Vision: Running into differences
Imagine you are walking down a busy street. As you move, your brain receives visual information that it uses, alongside other sensory inputs, to guide your next steps. Traditionally, it was thought that the parts of the brain that process sensory information, such as the primary visual cortex, were largely unaffected by body movements. It therefore came as a shock when a group of researchers discovered that running significantly increased neuronal activity in the primary visual cortex of mice (Niell and Stryker, 2010; Figure 1A).
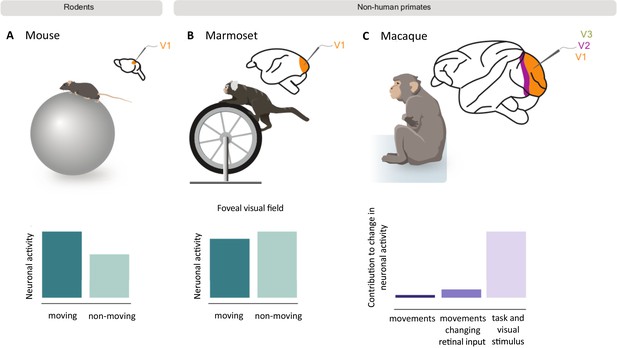
How movement impacts neuronal activity in the visual cortex of rodents and non-human primates.
Mice (A) and marmosets (B) were placed on a rotating treadmill (top) with their heads fixed in position so they could view visual stimuli on a screen. The neuronal activity of their primary visual cortex was then recorded as they ran or sat still. In mice, neuronal activity was higher when running (dark green bar) compared to when the mice were still (light green bar; bottom left graph). However, movement did not cause the same effect in marmosets, and slightly decreased the activity of neurons that respond to the foveal visual field (bottom middle graph). A similar study in macaques (C) also found that running only slightly increased neuronal activity in the primary (V1) and mid-level (V2 and V3) visual cortex. The bar graph shows how much of this change in activity was the result of spontaneous body movements (dark purple), movements that change visual inputs to the retina (purple), and visual stimuli and task-related variables (light purple). Panel A and B are schematic representations of the results from Liska et al., 2023; panel C represents the results from Talluri et al., 2023.
This rise in activity was different from the increase caused by arousal (Vinck et al., 2015), suggesting that the observed change in the primary visual cortex was due to the motion of the body. However, it remained unclear whether body movement also influences sensory processing in non-human primates, which are evolutionarily closer to humans. Now, in eLife, Jacob Yates, Alexander Huk and co-workers – including John Liska and Declan Rowley as joint first authors – report how running impacts the primary visual cortex of marmosets (Liska et al., 2023).
The team (who are based at the University of Texas at Austin, University of Maryland, University of Berkeley, California, and UCLA) recorded the neuronal activity of marmosets as they ran or remained stationary on a treadmill whilst looking at a screen, similar to the experimental set-up used in the previous mouse study (Figure 1B). The results were then compared to publicly available datasets from the experiments conducted in mice.
Analysis of the mouse dataset confirmed that body movements increase neuronal activity in the primary visual cortex, in agreement with previous studies. However, Liska, Rowley et al. found that running did not cause the same pronounced effect in marmosets. Intriguingly, the behavior of neurons in the primary visual cortex depended on which parts of the visual field they respond to: movement decreased the activity of foveal neurons, which receive inputs from the center of the visual field, but slightly increased the activity of peripheral neurons, which respond to inputs from the outermost parts of the visual field. Unlike humans and non-human primates, rodents do not have a fovea, which may contribute to the observed difference between the marmosets and mice.
Another possibility is that rodents and monkeys process non-visual inputs (such as running or attention) differently in their visual cortices. To test this, Liska, Rowley et al. applied a model to their data which tests to what degree non-visual inputs adjust the way neurons respond to visual stimuli. The team found that the model was able to explain a significant proportion of the data from both the marmosets and mice, including the variations they had observed between experimental trials in each species. This suggests that the different response to body movement between the marmosets and mice is not because they process non-visual factors in distinct ways.
A recent study similarly showed that body movement only minimally increases neuronal activity in the visual cortex of macaques, another non-human primate (Talluri et al., 2023; Figure 1C). The researchers found that only a small fraction of the activity in the visual cortex was due to spontaneous body movements (such as scratching). Instead, the majority was explained by movements which changed the visual inputs arriving to the brain, or visual stimuli and task-related variables, such as when the monkey received a reward.
