Synaptic proteins promote calcium-triggered fast transition from point contact to full fusion
Figures
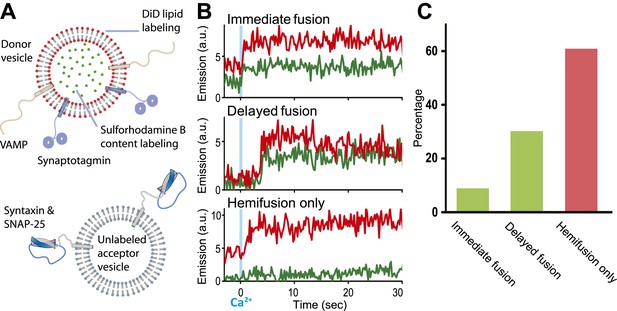
Single vesicle–vesicle microscopy for monitoring changes in the membrane and contents upon Ca2+-injection. (A) Labeling and reconstitution scheme of our single-vesicle lipid/content mixing system. Other factors and proteins can be added to the system. The detailed experimental protocol is described in (Kyoung et al., 2012) with modifications as described in ‘Materials and methods’. (B) Representative real-time fluorescence intensity traces with donor (synaptobrevin and synaptotagmin 1) and acceptor (syntaxin and SNAP-25) vesicles (red/upper traces: lipid dye fluorescence intensity, green/lower traces: content dye fluorescence intensity). Time point 0 indicates the instance of Ca2+ injection at 500 μM (blue vertical lines). Shown are instances of ‘immediate’ fusion, as defined by a content dye fluorescence intensity jump during the first 600 ms time bin upon Ca2+-injection along with a simultaneous jump in lipid dye fluorescence intensity, ‘delayed’ fusion, as defined by a content dye fluorescence intensity jump during a subsequent time bin, and ‘hemifusion-only’, as defined by a lipid dye fluorescence intensity jump without a content dye fluorescence intensity jump during the observation period of 50 s. For illustration purposes, only 30 s of the observation period are shown since there was no change in the subsequent 20 s. (C) Bar graph of the percentage of immediate, delayed fusion, and hemifusion-only events involving SNAREs and synaptotagmin 1. The bar graph was normalized with respect to the number of fluorescent spots that exhibited at least one lipid-mixing event during the observation period. Note, that there are fewer immediate fusion events compared to our previous experiments that were carried out at higher Ca2+ concentration (Kyoung et al., 2011).
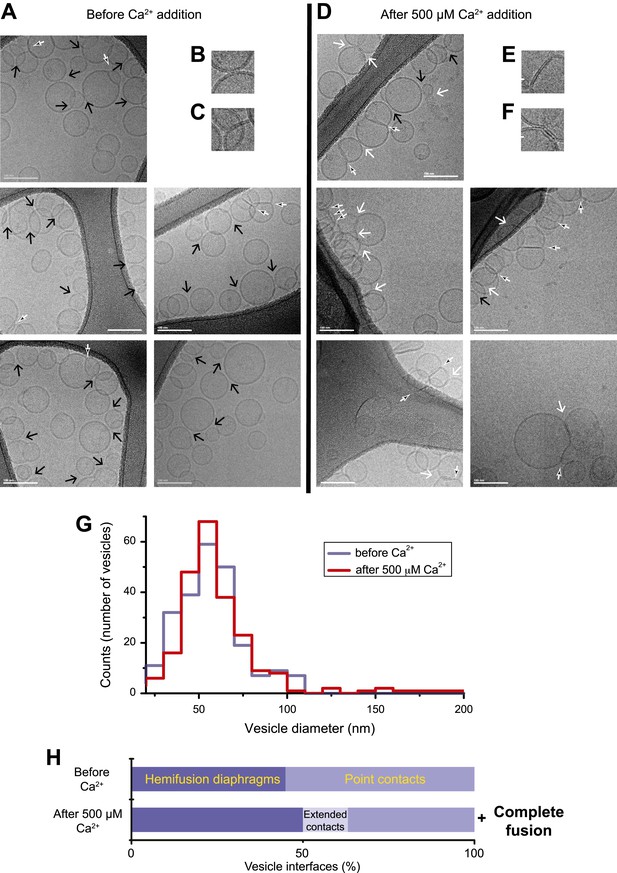
Imaging of donor/acceptor interface morphologies by cryo-EM before and after Ca2+ addition. (A–F) Cryo-EM images of mixtures of donor (synaptobrevin and synaptotagmin 1) and acceptor (syntaxin and SNAP-25) vesicles before (A–C) and approximately 35 s after (D–F) 500 μM Ca2+ addition (panels B, C, E, and F are close-up views). Vesicles were imaged in the holes of the substrate carbon film, visible as the darker areas in the image, in conditions that clearly show the lipid bilayers (‘Materials and methods’). The particular images shown in this figure were selected out of total of 16 (before Ca2+ addition) and 21 (after Ca2+ addition) EM micrographs, respectively, with emphasis on showing point