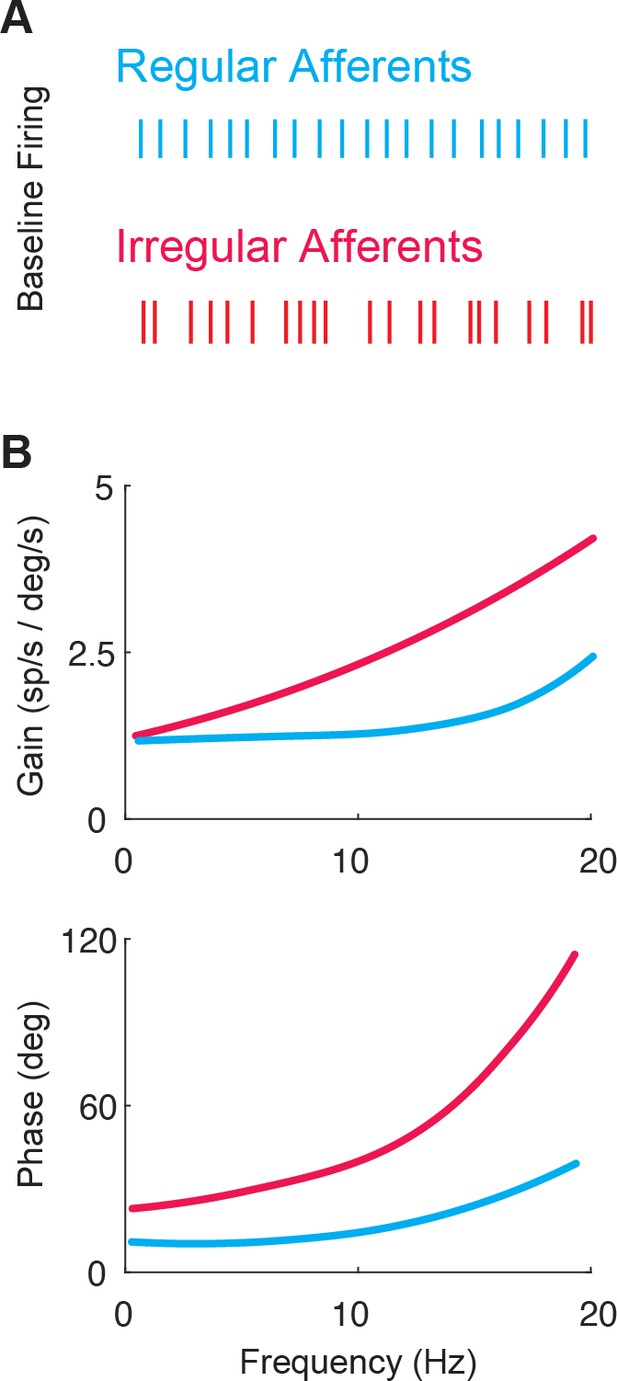
The two classes of vestibular afferents exhibit different baseline firing patterns and responses to head rotations.
(A) Firing patterns of regular (blue) and irregular (red) afferents at rest. (B) While both classes display high-pass tuning, i.e., increasing gain and phase lead as a function motion frequency, irregular afferents show stronger high-pass tuning. Adapted from Figure 4 from Massot et al., 2011.
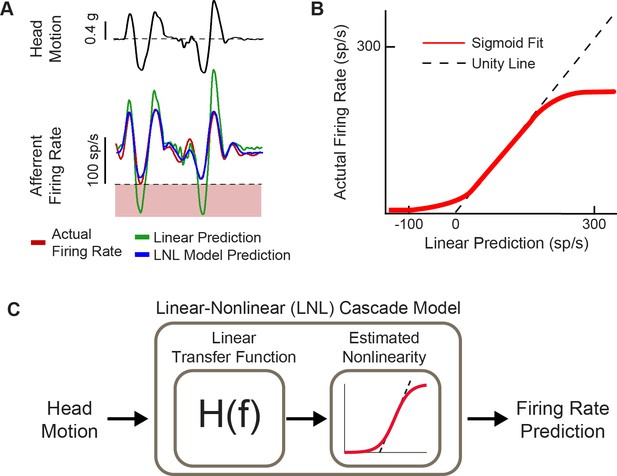
Natural head motion can drive afferent firing to exhibit nonlinearity.
