Vision: The inner workings of a miniature eye
How small can an organ get while remaining functional? Parasitic wasps from the Megaphragma genus are ideal organisms in which to investigate this question. Using a range of senses, including vision, they locate minuscule insects, known as thrips, inside which they lay their eggs (Polaszek et al., 2022). Upon hatching, the wasp larvae eat the insides of their hosts, pupate and emerge as adults from the remains, ready to repeat the cycle. This parasitic lifestyle imposes severe selective pressures; in particular, much like matryoshka dolls, the parasites generally need to be smaller than the animals they take advantage of. Since thrips are less than a millimeter long, Megaphragma wasps are minuscule, often barely reaching a fifth of a millimeter. Such extreme miniaturization requires drastic space-saving adaptations; the brain of Megaphragma viggianii wasps, for example, has fewer neurons which also lack nuclei (Polilov, 2012). Still, this reduction process can only go so far – especially for structures like the eye, which have strict size limitations due to the laws of physics and the nature of light.
Insects possess compound eyes formed of discrete, elongated units known as ommatidia. Like pixels in a camera sensor, each ommatidium samples a portion of the visual space and provides a fraction of the overall perceived image. To do so, ommatidia feature a lens that focuses and directs light onto the rhabdom, a structure stemming from photosensitive cells that convert light packets (photons) into electrical signals which are then relayed to brain neurons. To function properly, these building blocks must meet certain size requirements: in particular, the lenses must be large enough to focus an adequate amount of light from a certain direction without distortion, and the rhabdom must be sufficiently thick to guide the incoming photons.
With only 29 ommatidia, the eyes of M. viggianii wasps are one the smallest amongst animals that exhibit complex behaviors and spatial navigation (Figure 1A). Previous work has started to shed light on how their visual system can accommodate such extreme miniaturization (Chua et al., 2023). Now, in eLife, Alexey Polilov and colleagues – including Anastasia Makarova as first author – report the first complete 3D map of the compound eye of adult M. viggianii wasps with unprecedented detail (Makarova et al., 2025).
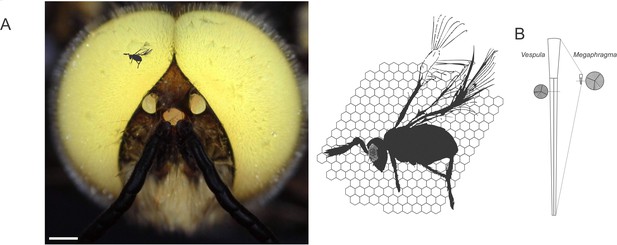
Size scaling of hymenopteran eyes, from Megaphragma viggianii to common wasps and honeybee drones.
(A) M. viggianii (black silhouette), honeybees (head of an albino drone with white eyes; left) and other bee-sized insects such as the common wasp (Vespula vulgaris) all rely on their vision to navigate their environment. Despite striking size differences, these species possess similar compound eyes consisting of individual units, or ommatidia (right; ommatidial facets of V. vulgaris represented as hexagonal shapes behind the full body of M. viggianii, whose ommatidia are depicted as small circular shapes in the eye). Scale bar, 0.5 mm. (B) The work by Makarova et al. sheds light on the organization of the eye in M. viggianii wasps. As in most insects, ommatidia in V. vulgaris (left) and M. viggianii (right) wasps are shaped like slightly tapered columns with a lens near their surface (top), and photosensitive structures (known as rhabdom; circular insets) further down inside. In M. viggianii, each ommatidium is only 20 µm long (compared to 350 µm in V. vulgaris), and the diameter of its lens reaches around 8 µm (25 µm in V. vulgaris). Yet the width of the rhabdom remains similar between the two species (1.5 µm diameter in V. vulgaris vs. 2 µm in M. viggianii).
Image credit: Honeybee drone picture by Gregor Belušič (CC BY 4.0); M. viggianii silhouette adapted from Figure 1B of Polilov, 2017 (CC BY 4.0); ommatidia silhouette adapted from Figure 4A–B of Gutiérrez et al., 2024 (CC BY 4.0).
The team, which is based at Lomonosov Moscow State University and various institutions in the United States, exploited one of the advantages conferred by the small size of the insect; namely, that its entire body could be sectioned and imaged via electron microscopy at once (Polilov, 2017). Imaging revealed that the eye is formed of 478 cells, with a single ommatidium bringing together nine photosensitive cells, four cone cells (that focus light), and two primary pigment cells containing granules full of opaque compounds.
Further analyses showed that the diameter of the lens in an ommatidium is only 8 µm at most, which is hardly sufficient to focus light onto photoreceptors without distortion. However, the underlying rhabdom has retained a relatively broad cross-section, 2 µm, which is similar to that found in large diurnal insects (Figure 1B). Together, the lens and rhabdom are therefore able to efficiently capture enough light for the eyes to perform during the day. In addition, the sides of the ommatidia are coated with very dense layers of pigment granules that optically isolate the rhabdoms, blocking stray light and therefore contributing to image formation.
