Target protein identification in live cells and organisms with a non-diffusive proximity tagging system
Figures
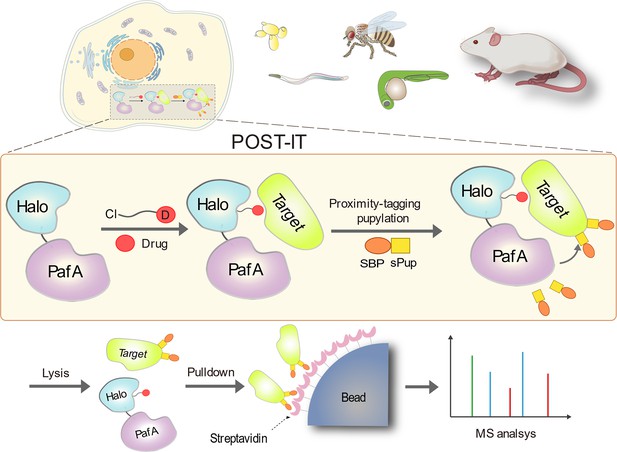
Schematic illustration of the POST-IT system for target identification.
Upon the introduction of a drug-specific HaloTag ligand derivative, POST-IT tags target proteins with sPup, a tagging process known as pupylation, in live cells or organisms. Subsequently, these proteins are enriched and identified via mass spectrometry (MS) analysis.

PafA fused with HaloTag exhibits high activity across a wide temperature range.
(A) Schematic representation of Halo-PafA and three Pup substrates (HB-Pup, TS-sPup, SBP-sPup). HB, TS, and SBP refer to 6×His and BCCP, twin-STII (Strep-tag II), and streptavidin binding peptide, respectively. (B) In vitro self-pupylation of Halo-PafA (1 μM) with HB-Pup, TS-sPup, or SBP-sPup (10 μM) at 37°C, 28°C, and 20°C for 30 min. (C) Polypupylated Halo-PafAs were observed as high molecular weight bands after 2 hr pupylation reaction with three Pup substrates (HB-Pup, TS-sPup, SBP-sPup) at 37°C. Arrows indicate the Halo-PafA band without pupylation.
-
Figure 1—figure supplement 1—source data 1
Uncropped and labeled gels of Coomassie blue staining for Figure 1—figure supplement 1.
- https://cdn.elifesciences.org/articles/102667/elife-102667-fig1-figsupp1-data1-v1.zip
-
Figure 1—figure supplement 1—source data 2
Raw unedited gels of Coomassie blue staining for Figure 1—figure supplement 1.
- https://cdn.elifesciences.org/articles/102667/elife-102667-fig1-figsupp1-data2-v1.zip

Lysine-mutated Pup substrates bypass polypupylation while retaining activities comparable to wild-type Pup.
(A) A schematic shows the lysine residues in HB-Pup, TS-sPup, and SBP-sPup. (B) In a pupylation assay, Halo-PafA exhibits dose-dependent self-pupylation with different concentrations of HB-Pup, TS-sPup, or TS-sPupK61R for 30 min. (C) Time-dependent polypupylation becomes evident, particularly after 1 hr incubation, as seen by high molecular weight bands. (D) Removing all lysine residues from TS-sPup completely prevents polypupylation. The asterisk indicates a possible experimental error causing a failed pupylation. (E) Eliminating lysine residues in SBP and sPup completely abolishes polypupylation. Halo-PafA was incubated with various concentrations of SBP-sPup, sSBP-sPupK61R, or SBPK4R-sPupK61R for 30 min. (F) Longer incubations do not induce polypupylation but enhance multipupylation in the cases of sSBP-sPupK61R and SBPK4R-sPupK61R. All reactions were performed with 1 μM of Halo-PafA and 10 μM of HB-Pup, TS-sPup, or TS-sPupK61R at 37°C. Yellow arrowheads point to polypupylated bands that are not detected in polypupylation-free sPup substrates. Black arrowheads and arrows indicate multipupylated bands and Halo-PafA, respectively.
-
Figure 1—figure supplement 2—source data 1
Uncropped and labeled gels of Coomassie blue staining for Figure 1—figure supplement 2.
- https://cdn.elifesciences.org/articles/102667/elife-102667-fig1-figsupp2-data1-v1.zip
-
Figure 1—figure supplement 2—source data 2
Raw unedited gels of Coomassie blue staining for Figure 1—figure supplement 2.
- https://cdn.elifesciences.org/articles/102667/elife-102667-fig1-figsupp2-data2-v1.zip

Optimization of Halo-PafA.
(A) Schematic illustration of Halo-PafA derivatives: Halo8KR-PafA, which includes eight lysine-to-arginine mutations to abolish self-pupylation, and Halo-PafAS126A, which contains a serine-to-alanine mutation at position 126 to decrease depupylase activity of PafA. (B) In vitro self-pupylation results show that Halo8KR-PafA exhibits minimal pupylation levels, similar to PafA alone. Halo-PafAS126A demonstrates reduced self- and polypupylation while enhancing multipupylation. Reactions were conducted with 1 μM of a Halo-PafA derivative and 10 μM of TS-sPup or TS-sPupK61R at 37°C for 30 min. Yellow arrowheads indicate polypupylated bands, while black arrowheads and arrows indicate multipupylated bands and Halo-PafA, respectively.
-
Figure 1—figure supplement 3—source data 1
Uncropped and labeled gel of Coomassie blue staining for Figure 1—figure supplement 3.
- https://cdn.elifesciences.org/articles/102667/elife-102667-fig1-figsupp3-data1-v1.zip
-
Figure 1—figure supplement 3—source data 2
Raw unedited gel of Coomassie blue staining for Figure 1—figure supplement 3.