(A) Afferent firing in response to natural head motion is better predicted by a linear-nonlinear (LNL) model compared to a linear model alone. (B) The estimated nonlinearity from afferent firing in (A). (C) Schematic of the LNL model that can accurately predict afferent firing in response to natural head motion. Panels A–C have been adapted from Figure 4 from Schneider et al., 2015.
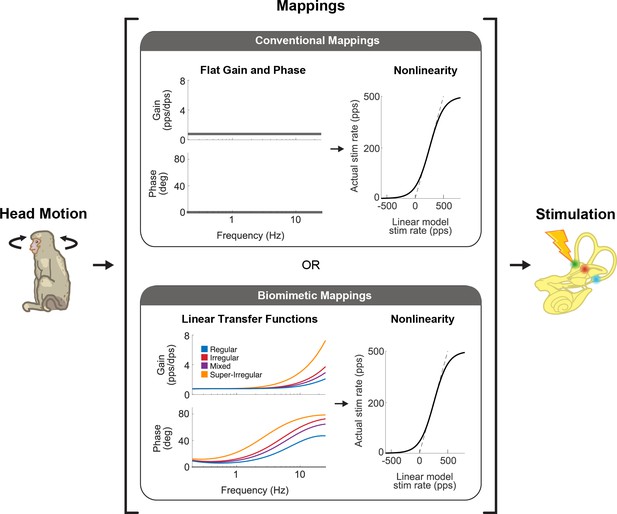
There are two main strategies that vestibular prostheses use to map head motion into the pulse rate of nerve stimulation.
Conventional mappings (top) directly link instantaneous head velocity to a specific stimulation rate (i.e. flat gain and no phase lead, only accounting for nonlinearity). In contrast, biomimetic mappings (bottom) use a linear-nonlinear cascade that well captures how vestibular afferents naturally behave. The linear part mimics the response dynamics of the afferents while the nonlinear part mimics the natural cut-off and saturation behaviors. This figure has been adapted from Figure 1 from Wiboonsaksakul et al., 2022.
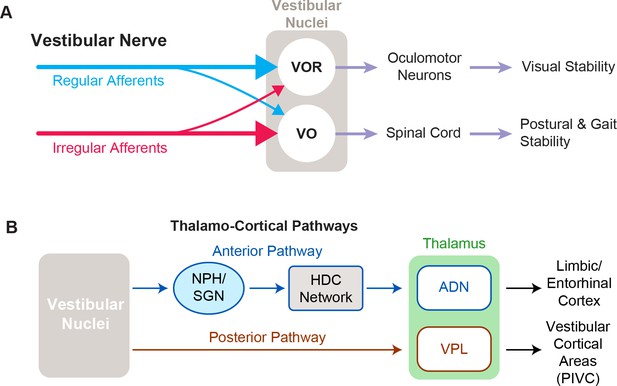
Functional pathways of the vestibular system.
(A) The two main cell types in vestibular nuclei are vestibulo-ocular reflex (VOR) neurons (top), which are involved in visual stability, and vestibular-only (VO) neurons (bottom), which are responsible for maintaining postural and gait stability. (B) Vestibular signals travel through two different pathways to reach the anterior and posterior parts of the thalamus, which are involved in navigation and self-motion perception, respectively.
