Correction: Systems analysis of the CO2 concentrating mechanism in cyanobacteria
Figures
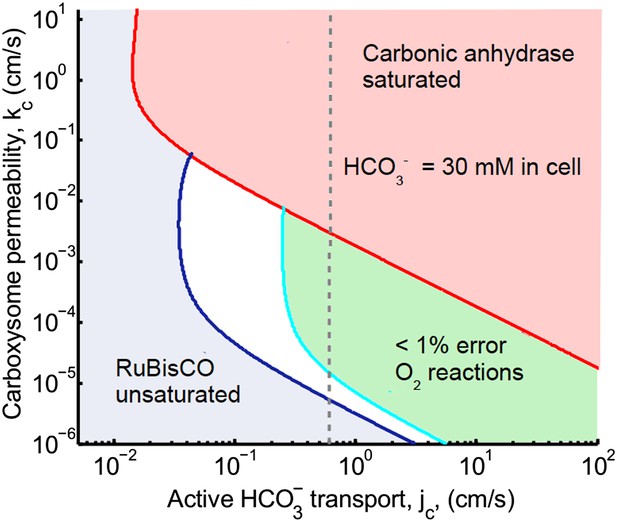
Plotted are the parameter values at which the CO2 concentration reaches some critical value.
The leftmost line (dark blue) indicates for what values of jc and kc the CO2 concentration in the carboxysome would half-saturate RuBisCO (Km). The middle line (light blue) indicates the parameter values which would result in a CO2 concentration where 99% of all RuBisCO reactions are carboxylation reactions and only 1% are oxygenation reactions when O2 concentration is 260 μM. To the left of the line the error is greater and to the right it is smaller. The right most (red) line indicates the parameter values which result in carbonic anhydrase saturating. Here the rate of conversion from CO2 to , α = 0, so there is no CO2 scavenging or facilitated uptake of CO2. The dotted line (grey) shows the kc and jc values, where the concentration in the cytosol is 30 mM. The concentration in the cytosol does not vary appreciably with kc in this parameter regime, and reaches 30 mM at . All other parameters, such as reaction rates are held fixed and the values can be found in the Tables 1 and 2.
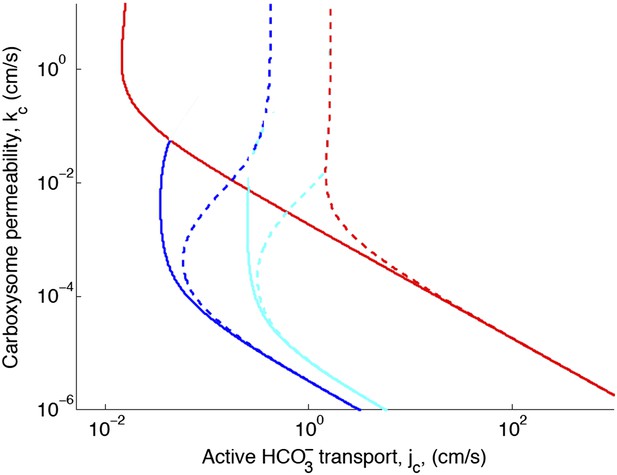
Phase space for transport and carboxysome permeability for varying diffusion in the carboxysome.
Solid lines show lines of constant CO2 concentration in the carboxysome for , or the diffusion constant of small molecule in water. Dashed lines show the same lines of constant CO2 concentration, bur for , or the diffusion constant of a small molecule in a 60% sucrose solution.
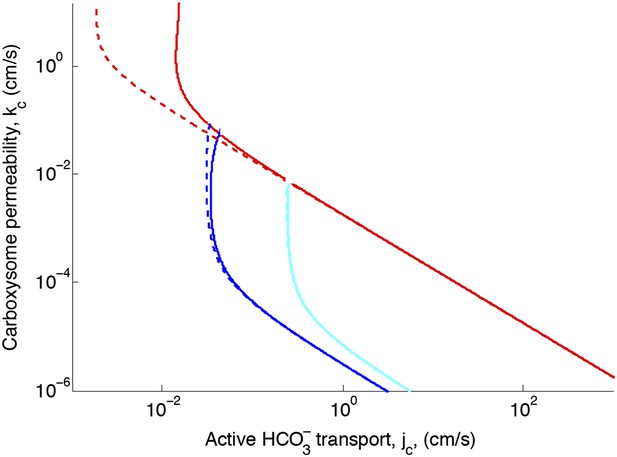
Effect of CO2 scavenging or facilitated uptake on phase space for transport, jc, and carboxysome permeability, kc.