- https://cdn.elifesciences.org/articles/102667/elife-102667-fig1-figsupp3-data2-v1.zip
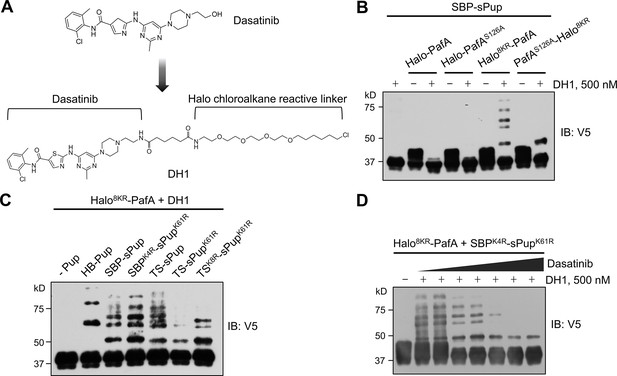
Optimized Halo-PafA and Pup substrates efficiently promote pupylation in vitro in a proximity-dependent manner.
(A) The chemical structure of DH1, an HTL derivative of dasatinib. (B) Comparison of proximity-tagging by different Halo-PafA derivatives on in vitro pupylation. (C) Comparison of different Pup substrates on in vitro pupylation with 500 nM DH1. (D) Pupylation levels by DH1 decrease with an increasing amount of dasatinib as a competitor, ranging from 0.2 μM to 10 μM. (B–D) All reactions were conducted with 1 μM of a Halo-PafA derivative, 10 μM of one of the different Pup substrates, and 0.5 μM of a purified short SRC with a 2xV5 tag, SRC(247-536)-2xV5, at 37°C for 30 min. Pupylation levels of SRC were assessed by immunoblot (IB) using an anti-V5 antibody.
-
Figure 2—source data 1
File containing labeled original western blots for Figure 2.
- https://cdn.elifesciences.org/articles/102667/elife-102667-fig2-data1-v1.zip
-
Figure 2—source data 2
Original files for western blots displayed in Figure 2.
- https://cdn.elifesciences.org/articles/102667/elife-102667-fig2-data2-v1.zip

Synthesis of dasatinib-Halo derivative 1 (DH1).
Reagents and conditions: (a) HATU, DIPEA, DMF, RT, 2 hr; (b) NaOH, THF, H2O, RT, 2 hr. RT, room temperature.
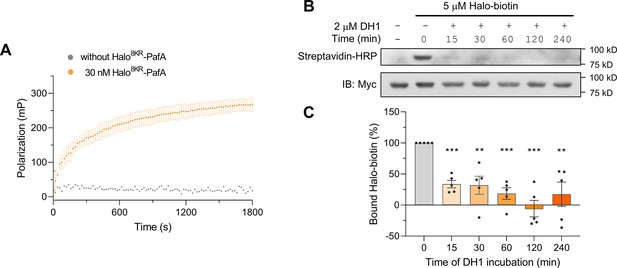
Halo8KR-PafA demonstrates efficient labeling with HaloTag ligands.
(A) Fluorescence polarization (FP) assay using 2 nM of HaloTag Alexa Fluor-488 (Halo-AF488) at 25°C. n = 2. Data are shown as mean ± s.d. (B) Western blot analysis for the Halo-Biotin competition assay. Halo-Biotin (5 μM) was incubated with 1 μM of Myc-Halo8KR-PafA at 37°C for 1 hr after a preincubation with 2 μM of DH1 for the indicated time period. Levels of bound Halo-Biotin were assessed by streptavidin-HRP. (C) Quantitative analysis of western blot results shown in (B). n = 5. Data are shown as mean ± s.e.m. p-Values were calculated by an unpaired two-sided t-test. **p<0.01, ***p<0.001; versus no DH1 competition.
-
Figure 2—figure supplement 3—source data 1
File containing labeled original western blots for Figure 2—figure supplement 3.
- https://cdn.elifesciences.org/articles/102667/elife-102667-fig2-figsupp3-data1-v1.zip
-
Figure 2—figure supplement 3—source data 2
Original files for western blots displayed in Figure 2—figure supplement 3.
- https://cdn.elifesciences.org/articles/102667/elife-102667-fig2-figsupp3-data2-v1.zip

Optimized Halo-PafA and Pup substrates efficiently promote pupylation in vitro in a proximity-dependent manner.
(A) Comparison of proximity-tagging by different Halo-PafA derivatives on in vitro pupylation using TSK8R-sPupK61R. (B) Comparison of different Pup substrates, SBPK4R-sPupK61R and TSK8R-sPupK61R, on in vitro pupylation. All reactions were conducted with 1 μM of a Halo-PafA derivative, 10 μM of a sPup substrate, and 0.5 μM of a purified short SRC with a 2xV5 tag, SRC(247-536)-2xV5, at 37°C for 30 min. Pupylation levels of SRC were assessed by immunoblot (IB) using an anti-V5 antibody.
-
Figure 2—figure supplement 4—source data 1
File containing labeled original western blots for Figure 2—figure supplement 4.
- https://cdn.elifesciences.org/articles/102667/elife-102667-fig2-figsupp4-data1-v1.zip
-
Figure 2—figure supplement 4—source data 2
Original files for western blots displayed in Figure 2—figure supplement 4.
- https://cdn.elifesciences.org/articles/102667/elife-102667-fig2-figsupp4-data2-v1.zip
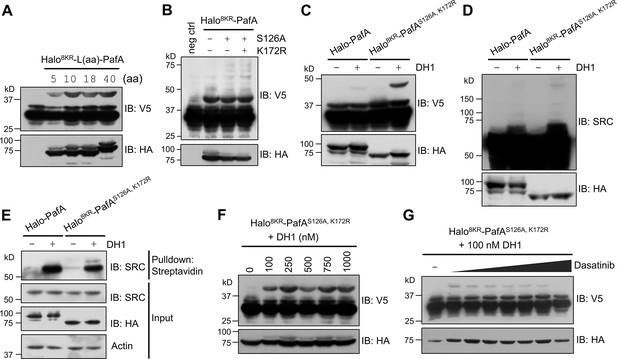
POST-IT can label target proteins in live cells.