So, how do such striking differences between mice and two primate species come about? Liska, Rowley et al. propose several possible mechanisms. First, the distinction may be due to neurons in the primary visual cortex responding differently to the neurotransmitter acetylcholine: in primates, acetylcholine generally suppresses activity in the visual cortex, but in rodents, it increases activity (Disney et al., 2007; Pakan et al., 2016). Second, because the visual system of a primate has more specialized areas than the rodent visual system, factors like running may influence those regions rather than condensing its effects to the primary visual cortex. Third, the neurons in the primary visual cortex of mice may be more sensitive to running because they receive substantial direct projections from premotor areas of the brain which plan and organize movement (Leinweber et al., 2017) – this is note the case for the neurons in the primary visual cortex of monkeys (Markov et al., 2014).
The findings of Liska, Rowley et al. open up several exciting avenues for exploring how the brain integrates sensory inputs with movements. Categorizing the movements as relevant or irrelevant to the task at hand might shed light on which aspects of non-visual inputs affect sensory areas the most. More generally, by investigating how visual areas in mice and monkeys respond to non-visual inputs, it may be possible to uncover overarching mechanisms that are used by sensory systems to integrate and sample information from the environment.
References
-
Activity in primate visual cortex is minimally driven by spontaneous movementsNature Neuroscience 26:1953–1959.https://doi.org/10.1038/s41593-023-01459-5
Article and author information
Author details
Publication history
Copyright
© 2024, Psarou et al.
This article is distributed under the terms of the Creative Commons Attribution License, which permits unrestricted use and redistribution provided that the original author and source are credited.
Metrics
-
- 594
- views
-
- 46
- downloads
-
- 0
- citations
Views, downloads and citations are aggregated across all versions of this paper published by eLife.
Download links
Downloads (link to download the article as PDF)
Open citations (links to open the citations from this article in various online reference manager services)
Cite this article (links to download the citations from this article in formats compatible with various reference manager tools)
Further reading
-
- Computational and Systems Biology
- Neuroscience
Accumulating evidence to make decisions is a core cognitive function. Previous studies have tended to estimate accumulation using either neural or behavioral data alone. Here, we develop a unified framework for modeling stimulus-driven behavior and multi-neuron activity simultaneously. We applied our method to choices and neural recordings from three rat brain regions—the posterior parietal cortex (PPC), the frontal orienting fields (FOF), and the anterior-dorsal striatum (ADS)—while subjects performed a pulse-based accumulation task. Each region was best described by a distinct accumulation model, which all differed from the model that best described the animal’s choices. FOF activity was consistent with an accumulator where early evidence was favored while the ADS reflected near perfect accumulation. Neural responses within an accumulation framework unveiled a distinct association between each brain region and choice. Choices were better predicted from all regions using a comprehensive, accumulation-based framework and different brain regions were found to differentially reflect choice-related accumulation signals: FOF and ADS both reflected choice but ADS showed more instances of decision vacillation. Previous studies relating neural data to behaviorally inferred accumulation dynamics have implicitly assumed that individual brain regions reflect the whole-animal level accumulator. Our results suggest that different brain regions represent accumulated evidence in dramatically different ways and that accumulation at the whole-animal level may be constructed from a variety of neural-level accumulators.
-
- Neuroscience
Our propensity to materiality, which consists in using, making, creating, and passing on technologies, has enabled us to shape the physical world according to our ends. To explain this proclivity, scientists have calibrated their lens to either low-level skills such as motor cognition or high-level skills such as language or social cognition. Yet, little has been said about the intermediate-level cognitive processes that are directly involved in mastering this materiality, that is, technical cognition. We aim to focus on this intermediate level for providing new insights into the neurocognitive bases of human materiality. Here, we show that a technical-reasoning process might be specifically at work in physical problem-solving situations. We found via two distinct neuroimaging studies that the area PF (parietal F) within the left parietal lobe is central for this reasoning process in both tool-use and non-tool-use physical problem-solving and can work along with social-cognitive skills to resolve day-to-day interactions that combine social and physical constraints. Our results demonstrate the existence of a specific cognitive module in the human brain dedicated to materiality, which might be the supporting pillar allowing the accumulation of technical knowledge over generations. Intensifying research on technical cognition could nurture a comprehensive framework that has been missing in fields interested in how early and modern humans have been interacting with the physical world through technology, and how this interaction has shaped our history and culture.