contacts before Ca2+ addition and extended interfaces after Ca2+ addition in order to illustrate the variety of these particular states. Arrows indicate interfaces between vesicles that are approximately perpendicular to the direction of the projection (see ‘Materials and methods’ for definitions of all contact and interface types). Large black arrow: point contact (representative close-up in B), small black/white arrow: hemifusion diaphragm (representative close-ups in C and E), and large white arrow: extended close contact (representative close-up in F). Scale bars in A and D are 100 nm, and 20 nm in B, C, E, and F. (G) Distribution of vesicle sizes before and after Ca2+ addition. Vesicle diameters were calculated from all cryo-EM images in both conditions (‘Materials and methods’). (H) Bar graph of the percentage of various vesicle interfaces, that is, point contacts, hemifusion diaphragms, and extended close contacts (including a few instances of mixed, i.e., extended/hemifused, interfaces), normalized with respect to the total number of interfaces observed before and after addition of 500 μM Ca2+, respectively (‘Materials and methods’). In addition to the changes in the distribution of vesicle interfaces upon Ca2+ addition, some amount of complete fusion between vesicles occurred as indicated by the shift of the diameter distribution in panel G towards larger values. Source files of all cryo-EM micrographs used for the quantitative analysis are available in the Figure 2—source data 1.
-
Figure 2—source data 1
Source files for cryo-EM data.
This zip archive contains all cryo-EM images used for the quantitative analyses shown in Fig. 2. The folder named “No_Ca++” contains the images before Ca++ addition (individual files are named P3_1_**. tif or jpg), and folder named “With_Ca++” contains the images ∼35s after Ca++ addition (individual files are named P3_3_**.tif or jpg).
Images were collected in low dose conditions at 200 kV acceleration voltage on a CM200 FEG electron microscope (FEI) with a 2k × 2k Gatan UltraScan 1000 camera, at 50,000× magnification and 1.5 mm underfocus.
The full resolution data were exported as 16 bit “tif” files (2048 × 2048 pixels, scale 0.2 nm/pixel at specimen (the corresponding files have the extension “tif”). Note that these files cannot not be viewed with a standard picture viewer, but must be viewed with a program, such as “ImageJ”. To facilitate easier viewing, the original images were converted to smaller (1024×1024, 0.4 nm/pixel), contrast adjusted jpeg images (8 bits) for easy and immediate visualization with commonly used picture viewers (the corresponding files have the extension “jpg”).