(A) Effect of linker length between Halo8KR and PafA on target-labeling. HEK293T cells were co-transfected with HA-Halo8KR-PafA containing the specified length of linker, SBPK4R-sPupK61R, and SRC(247-536)-2xV5. (B) Introducing mutations S126A and K172R into PafA significantly enhances its proximity-tagging efficiency on target. (C–E) Comparison of labeling activity of Halo-PafA and Halo8KR-PafAS126A,K172R, a form used for POST-IT. (F, G) POST-ITDH1 mediates proximity-tagging in a DH1-dose dependent manner (F), an effect completely inhibited by competitive dasatinib, ranging from 0.025 to 25.6 μM (G). Pupylation levels of exogenously expressed short SRC (C, F, G), endogenous SRC (D), or pull-downed endogenous SRC (E) were assessed by immunoblot (IB) analysis using antibodies against V5-tag or SRC for exogenous or endogenous SRC, respectively. In all experiments, SBPK4R-sPupK61R was used for co-transfection, and cells were treated with 500 nM DH1, except in (F, G).
-
Figure 3—source data 1
File containing labeled original western blots for Figure 3.
- https://cdn.elifesciences.org/articles/102667/elife-102667-fig3-data1-v1.zip
-
Figure 3—source data 2
Original files for western blots displayed in Figure 3.
- https://cdn.elifesciences.org/articles/102667/elife-102667-fig3-data2-v1.zip

Test of small molecule-induced proximity-tagging by PafA in HEK293T cells.
(A) Schematic illustration of plasmid constructs and a model of proximity-tagging by PafA. In the presence of rapamycin, HA-FKBP-EGFP and V5-FRB-mKate2-PafA heterodimerize via rapamycin, facilitating proximity for PafA to pupylate HA-FKBP-EGFP. (B) V5-FKBP-EGFP is highly pupylated only in the presence of rapamycin, while FRB-mKate2-PafA undergoes substantial self-pupylation regardless of rapamycin treatment. (C) The pupylation levels of the target, HA-FKBP-EGFP, and the self-pupylation of V5-FRB-mKate2-PafA increase in an HB-Pup dose-dependent manner. (D) Evaluation of different Pup substrates on proximity-tagging and self-pupylation. (B–D) HEK293T cells were co-transfected with HA-FKBP-EGFP, V5-FRB-mKate2-PafA, and an indicated Pup substrate. Twenty-four hours post-transfection, the cells were treated with 100 nM rapamycin for an additional 24 hr. Pupylation levels of HA-FKBP-EGFP and V5-FRB-mKate2-PafA were assessed by immunoblot (IB) using antibodies against HA-tag and V5-tag, respectively.
-
Figure 3—figure supplement 1—source data 1
File containing labeled original western blots for Figure 3—figure supplement 1.
- https://cdn.elifesciences.org/articles/102667/elife-102667-fig3-figsupp1-data1-v1.zip
-
Figure 3—figure supplement 1—source data 2
Original files for western blots displayed in Figure 3—figure supplement 1.
- https://cdn.elifesciences.org/articles/102667/elife-102667-fig3-figsupp1-data2-v1.zip
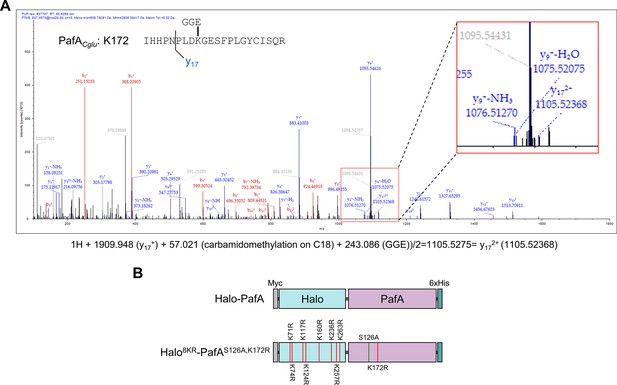
Identification of self-pupylated lysine residues in PafA.
(A) Mass spectrometry analysis identified K172 as the most frequently pupylated lysine in PafA. An inset provides an enlarged view of the Nano-LC-MS/MS mass spectrum. (B) Constructs of Halo-PafA and Halo8KR-PafAS126A,K172R containing 8KR mutations in HaloTag and mutations of S126A and K172R in PafA.
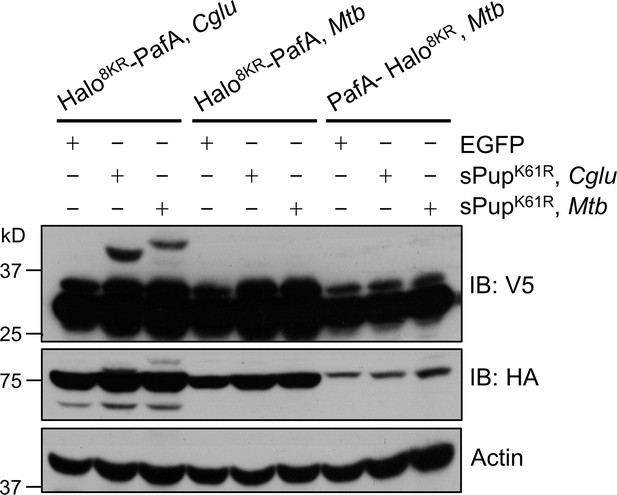
PafA from Cglu outperforms PafA from Mtb in the POST-IT system in live cells.
HEK293T cells were co-transfected with one of the HA-Halo-PafA derivatives and a sPup substrate as shown above, along with SRC(247-536)-2xV5 as a target. Twenty-four hours later, cells were incubated with 250 nM DH1 for an additional 24 hr. Immunoblot (IB) analysis reveals that PafA from Cglu exhibits robust labeling with sPupK61R, whether from Cglu or Mtb, whereas PafA from Mtb shows no detectable labeling.
-
Figure 3—figure supplement 3—source data 1
File containing labeled original western blots for Figure 3—figure supplement 3.
- https://cdn.elifesciences.org/articles/102667/elife-102667-fig3-figsupp3-data1-v1.zip
-
Figure 3—figure supplement 3—source data 2
Original files for western blots displayed in Figure 3—figure supplement 3.