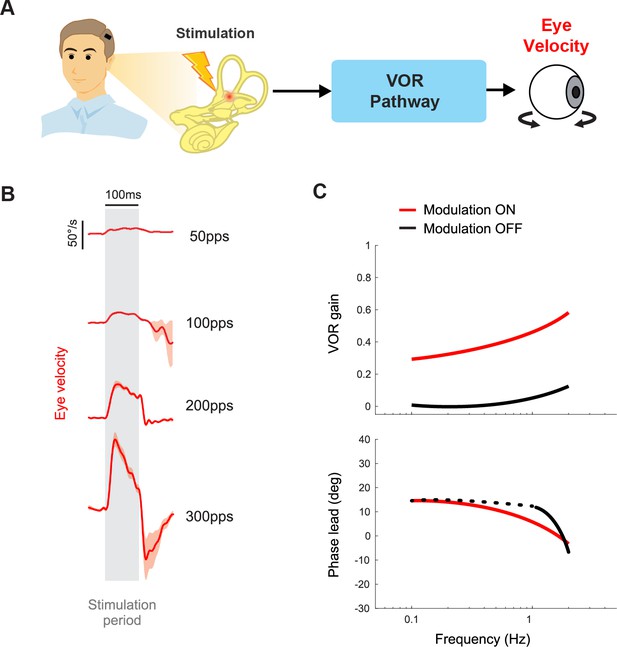
Vestibular prostheses can restore vestibulo-ocular reflex (VOR) functions to improve visual stability.
(A) Pathway diagram of the VOR. Head motion information is encoded in prosthetic stimulation pulses to vestibular nerve. This information, in turn, generates eye movement that counteracts the head movement. (B) Prosthetic pulse trains can evoke VOR eye movement associated with the specific canal being stimulated. Adapted from Figure 1 from Mitchell et al., 2013. (C) In normal operation where the prosthetic pulses encode the head motion, gain and phase of the VOR response can be calculated. Data from a human clinical trial shows improved VOR gains when prosthesis modulation is on. Adapted from Figure 8 from Boutros et al., 2019a.
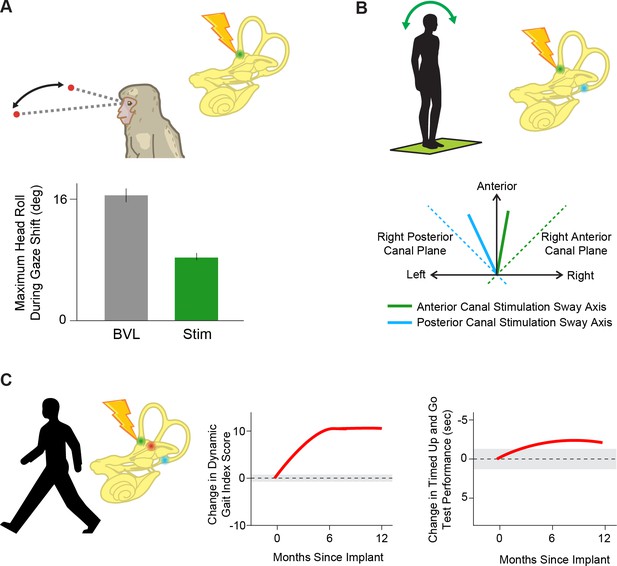
Prosthetic stimulation can drive reflexes and help restore balance.
(A) Providing head velocity information in the anterior canal plane through a prosthesis during gaze shifts in a rhesus macaque reduces overall head motion. Adapted from Figure 6 from Thompson et al., 2016. (B) Stimulating the vertical (anterior, green and posterior, blue) semicircular canals individually in humans drives postural sway (solid lines) in the direction of that canal’s plane (dotted lines). Adapted from Figure 3 from Phillips et al., 2013. (C) Stimulating all three semicircular canals independently with a prosthesis leads to improved scores on dynamic gait index and timed up and go tests, important clinical tests of posture and gait during everyday activities. Adapted from Figure 2 from Chow et al., 2021.