- https://doi.org/10.7554/eLife.00109.005
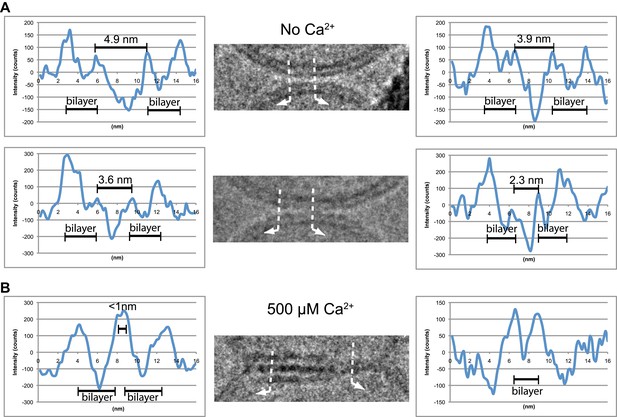
Image density profile analysis of selected vesicle–vesicle interfaces. (A) Image density profile analysis of two representative point contacts between vesicles at zero Ca2+. Dotted lines indicate 2 nm thick sections selected from the cryo-EM images. These sections were used to generate the profiles shown to the left and the right of the close-up views in the center (for details, see ‘Materials and methods’). (B) Image density profile analysis of a transition from extended close contact to hemifusion diaphragm after addition of 500 μM Ca2+. As in panel A, selected sections are indicated by dotted lines, and the corresponding profiles are shown the left and right of the close-up view in the center.
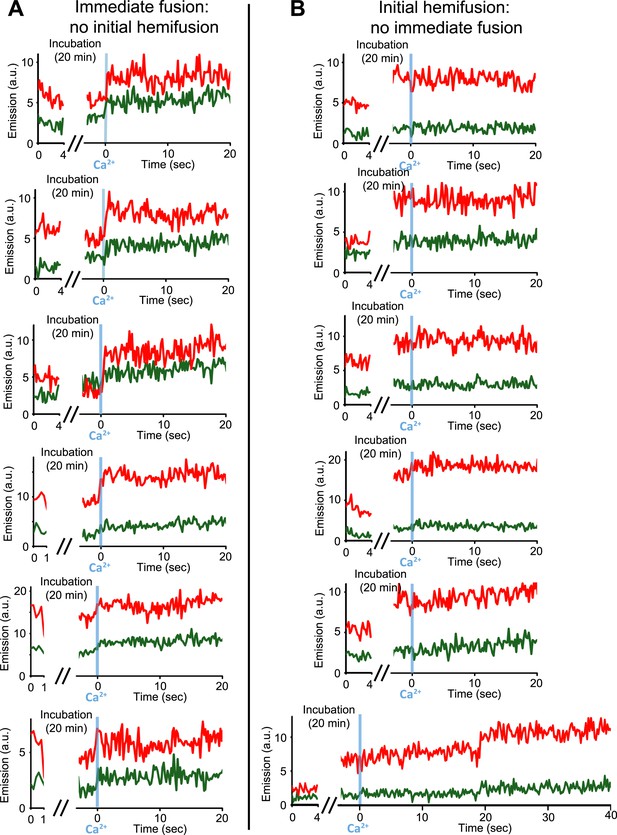
Upon Ca2+-injection, immediate fusion events start form hemifusion-free point contacts whereas initially hemifused states are slow to fuse. (A) Representative real-time fluorescence intensity traces of instances of immediate fusion with imaging of initial florescence intensity levels right after addition of donor (synaptobrevin and synaptotagmin 1) to acceptor vesicles (syntaxin and SNAP-25) (red/upper traces: lipid dye fluorescence intensity, green/lower traces: content dye fluorescence intensity). Time point 0 indicates the instance of Ca2+ injection at 500 μM (blue vertical lines). As in Figure 1, immediate fusion is defined as a content dye fluorescence intensity jump that occurs during the first 600 ms time bin upon Ca2+ injection. In all cases, no significant (i.e., above noise level) lipid fluorescence intensity change was observed during the incubation period, which excludes the possibility of a hemifused initial state before Ca2+ triggering. Moreover, ‘all’ (seven) observed traces with immediate fusion upon Ca2+ injection exhibited this behavior. (B) Representative real-time fluorescence intensity traces of cases where the membranes are hemifused prior to Ca2+-injection. Initial hemifusion was characterized by a significant increase (i.e., above noise level) in only lipid dye fluorescence intensity between the initial recording and at the end of incubation period at 0 Ca2+. For approximately 400 fluorescent spots co-localized in both the initial recording and at the end of the incubation period, we observed 51 traces showing (1) an increase in lipid dye fluorescence and (2) no change in content dye fluorescence intensity during the incubation period but before the Ca2+ injection. For most of the 51 cases, no content mixing was observed upon Ca2+-injection during the observation period of 50 s except for two cases (see one example in the bottom panel) where delayed content mixing occurred at a later time. For illustration purposes only 20 s of the 50 s observation period are shown all traces but one since there was no change in the subsequent 30 s.