- https://cdn.elifesciences.org/articles/102667/elife-102667-fig3-figsupp3-data2-v1.zip
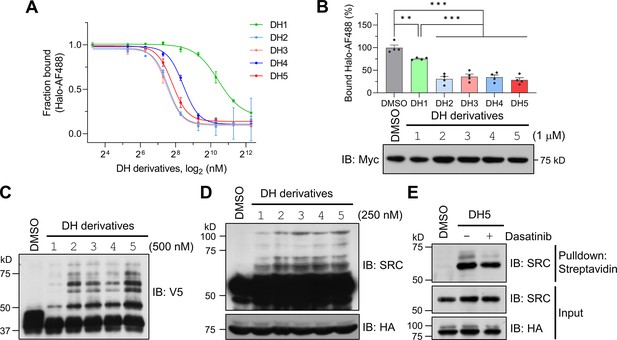
Dasatinib-HTL (DH) derivatives with modified and longer linkers enhances POST-IT performance.
(A) In vitro binding assay for DH derivatives via fluorescence polarization (FP) assay. Purified Myc-Halo-PafA (15 nM) and 1 nM Halo-AF488 were incubated with various DH derivatives in a serial dilution at 37°C for 30 min prior to FP measurement. n = 2. Data are shown as mean ± s.d. (B) Cellular binding assay for DH derivatives by FP measurement. HEK293T cells transfected with Myc-Halo-PafA were later incubated with 1 μM of each DH derivative or DMSO for 3 hr, followed by cell lysate incubation with 2 nM of Halo-AF488 at 37°C for 30 min. n = 4. Data are shown as mean ± s.e.m. p-Values were calculated by an unpaired two-sided t-test. *p<0.05; **p<0.01; ***p<0.001; versus DMSO. Immunoblot (IB) presents a representative image of the input levels for each condition, demonstrating that treatment with DH derivatives did not alter the expression levels of Myc-Halo-PafA. (C) In vitro labeling activity comparison among DH derivatives. Purified recombinant Halo8KR-PafA (0.5 μM), SBPK4R-sPupK61R (10 μM), and SRC(247-536)-2xV5 (0.5 μM) were incubated with 0.5 μM of each DH derivative at 37°C for 30 min. (D, E) In cellular labeling activity comparison among DH derivatives. HA-Halo8KR-PafA and SBPK4R-sPupK61R were used for co-transfection, and cells were treated with 250 nM of various DH derivatives. Pupylation levels of purified short SRC (C), endogenous SRC (D), or pull-downed endogenous SRC (E) were assessed by IB using anti-V5 or anti-SRC antibodies for exogenous or endogenous SRC, respectively.
-
Figure 4—source data 1
File containing labeled original western blots for Figure 4.
- https://cdn.elifesciences.org/articles/102667/elife-102667-fig4-data1-v1.zip
-
Figure 4—source data 2
Original files for western blots displayed in Figure 4.
- https://cdn.elifesciences.org/articles/102667/elife-102667-fig4-data2-v1.zip
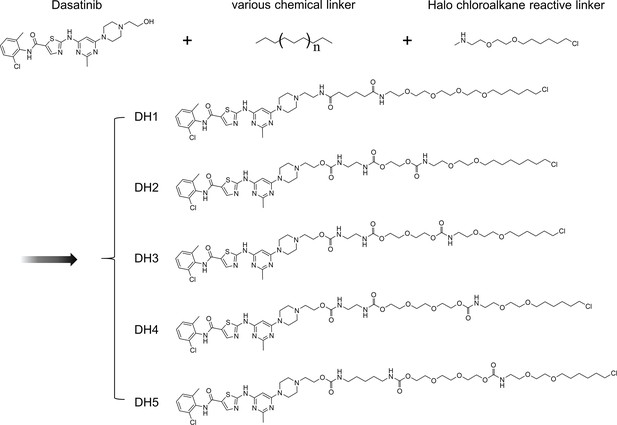
Chemical structures of dasatinib and its HTL derivatives with varied linkers.
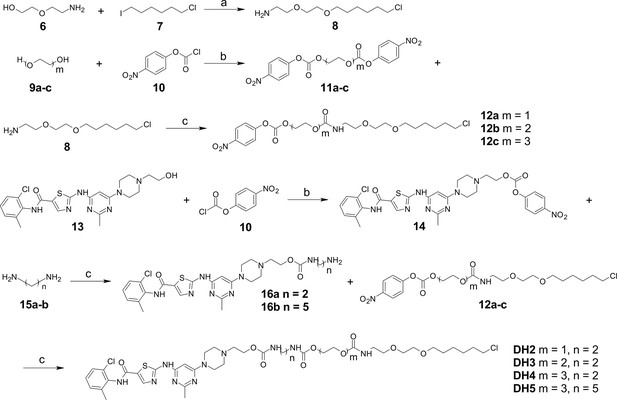
Synthesis of dasatinib-Halo derivatives 2–5 (DH2-5).
Reagents and conditions: (a) NaH, DMF, 0°C, 2 hr; (b) Et3N, DCM, RT, 12 hr; (c) DMF, RT, 2 hr. RT, room temperature.

DH5 maintained robust kinase inhibitory activity.
(A, B) SRC kinase activity was measured in vitro to assess inhibitory activities of dasatinib (A) and DH5 (B). A purified, catalytically active SRC(251-536), at a concentration of 0.8 μM, was incubated with a serial dilution of either dasatinib or DH5. The IC50 for each compound was determined from their dose-response curves. n = 3. Data are shown as mean ± s.e.m.
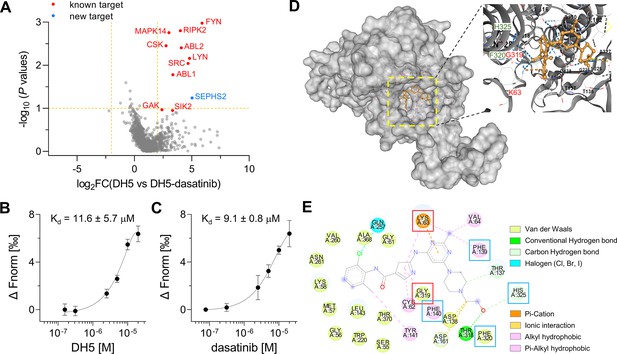
Target-ID by POST-ITDH5.