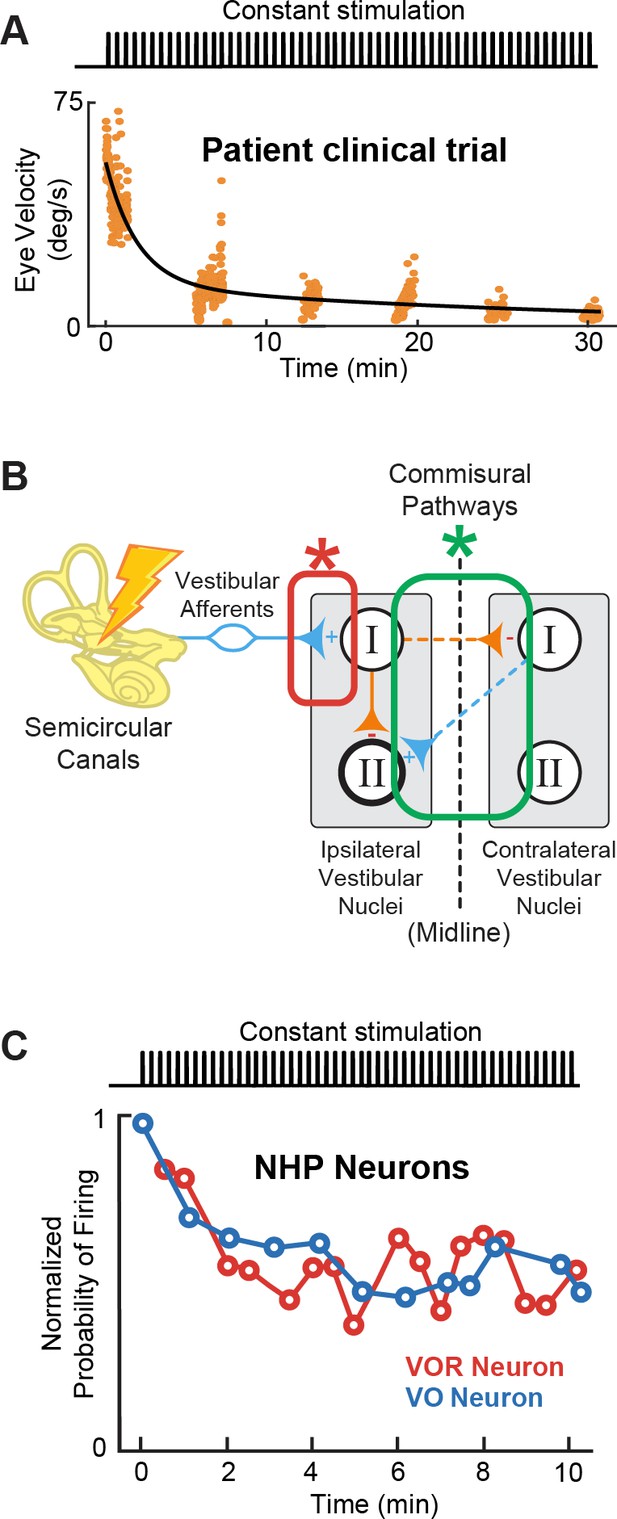
Adaptation to prosthetic pulses at the afferent-vestibular nuclei synapses is problematic.
(A) Vestibulo-ocular reflex (VOR) adaptation to onset of baseline prosthetic stimulation from a clinical trial. Adapted from Figure 5 from Boutros et al., 2019a. (B) Compensatory synaptic changes occur at the afferent-vestibular nuclei synapses (red) as well as in central commissural pathways (green). (C) Problematic adaptation of VOR and VO neurons to prosthetic stimulation. Adapted from Figure 6 from Mitchell et al., 2016; Mitchell et al., 2017.