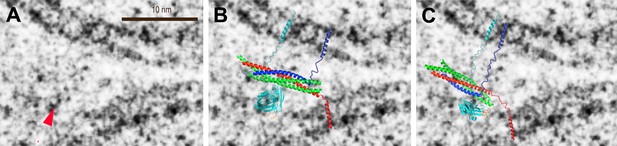
Modeling of putative protein density in a pre-fusion point contact state. (A) Close up view of Figure 3A, top middle panel, around a significant density feature (greater than a factor of two above noise level) that is not part of the membranes (red arrow). Only the density features on the left-hand side of the panel were considered since the carbon grid (dark feature in the lower right corner in Figure 3A, top middle panel) may have affected protein interactions. (B) Overlay of the model of the complex of the C2A–C2B fragment of synaptotagmin 1 (cyan) and the neuronal SNARE complex (synaptobrevin—blue, syntaxin—red, SNAP-25—green) based on 34 single-molecule FRET experiments (Choi et al., 2010). The last four C-terminal helical turns of synaptobrevin were modeled as a random coil. (C) Overlay of a model of the complex of the C2B domain of synaptotagmin 1 and the neuronal SNARE complex based on proximity between the C2B domain polybasic region and residues D186, D193 of SNAP-25, both of which show sizeable chemical shift perturbations in NMR experiments upon complex formation, among other perturbations (Dai et al., 2007). Six C-terminal α-helical turns of synaptobrevin and one α-helical turn of syntaxin were modeled as a random coil. The transmembrane domains were modeled as α-helices, and the linkers as random coils. See ‘Materials and methods’ for details.
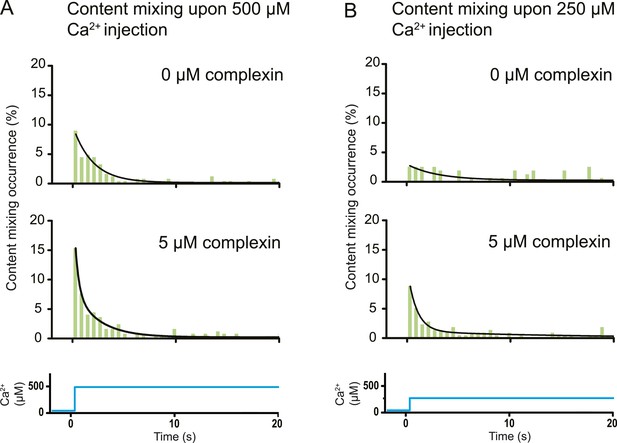
Effect of complexin on Ca2+-triggered fusion probability. Probability of fusion vs time upon 500 μM (A) or 250 μM (B) Ca2+-injection (i.e., occurrence of Ca2+-triggered content-mixing events vs time) with and without 5 μM complexin. The same donor (synaptobrevin and synaptotagmin 1) and acceptor (syntaxin and SNAP-25) vesicles were used as in Figure 1. Histograms were normalized to the number of respective fluorescence intensity time traces showing at least one lipid-mixing event during the observation period of 50 s (see ‘Materials and methods’ for details). For illustration purposes only 20 s of the 50 s observation period are shown since there were few events in the subsequent 30 s; a time binning of 600 ms was used. Black lines are fits to exponential decay functions over the entire 50 secs. For 500 μM Ca2+, in the absence of complexin the fitted function is f(t) = 0.0015 + 0.096e−t/1.93 and it is 10 times more likely compared to a two-exponential fit, and in the presence of 5 μM complexin the fitted function is f(t) = 0.0021 + 0.084e−t/2.38 + 0.18e−t/0.36 and it is 107 times more likely compared to a single exponential fit. For 250 μM Ca2+, in the absence of complexin the fitted function is f(t) = 0.0026 + 0.027e−t/2.98 and it is three times more likely compared to a two-exponential fit, and in the presence of 5 μM complexin the fitted function is f(t) = 0.0005 + 0.10e−t/0.89 + 0.011e−t/14.5 and it is 1014 times more likely compared to a single exponential fit.