(A) Volcano plot displaying the relative fold change (FC) of DH5-binding proteins compared to those with dasatinib competition. Known target proteins for dasatinib were highlighted in red, and a newly identified target protein, SEPHS2, was marked in blue. (B, C) Microscale thermophoresis (MST) analysis demonstrates a direct interaction between DH5 and Cy5-labeled SEPHS2 (B) or between dasatinib and Cy5-labeled SEPHS2 (C), yielding Kd values of 11.6 ± 5.7 µM and 9.1 ± 0.8 µM, respectively. n = 2. Data are shown as mean ± s.d. (D) Molecular docking binding pose between dasatinib and SEPHS2. The yellow box indicates the binding pocket, expanded for a closer view. The binding pocket of dasatinib precisely fits into the active sites of SEPHS2. (E) Diagram of 2D molecular docking interaction between SEPHS2 and dasatinib. Red boxes highlight the active sites, and blue boxes indicate residues among HUB nodes.
-
Figure 5—source data 1
Proteomics result for DH5.
- https://cdn.elifesciences.org/articles/102667/elife-102667-fig5-data1-v1.xlsx
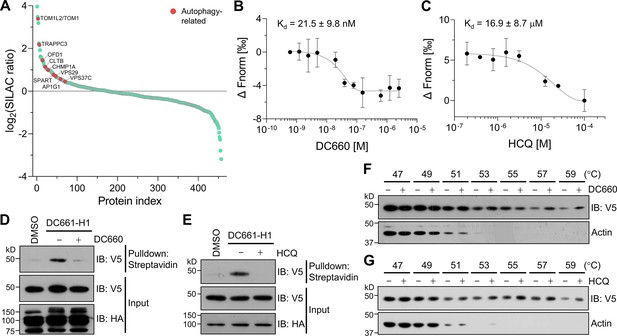
Target-ID for hydroxychloroquine (HCQ) by POST-ITDC661-H1.
(A) Rank plot analysis of SILAC ratio values of proteins in three biological replicates. Autophage-related proteins ranked highly are marked in red. Heavy-labeled cells were treated with 200 nM DC661-H1, whereas light-labeled cells underwent incubation with both DC661-H1 (200 nM) and competitive DC660 (2 µM). (B, C) Microscale thermophoresis (MST) analyses demonstrate direct interactions of DC660 (B) and HCQ (C) with VPS37C, yielding Kd values of 21.5 ± 9.8 nM and 16.9 ± 8.7 µM, respectively. n = 2. Data are shown as mean ± s.d. (D, E) Immunoblot results indicate that the VPS37C-V5 protein from cells treated with DC661-H1 was significantly enriched after streptavidin pulldown. The competition between DC661-H1 and either DC660 (D) or HCQ (E) nearly completely abolished VPS37C binding. HEK293T cells were co-transfected with HA-Halo8KR-PafAS126A,K172R, SBPK4R-sPupK61R, and VPS37C-V5. After 24 hr, cells were incubated with 200 nM of DC661-H1, with or without 2 µM of DC660 (D) or HCQ (E). Twenty-four hours later, cells were collected for further analysis. (F, G) Cellular thermal shift assay (CETSA) results demonstrate that VPS37C becomes thermostable when exposed to DC660 (F) or HCQ (G). Selective stabilization of VPS37C is evident at temperatures of 53°C or higher, a phenomenon not observed in β-actin.
-
Figure 6—source data 1
File containing labeled original western blots for Figure 6.
- https://cdn.elifesciences.org/articles/102667/elife-102667-fig6-data1-v1.zip
-
Figure 6—source data 2
Original files for western blots displayed in Figure 6.
- https://cdn.elifesciences.org/articles/102667/elife-102667-fig6-data2-v1.zip
-
Figure 6—source data 3
SILAC result for DC661-H1.
- https://cdn.elifesciences.org/articles/102667/elife-102667-fig6-data3-v1.xlsx
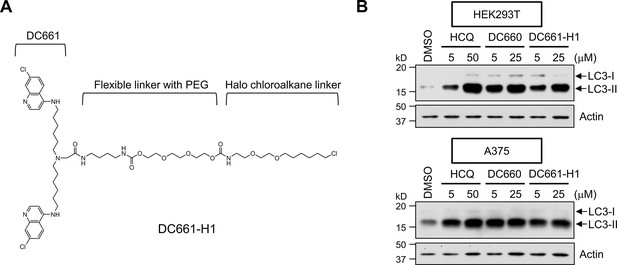
A DC661-HTL derivative, DC661-H1, is highly active as an autophagy inhibitor.
(A) The chemical structure of DC661-H1, annotated with key functional groups. (B) Autophagy inhibition by DC661-H1 was assessed through the increased levels of the LC3-II band by western blot using an anti-LC3B antibody. DC661-H1 significantly increased the levels of LC3-II in both HEK293T and A375 cells, comparably to HCQ and DC660, an analog of DC661 that lacks a methyl group at the central linker nitrogen. Cells were treated with the indicated concentration of each compound for 12 hr prior to western blot analysis.
-
Figure 6—figure supplement 1—source data 1
File containing labeled original western blots for Figure 6—figure supplement 1.
- https://cdn.elifesciences.org/articles/102667/elife-102667-fig6-figsupp1-data1-v1.zip
-
Figure 6—figure supplement 1—source data 2
Original files for western blots displayed in Figure 6—figure supplement 1.
- https://cdn.elifesciences.org/articles/102667/elife-102667-fig6-figsupp1-data2-v1.zip
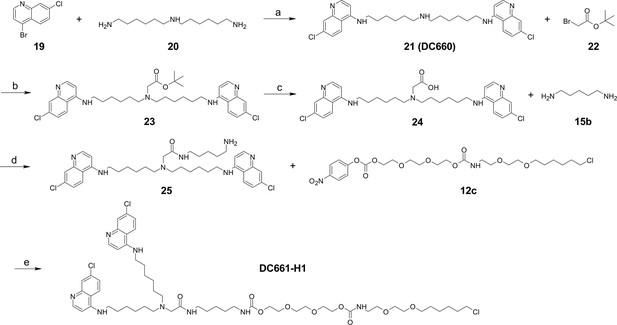
Synthesis of DC661-Halo 1 (DC661-H1).
Reagents and conditions: (a) Pd(OAc)2, BINAP, K3PO4, 1,4-dioxane, 100°C, 12 hr; (b) K2CO3, DMF, 80°C, 12 hr; (c) TFA, DCM, RT, 1 hr; (d) HATU, DIPEA, RT, 2 hr; (e) DMF, RT, 2 hr. RT, room temperature.