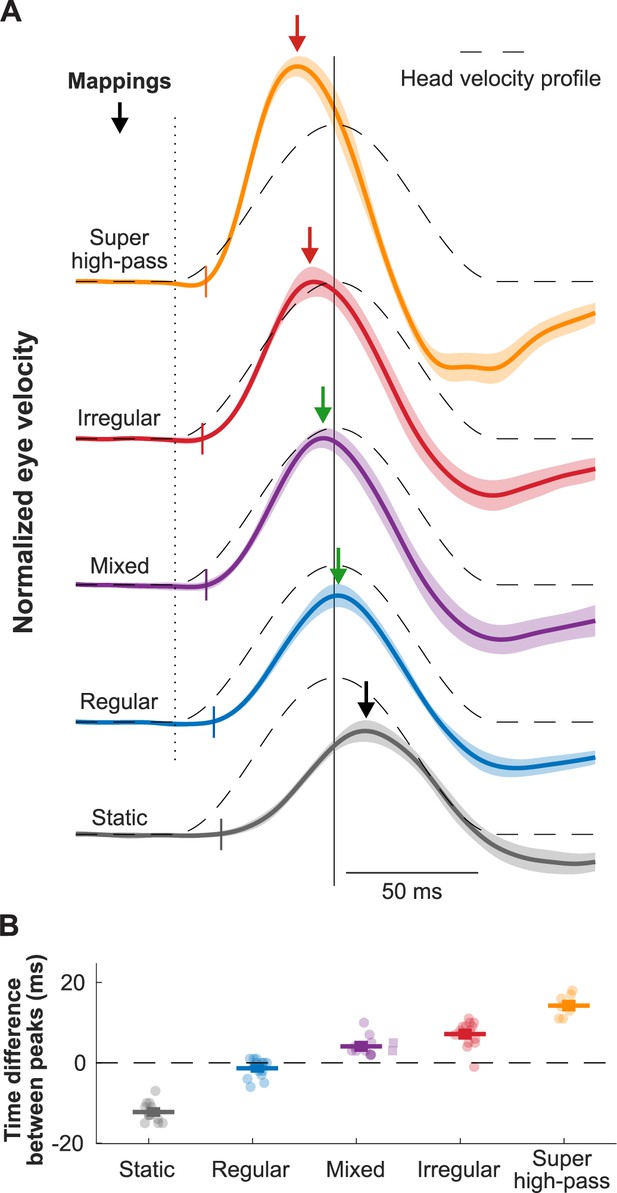
Biomimetic encoding of head motion improves timing accuracy of vestibulo-ocular reflex (VOR).
(A) Normalized traces of the evoked eye movements during transient head movement using different mappings. Dashed lines show the inverted head velocity. Dotted vertical line denotes the start of the head movement. Solid vertical line denotes the peak of the inverted head movement. Short colored vertical lines indicate the estimated latency. Arrows show the peak of the eye movement response. (B) Quantification of the difference between the peak timing of the head and eye velocity for the traces in (A). This figure has been adapted from Figure 4 from Wiboonsaksakul et al., 2022.
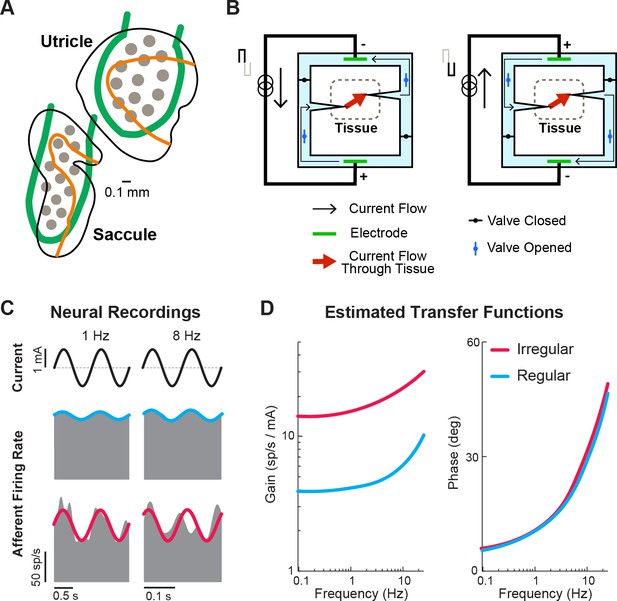
Ongoing developments toward more naturalistic stimulation in vestibular prostheses.
(A) Schematic of the electrode array for stimulation of the otolith organs. Gray circles denote electrode contacts. Orange lines denote the line of polarity reversal (i.e. where different hair cells are sensitive to different motion directions). Adapted from Figure 1 from Hageman et al., 2020. (B) Schematic of a safe direct current stimulator, which can continuously deliver direct current in one direction to tissue while preventing unwanted electro-chemical reactions at the electrode interface. Adapted from Figure 1 from Aplin and Fridman, 2019. (C) Firing rate of recorded canal afferents in response to galvanic vestibular stimulation (GVS). (D) Estimated transfer functions of the afferent response to GVS from the neural data in (C). Panels C and D have been adapted from Figure 2 from Kwan et al., 2019.