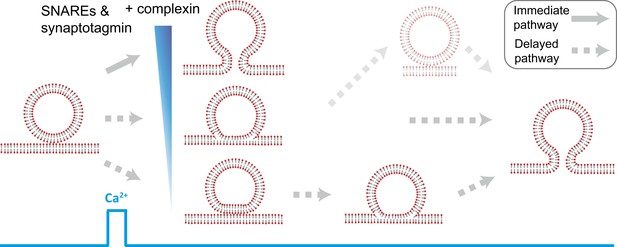
Model of multiple fusion pathways upon Ca2+ injection starting from a point contact. Complexin synchronizes Ca2+-triggered fusion by increasing the number of immediate fusion processes from point contact to fusion pore opening, relative to delayed fusion pathways involving stable hemifusion diaphragms and other long-lived intermediates.
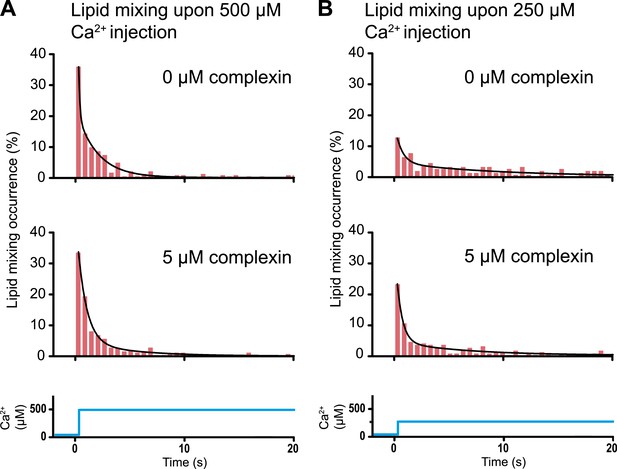
Histograms of the occurrence of Ca2+-triggered lipid-mixing events with and without 5 μM complexin, corresponding to the experiments shown in Figure 6. (A) Ca2+ injection at 500 μM. (B) Ca2+ injection at 250 μM. Black lines are fits to exponential decay functions over the entire 50 s. For 500 μM Ca2+, in the absence of complexin the fitted function is f(t) = 0.0014 + 2.66e−t/0.11 + 0.22e−t/1.94 and it is 1018 times more likely compared to a single exponential fit, and in the presence of 5 μM complexin the fitted function is f(t) = 0.0006 + 0.42e−t/0.81 + 0.05e−t/4.76 and it is 1010 times more likely compared to a single exponential fit. For 250 μM Ca2+, in the absence of complexin the fitted function is f(t) = 0.0023 + 0.13e−t/0.61 + 0.05e−t/9.05 and it is 106 times more likely compared to a single exponential fit, and in the presence of 5 μM complexin the fitted function is f(t) = 0.003 + 0.33e−t/0.51 + 0.05e−t/6.34 and it is 1014 times more likely compared to a single exponential fit.