Plotted are the parameter values at which CO2 concentration reaches some critical value. The leftmost line (dark blue) indicates for what values of jc and kc the CO2 concentration in the carboxysome would saturate RuBisCO. The middle line (light blue) indicates the parameter values which would result in a CO2 concentration where 99% of all RuBisCO reactions are carboxylation reactions and only 1% are oxygenation reactions when O2 concentration is 260 μM. The rightmost (red) line indicates the parameter values which result in carbonic anhydrase saturating. Here α = 0 cm/s (solid lines) and α = 1 cm/s (dashed line), showing the effect of CO2 scavenging or facilitated uptake on the phase space. All other parameters, such as reaction rates are held fixed and the values can be found Table 1.
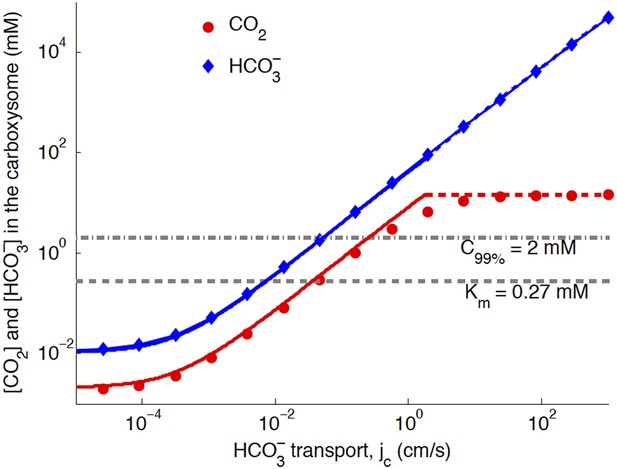
Numerical solution (diamonds and circles) and analytic solutions (carbonic anhydrase unsaturated, solid lines, and saturated, dashed lines) correspond well.
transport is varied, and all other system parameters are held constant. The CO2 concentration above which RuBisCO is saturated is Km (grey dashed line). The CO2 concentration where the oxygen reaction error rate will be 1% is C99% (grey dash-dotted line). The transition between carbonic anhydrase being unsaturated and saturated happens where the two analytic solutions meet (where the dashed and solid red lines meet). Below a critical value of transport, the level of transport is lower than the leaking through the cell membrane. For these simulations and solutions the carboxysome permeability was kc = 10−3 cm/s (in previous version kc = 6 × 10−3 cm/s was used).
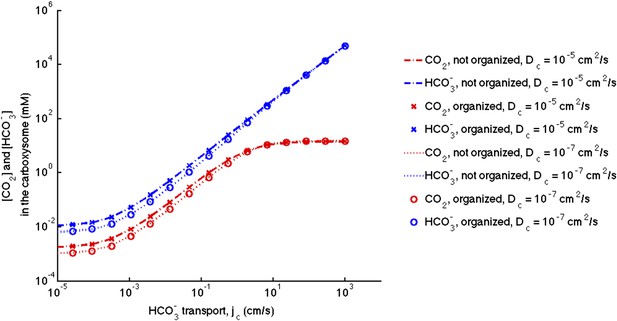
No effect of localizing carbonic anhydrase to the shell of the carboxysome.
We assume the same amount of carbonic anhydrase and RuBisCO activity for each simulation and compare the case with the enzymes evenly distributed throughout the carboxysome to the case where the carbonic anhydrase is localized to the inner carboxysome shell. The (-.-) lines are for no organization and (x) for organized with Dc = 10−5 cm2/s. The (…) lines are for no organization and (o) are for organized with Dc = 10−5 cm2/s.
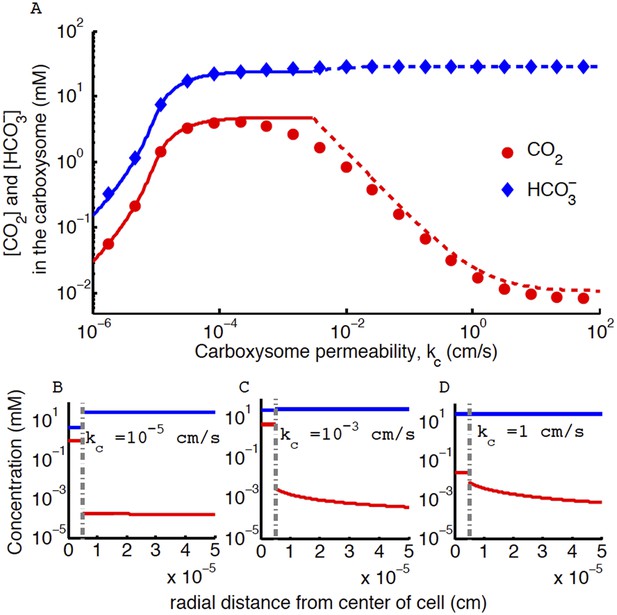
Concentration of CO2 in the carboxysome with varying carboxysome permeability.