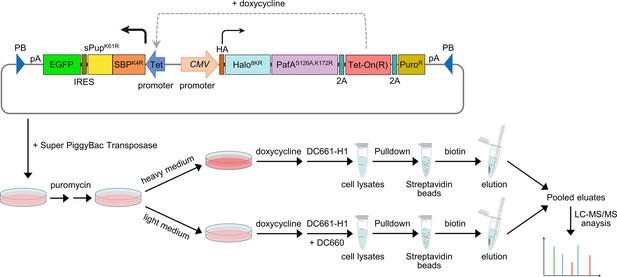
Schematic of the experimental flow for applying POST-IT in SILAC.
A PiggyBac (PB) plasmid construct containing the POST-IT system was co-transfected with the Super PiggyBac Transposase expression vector into HEK293T cells. Four days later, the cells were treated with 5 µg/ml puromycin for approximately 2 weeks. Media containing puromycin were changed every 3–4 days. Cells were divided into two groups and treated with either heavy (K8R10) or light medium (K0R0). The expression of POST-IT was induced by the addition of 100 ng/ml doxycycline. Two days later, 500 nM DC661-H1 was added to the heavy medium, or 500 nM DC661-H1 and 5 µM DC660 to the light medium. After 24 hr of incubation, cell lysates were prepared, pulled down by streptavidin magnetic beads, washed sequentially with wash buffer A and then wash buffer B, and eluted with 5 mM biotin and 10 µM DC660. The eluates were combined and precipitated using TCA/DOC, separated by Tricine-SDS-PAGE, and analyzed by LC-MS/MS. Refer to the ‘Materials and methods’ section for more details.
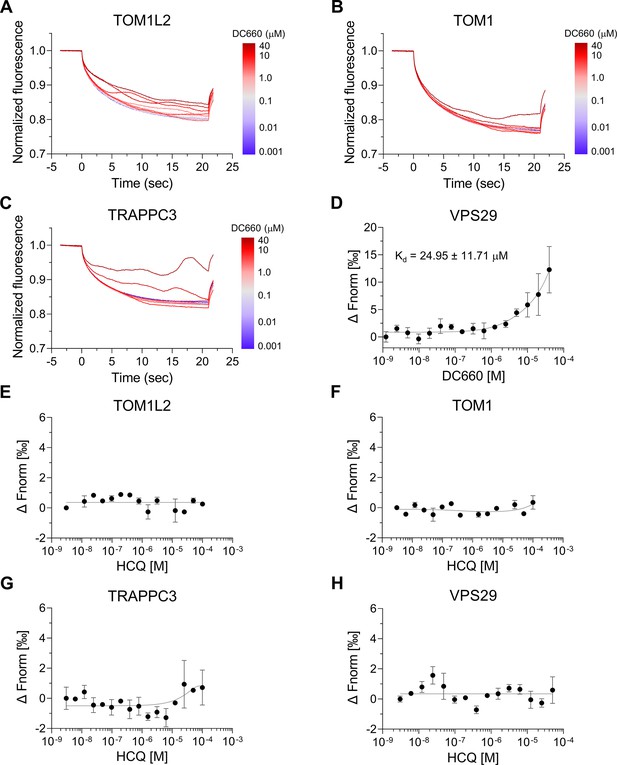
Microscale thermophoresis (MST) binding analysis of candidate proteins identified with DC661-H1.
(A–C) Thermophoretic movement of DC660 bound to purified target proteins labeled with Cy5 dye. TOM1L2, TOM1, and TRAPPC3 exhibited ligand-induced aggregation, suggesting their interaction with DC660. (D–H) Dose–response curves of normalized fluorescence changes for target proteins binding. VPS29 demonstrated substantial affinity to DC660 with a Kd of 24.95 ± 11.71 µM (D), but showed no binding to HCQ (H). TOM1L2, TOM1, and TRAPPC3 did not show detectable binding to HCQ (E–H). n = 2. Data are shown as mean ± s.d.

Molecular docking binding poses of DC661 and HCQ with the VPS37 family.
(A) Structural superposition of VPS37C with other members of the VPS37A/B/D family reveals high structural similarities within the VPS37 family. The crystal structure of the ESCRT-1 complex from yeast (2P22) is shown on the far right. STP22 and SRN2 are yeast orthologs of VPS23(TSG101) and VPS37, respectively. (B) Molecular docking binding poses of DC661 with the VPS37 family. (C) Molecular docking binding poses of HCQ with the VPS37 family.

POST-IT enables in vivo target-ID.
(A) Schematic of experimental design for applying POST-IT in zebrafish. A plasmid construct containing the POST-IT system was injected into embryos at the one-cell stage. The injected embryos were dechorionated 24 hr later, and then treated with DH5 or DMSO for an additional 24 hr. Embryos lysates were collected and analyzed by immunoblot. (B) Representative images of injected embryos. Bright-field (BF) images reveal no overt toxicity from POST-IT expression. EGFP images display robust expression of POST-IT. Scale bar, 500 µm. (C) Immunoblot analysis demonstrates that POST-IT exhibits significant enrichment of SRC in the presence of DH5 but not DMSO.
-
Figure 7—source data 1
File containing labeled original western blots for Figure 7.
- https://cdn.elifesciences.org/articles/102667/elife-102667-fig7-data1-v1.zip
-
Figure 7—source data 2
Original files for western blots displayed in Figure 7.
- https://cdn.elifesciences.org/articles/102667/elife-102667-fig7-data2-v1.zip

Expression of POST-IT does not cause toxicity in zebrafish.
(A) Quantitative analysis shows that embryos injected with POST-IT plasmid exhibit no signs of toxicity. Embryos were injected at one-cell stage, and phenotypes were assessed 24 hr later. n = 3. Data are shown as mean ± s.e.m. (B) Representative images of transgenic zebrafish embryos expressing heat-shock-inducible POST-IT show no obvious toxicity after heat shock. EGFP images confirm robust expression of POST-IT. Healthy embryos at 1 dpf were treated at 39°C for 30 min and imaged at 2 dpf. Scale bar, 500 µm. (C) Quantitative data related to (B) indicate that POST-IT expression following heat treatment does not cause toxicity. n = 3. Data are shown as mean ± s.e.m.