Examining cell structure revealed that, unlike neurons in the brain of M. viggianii, eye cells have nuclei; probably due to optical constraints, these cells remained large enough to escape extreme miniaturization and retain an essential organelle lost elsewhere in the visual pathway. Finally, Makarova et al. show that photoreceptor cells are packed with mitochondria, suggesting that vision is metabolically costly to the wasps.
In many insects, an eye region known as the dorsal rim area is part of a visual system used to analyze polarized light from the sky, which serves as a stable spatial reference when animals travel in arbitrary directions or across longer distances (Labhart and Meyer, 1999). Surprisingly, in M. viggiani wasps, about a third of the available ommatidia, located dorsally, show specializations for the detection of polarized light; this includes a special rhabdom geometry, smaller optical parts and even cell nuclei inserted into the optical pathway that may play an optical role, similar to eye structures facilitating polarized light navigation in honeybees (Meyer and Labhart, 1981). The compound eye thus shows a remarkable resource allocation, with ~30% dedicated to navigation and ~60% to image formation. Lastly, the analysis revealed the presence of three photoreceptors disconnected from the eye optics behind the first ommatidia in the dorsal margin; these structures possibly contribute to other biological processes requiring light detection, such as the regulation of circadian rhythms.
Overall, the work by Makarova et al. represents a valuable quantitative morphological reference for the future studies of compound eyes and miniaturized organs in visual physiology and cell biology. It will inform the design of miniaturized imaging sensors, enable the numerical modelling of small compound eyes, and support the framework for the understanding of cellular architecture in eukaryotic organisms.
References
-
A complete reconstruction of the early visual system of an adult insectCurrent Biology 33:4611–4623.https://doi.org/10.1016/j.cub.2023.09.021
-
Spatial resolution and optical sensitivity in the compound eyes of two common European wasps, Vespula germanica and Vespula vulgarisThe Journal of Experimental Biology 227:jeb246670.https://doi.org/10.1242/jeb.246670
-
Pore canals in the cornea of a functionally specialized area of the honey bee’s compound eyeCell and Tissue Research 216:491–501.https://doi.org/10.1007/BF00238646
-
The smallest insects evolve anucleate neuronsArthropod Structure & Development 41:29–34.https://doi.org/10.1016/j.asd.2011.09.001
Article and author information
Author details
Publication history
Copyright
© 2025, Belušič
This article is distributed under the terms of the Creative Commons Attribution License, which permits unrestricted use and redistribution provided that the original author and source are credited.
Metrics
-
- 245
- views
-
- 22
- downloads
-
- 0
- citations
Views, downloads and citations are aggregated across all versions of this paper published by eLife.
Download links
Downloads (link to download the article as PDF)
Open citations (links to open the citations from this article in various online reference manager services)
Cite this article (links to download the citations from this article in formats compatible with various reference manager tools)
Further reading
-
- Evolutionary Biology
- Genetics and Genomics
Expression quantitative trait loci (eQTLs) provide a key bridge between noncoding DNA sequence variants and organismal traits. The effects of eQTLs can differ among tissues, cell types, and cellular states, but these differences are obscured by gene expression measurements in bulk populations. We developed a one-pot approach to map eQTLs in Saccharomyces cerevisiae by single-cell RNA sequencing (scRNA-seq) and applied it to over 100,000 single cells from three crosses. We used scRNA-seq data to genotype each cell, measure gene expression, and classify the cells by cell-cycle stage. We mapped thousands of local and distant eQTLs and identified interactions between eQTL effects and cell-cycle stages. We took advantage of single-cell expression information to identify hundreds of genes with allele-specific effects on expression noise. We used cell-cycle stage classification to map 20 loci that influence cell-cycle progression. One of these loci influenced the expression of genes involved in the mating response. We showed that the effects of this locus arise from a common variant (W82R) in the gene GPA1, which encodes a signaling protein that negatively regulates the mating pathway. The 82R allele increases mating efficiency at the cost of slower cell-cycle progression and is associated with a higher rate of outcrossing in nature. Our results provide a more granular picture of the effects of genetic variants on gene expression and downstream traits.
-
- Ecology
- Evolutionary Biology
While host phenotypic manipulation by parasites is a widespread phenomenon, whether tumors, which can be likened to parasite entities, can also manipulate their hosts is not known. Theory predicts that this should nevertheless be the case, especially when tumors (neoplasms) are transmissible. We explored this hypothesis in a cnidarian Hydra model system, in which spontaneous tumors can occur in the lab, and lineages in which such neoplastic cells are vertically transmitted (through host budding) have been maintained for over 15 years. Remarkably, the hydras with long-term transmissible tumors show an unexpected increase in the number of their tentacles, allowing for the possibility that these neoplastic cells can manipulate the host. By experimentally transplanting healthy as well as neoplastic tissues derived from both recent and long-term transmissible tumors, we found that only the long-term transmissible tumors were able to trigger the growth of additional tentacles. Also, supernumerary tentacles, by permitting higher foraging efficiency for the host, were associated with an increased budding rate, thereby favoring the vertical transmission of tumors. To our knowledge, this is the first evidence that, like true parasites, transmissible tumors can evolve strategies to manipulate the phenotype of their host.