(A) Numerical solution (diamonds and circles) and analytic solutions (carbonic anhydrase unsaturated, solid lines, and saturated, dashed lines) correspond well. On all plots CO2 (red circle) (blue diamond). Concentration in the cell along the radius, r, with carboxysome permeability kc = 10−5 cm/s (B), kc = 10−3 cm/s (C), kc = 1 cm/s (D). Grey dotted lines in (B), (C), (D) indicate location of the carboxysome shell boundary. The transition from low CO2 at high permeability (D) to maximum CO2 concentration at optimal permeability (C) occurs at . At low carboxysome permeability (B) diffusion into the carboxysome is slower than consumption. For all subplots the rate of CO2 to conversion at the cell membrane, α = 0 cm/s and uptake, jc = 0.6 cm/s. Qualitative results remain the same with varying jc, increasing α will increase the gradient of CO2 across the cell as CO2 is converted to at the cell membrane.
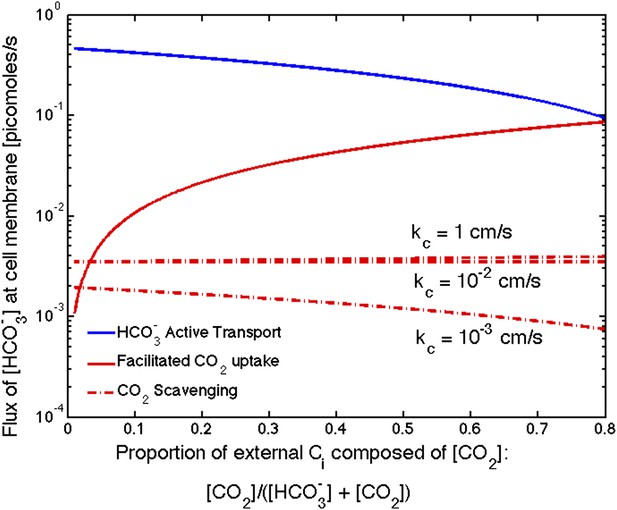
Size of the flux in one cell from varying sources, as the proportion of CO2 to outside the cell changes changes.
We show results for three carboxysome permeabilities, kc, and only the scavenging is effected. Total external inorganic carbon is 15 μM, jc = 1 cm/s and . Scavenging is negligibly small for all values of kc shown. Unless there is very little in the environment, transport seems to be more efficient than CO2 facilitated uptake.
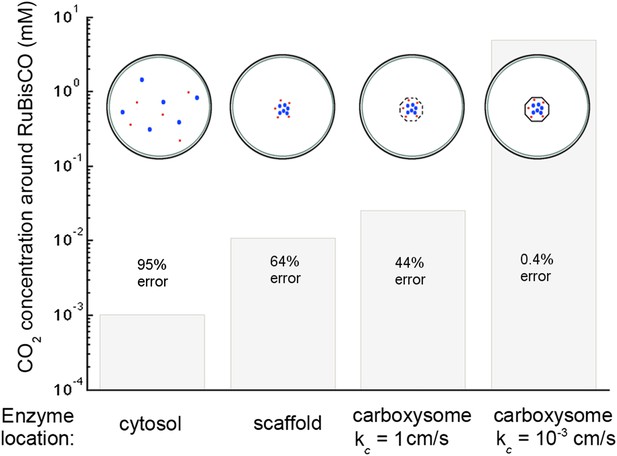
Concentration of CO2 achieved through various cellular organizations of enzymes, where we have selected the transport level such that the concentration in the cytosol is 30 mM.
O2 concentration is 260 μM. The oxygenation error rates, as a percent of total RuBisCO reactions are indicated on the concentration bars. The cellular organizations investigated are RuBisCO and carbonic anhydrase distributed throughout the entire cytosol, co-localizing RuBisCO and carbonic anhydrase on a scaffold at the center of the cell without a carboxysome shell, RuBisCO and carbonic anhydrase encapsulated in a carboxysome with high permeability at the center of the cell, and RuBisCO and carbonic anhydrase encapsulated in a carboxysome with optimal permeability at the center of the cell.
Tables
Fate of carbon brought into the cell for jc = 0.6 cm/s and kc = 1 × 10−3 cm/s
Formula | % Of flux | ||
---|---|---|---|
transport | jcHout | 3.26 × 10−4 | |
leakage | 3.2 × 10−4 | 98.6% | |
CO2 leakage | 4.5 × 10−6 | 1.4% | |
carboxylation | 8.2 × 10−8 | 0.03% | |
oxygenation | 6.7 × 10−10 | 2 × 10−4 % |
Fate of carbon brought into the cell for jc = 0.06 cm/s and kc = 1 × 10−3 cm/s
Formula | % Of flux | ||
---|---|---|---|
transport | jcHout | 2.8 × 10−5 | |
leakage | 2.7 × 10−5 | 96.6% | |
CO2 leakage | 8.8 × 10−7 | 3.2% | |
carboxylation | 5.4 × 10−8 | 0.2% | |
oxygenation | 2.3 × 10−9 | 8 × 10−3% |