Tables
Identification of lysine residues for pupylation in PafA by mass spectrometry.
Protein | Score | Coverage (%) | # PSMs* | Detected pupylated sequences | MH+ (Da) | Xcorr | Lysine | Rank of pupylation |
---|---|---|---|---|---|---|---|---|
PafA | 607.055 | 89 | 1197 | |||||
1 | RIMGIETEYGLTFVDGDSKK | 2502.21808 | 5.40 | K29 | 3 | |||
2 | RIMGIETEYGLTFVDGDSKK | 2518.21299 | 5.35 | |||||
2 | IMGIETEYGLTFVDGDSKK | 2362.11188 | 3.71 | |||||
1 | IMGIETEYGLTFVDGDSKK | 2346.11697 | 3.46 | K30 | 5 | |||
1 | MFRPIVEKYSSSNIFIPNGSR | 2702.32428 | 2.93 | K47 | 5 | |||
1 | MAVDAEESLAK | 1406.64679 | 3.39 | K106 | 2 | |||
2 | MAVDAEESLAKEDIAGQVYLFK | 2670.29672 | 3.86 | |||||
4 | MAVDAEESLAKEDIAGQVYLFK | 2686.29164 | 3.86 | |||||
5 | IHHPNPLDKGESFPLGYCISQR | 2808.35222 | 5.10 | K172 | 1 | |||
3 | IHHPNPLDKGESFPLGYCISQR | 2809.33624 | 2.88 | |||||
1 | ILKAADTLGVPVTVDWMR | 2228.17436 | 3.61 | K427 | 4 | |||
1 | ILKAADTLGVPVTVDWMR | 2244.16928 | 3.49 |
-
*
PSM: peptide-spectrum match.
Reagent type (species) or resource | Designation | Source or reference | Identifiers | Additional information |
---|---|---|---|---|
Gene (Homo sapiens) | SRC | NCBI | NM_005417.5 | |
Gene (H. sapiens) | VPS37C | NCBI | NM_017966.5 | |
Gene (H. sapiens) | SEPHS2 | NCBI | NM_012248.4 | |
Strain, strain background (Danio rerio, AB) | Zebrafish | Institute of Hydrobiology, Wuhan, China | ||
Strain, strain background (Escherichia coli) | BL21(DE3) | Weidibio | Cat# EC1002 | Electrocompetent cells |
Strain, strain background (E. coli) | ArticExpress(DE3) | HaiGene | Cat# K0004 | Electrocompetent cells |
Cell line (H. sapiens) | HEK293T | ATCC | CRL-3216, RRID:CVCL_0063 | |
Cell line (H. sapiens) | A375 | ATCC | CRL-1619, RRID:CVCL_A375 | |
Antibody | Anti-LC3B (rabbit monoclonal) | ABclonal | Cat# A19665, RRID:AB_2862723 | WB (1:10,000) |
Antibody | Anti-V5-tag (rabbit monoclonal) | ABclonal | Cat# AE089 | WB (1:5000) |
Antibody | Anti-Myc-tag (rabbit monoclonal) | ABclonal | Cat# AE070, RRID:AB_2863795 | WB (1:5000) |
Antibody | Anti-HA-tag (mouse monoclonal) | ABclonal | Cat# AE008, RRID:AB_2770404 | WB (1:2500) |
Antibody | Anti-Flag-tag (rabbit monoclonal) | ABclonal | Cat# AE092, RRID:AB_2940847 | WB (1:10,000) |
Antibody | Anti-β-Actin (rabbit monoclonal) | ABclonal | Cat# AC026, RRID:AB_2768234 | WB (1:10,000) |
Antibody | Anti-Src(36D10) (rabbit monoclonal) | Cell Signaling Technology | Cat# 2109, RRID:AB_2106059 | WB (1:5000) |
Recombinant DNA reagent | pET28a-Myc-Halo-PafA | This paper | Plasmid construction (Dr. YN Jin lab) | |
Recombinant DNA reagent | pET28a-HB-Pup | This paper | Plasmid construction (Dr. YN Jin lab) | |
Recombinant DNA reagent | pET28a-SBP-sPup | This paper | Plasmid construction (Dr. YN Jin lab) | |
Recombinant DNA reagent | pET28a-TS-sPup | This paper | Plasmid construction (Dr. YN Jin lab) | |
Recombinant DNA reagent | pET28a-TS-sPup(K61R) | This paper | Plasmid construction (Dr. YN Jin lab) | |
Recombinant DNA reagent | pET28a-TS(K1,2R)-sPup(K61R) | This paper | Plasmid construction (Dr. YN Jin lab) | |
Recombinant DNA reagent | pET28a-SBP(K4R)-sPup(K61R) | This paper | Plasmid construction (Dr. YN Jin lab) | |
Recombinant DNA reagent | pET28a-sSBP-sPup(K61R) | This paper | Plasmid construction (Dr. YN Jin lab) | |
Recombinant DNA reagent | pET28a-Halo(8KR)-PafA-6xHis | This paper | Plasmid construction (Dr. YN Jin lab) | |
Recombinant DNA reagent | pET28a-mycHalo-PafA(S126A) | This paper | Plasmid construction (Dr. YN Jin lab) | |
Recombinant DNA reagent | pGEX-6P-2-PafA | This paper | Plasmid construction (Dr. YN Jin lab) | |
Recombinant DNA reagent | pGEX-6P-2-2xmycPafA(S126A)-Halo(8KR) | This paper | Plasmid construction (Dr. YN Jin lab) | |
Recombinant DNA reagent | pET28a-SRC(251-536)-2xV5 | This paper | Plasmid construction (Dr. YN Jin lab) | |
Recombinant DNA reagent | pET28a-N-SBPII-SEPHS2(U60C)-2xV5 | This paper | Plasmid construction (Dr. YN Jin lab) | |
Recombinant DNA reagent | pET28a-SAVSBPM18-XTEN-DBD | This paper | Plasmid construction (Dr. YN Jin lab) | |
recombinant DNA reagent | pTol2-3xHA-FKBP-EGFP-pA | This paper | Plasmid construction (Dr. YN Jin lab) | |
Recombinant DNA reagent | pTol2-3xV5-FRB-mKate2-PafA-2ApA-CK2 | This paper | Plasmid construction (Dr. YN Jin lab) | |
Recombinant DNA reagent | pEF6a~HB-PupE | PMID:30104635 | Plasmid construction (Dr. YN Jin lab) | |
Recombinant DNA reagent | pEF6a~SBP-sPup | This paper | Plasmid construction (Dr. YN Jin lab) | |
Recombinant DNA reagent | pEF6a~SBP(K4R)-sPup(K61R) | This paper | Plasmid construction (Dr. YN Jin lab) | |
Recombinant DNA reagent | pEF6a~sSBP-sPup(K61R) | This paper | Plasmid construction (Dr. YN Jin lab) | |
Recombinant DNA reagent | pTol2-HA-Halo-PafA-2ApA-CK2 | This paper | Plasmid construction (Dr. YN Jin lab) | |
Recombinant DNA reagent | pTol2-HA-His-Halo(8KR)-5aa-PafA-His | This paper | Plasmid construction (Dr. YN Jin lab) | |
Recombinant DNA reagent | pTol2-HA-His-Halo(8KR)-10aa-PafA-His | This paper | Plasmid construction (Dr. YN Jin lab) | |
Recombinant DNA reagent | pTol2-HA-His-Halo(8KR)-18aa-PafA-His | This paper | Plasmid construction (Dr. YN Jin lab) | |
Recombinant DNA reagent | pTol2-HA-His-Halo(8KR)-40aa-PafA-His | This paper | Plasmid construction (Dr. YN Jin lab) | |
Recombinant DNA reagent | pTol2-HA-His-Halo(8KR)-18aa-PafA(S126A)-His | This paper | Plasmid construction (Dr. YN Jin lab) | |
Recombinant DNA reagent | pTol2-HA-His-Halo(8KR)-18aa-PafA(S126A,K172R)-His | This paper | Plasmid construction (Dr. YN Jin lab) | |
Recombinant DNA reagent | pTol2-SRC(247-536)-2xV5-2ApA-CK2 | This paper | Plasmid construction (Dr. YN Jin lab) | |
Recombinant DNA reagent | pEF6a~SBP(K4R)-tbsPup(K61R) | This paper | Plasmid construction (Dr. YN Jin lab) | |
Recombinant DNA reagent | pTol2-HA-His-Halo(8KR)-tbPafA-His | This paper | Plasmid construction (Dr. YN Jin lab) | |
Recombinant DNA reagent | pTol2-HA-His-tbPafA-Halo(8KR)-His | This paper | Plasmid construction (Dr. YN Jin lab) | |
Recombinant DNA reagent | PB-Tet-Halo(8KR)-PafA(S126A,K172R)-Ter3G-SBP(K4R)-sPup(K61R)-ires-PuroR-P2A-EGFP | This paper | Plasmid construction (Dr. YN Jin lab) | |
Recombinant DNA reagent | pHAGE-ires-Puro-VPS37C-2xV5 | This paper | Plasmid construction (Dr. YN Jin lab) | |
Recombinant DNA reagent | pUbi-QFGal4-2A-POST-IT | This paper | Plasmid construction (Dr. YN Jin lab) | |
Recombinant DNA reagent | pHsp-QFGal4-2A-POST-IT | This paper | Plasmid construction (Dr. YN Jin lab) | |
Peptide, recombinant protein | RR-SRC peptide (RRLIEDAEYAARG) | Sangon | Cat# T510264-0001 | |
Commercial assay or kit | Kinase-Lumi Luminescent Kinase Assay Kit | Beyotime | Cat# S0150S | |
Chemical compound, drug | DH1 | This paper | Chemical synthesis (Dr. H-B Zhou lab) | |
Chemical compound, drug | DH2 | This paper | Chemical synthesis (Dr. H-B Zhou lab) | |
Chemical compound, drug | DH3 | This paper | Chemical synthesis (Dr. H-B Zhou lab) | |
Chemical compound, drug | DH4 | This paper | Chemical synthesis (Dr. H-B Zhou lab) | |
Chemical compound, drug | DH5 | This paper | Chemical synthesis (Dr. H-B Zhou lab) | |
Chemical compound, drug | DC661-H1 | This paper | Chemical synthesis (Dr. H-B Zhou lab) | |
Chemical compound, drug | DC660 | This paper | Chemical synthesis (Dr. H-B Zhou lab) | |
Chemical compound, drug | Hydroxychloroquine | TargetMol | Cat# T9287 | |
Chemical compound, drug | Dasatinib | Shanghai yuanye Bio-Technology | Cat# S45672-25MG | |
Chemical compound, drug | HaloTag Alexa Fluor 488 Ligand | Promega | Cat# G1001 | |
Chemical compound, drug | HaloTag Biotin Ligand | Promega | Cat# G8281 | |
Software, algorithm | Fiji | PMID:22743772 | RRID:SCR_002285 | |
Software, algorithm | Prism 9.5 | GraphPad Software | RRID:SCR_002798 | |
Software, algorithm | MaxQuant (2.1.0.0) | PMID:19029910 | RRID:SCR_14485 | |
Software, algorithm | FragPipe software v20.0 | PMID:28394336 | RRID:SCR_14485 | |
Other | Streptavindin-HRP | Cell Signaling Technology | Cat# 3999, RRID:AB_10830897 | WB (1:5000), for Halo-biotin competition assay |
Other | Dialyzed fetal bovine serum (FBS) | VivaCell | Cat# C3820-0100 | For SILAC experiment |
Other | L-Lysine∙HCl | aladdin | Cat# L113006-25g | For SILAC experiment |
Other | L-Arginine∙HCl | aladdin | Cat# 1119-34-25g | For SILAC experiment |
Other | L-Proline | aladdin | Cat# P120032-25g | For SILAC experiment |
Other | 13C615N2 labeled L-lysine∙HCl | Silantes | Cat# 211603902 | For SILAC experiment |
Other | 13C615N4 labeled L-arginine∙HCl | Silantes | Cat# 201603902 | For SILAC experiment |
Other | SILAC DMEM | Silantes | Cat# 280001200 | For SILAC experiment |