Mitochondrial CoQ deficiency is a common driver of mitochondrial oxidants and insulin resistance
Figures
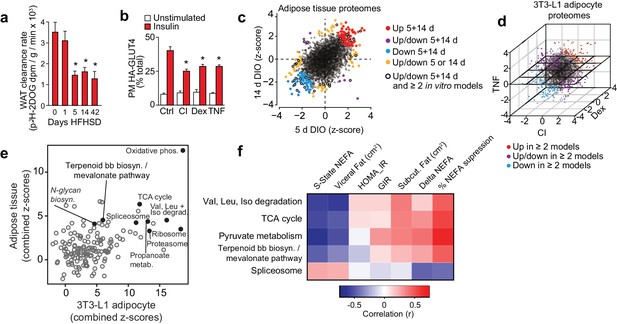
Proteomic analysis of adipocyte insulin resistance.
(A) Adipose tissue 3H-2-DOG uptake during a glucose tolerance test in mice fed a high fat high sucrose diet (HFHSD) for indicated times. Results show mean ±S.E.M. of eight mice. *p<0.05 versus mice fed a HFHSD for 0 days, t-tests corrected for multiple comparisons. (B) 3T3-L1 adipocytes were treated with chronic insulin (CI), dexamethasone (Dex) or tumour necrosis factor-α (TNF) to induce insulin resistance and stimulated with 100 nM insulin for 20 min where indicated before determination of insulin sensitivity by HA-GLUT4 abundance at the plasma membrane (PM). Results show mean ±S.E.M. of six separate experiments. *p<0.05 versus insulin-stimulated control cells, t-tests corrected for multiple comparisons. (C) Scatter plot of z-scores of protein changes (p<0.01) in adipose tissue from mice fed a HFHSD for 5 or 14 d (red = proteins consistently up-regulated, blue = proteins consistently down-regulated, purple = proteins with mixed response (up/down), orange = proteins altered at a single time point, black open circle = changed at both HFHSD time points and at least two in vitro models). (D) Three-dimensional direction analysis for proteomic data across the three 3T3-L1 adipocyte models of insulin resistance (coloured proteins = p < 0.01). Axes correspond to the z-scores of protein changes and coloured points indicate proteins changed in at least two out of three models (red = proteins up-regulated, blue = proteins down-regulated, purple = proteins with mixed response (up/down) across models). (E) Scatter plot of z-scores for pathways from proteomic analysis of insulin-resistant tissue (y-axis) or cells (x-axis). Selected pathways with z-scores >4 in tissue and cells are highlighted. (F) Heat map of correlations between expression of proteins within selected KEGG pathways and clinical measures from a cohort of obese subjects. Pathways of interest from (E) with significant (>0.423 or <−0.423) correlation with % suppression of circulating non-esterified fatty acids (NEFA) during a hyperinsulinaemic-euglycaemic clamp are shown (S-State NEFA, steady-state non-esterified free fatty acids during hyperinsulinaemic-euglycaemic clamp; HOMA_IR, Homeostatic model assessment of insulin resistance; GIR, glucose infusion rate during a hyperinsulinaemic-euglycaemic clamp; Delta NEFA, change in circulating NEFA concentrations between baseline and during a hyperinsulinaemic-euglycaemic clamp; % suppression NEFA, percentage suppression of NEFAs between baseline and during a hyperinsulinaemic-euglycaemic clamp; Val, valine; Leu, leucine; Iso, isoleucine; TCA, tricarboxylic acid cycle; Terpenoid bb biosyn., Terpenoid backbone biosynthesis). See also Figure 1—figure supplement 1.
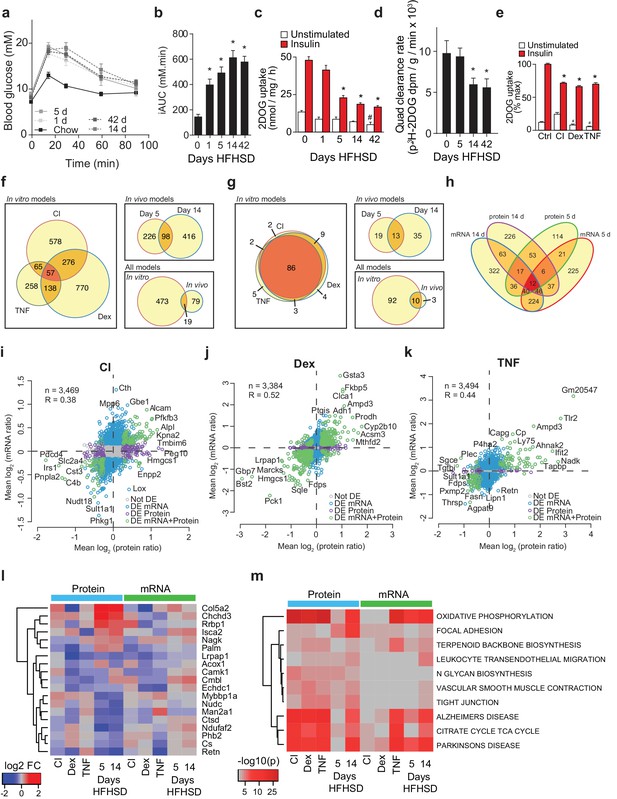
Comparision of transcriptomic and proteomic changes in insulin-resistant adipocytes.
(A) Blood glucose concentrations during a glucose tolerance test (GTT) in mice fed a HFHSD for indicated times. (B) Incremental AUC (iAUC) for blood glucose concentrations during a GTT in Figure 1—figure supplement 1A. Results show mean ±S.E.M. of nine to fifteen mice. *p<0.05 versus mice fed a HSHFD for 0 d, t-tests corrected for multiple comparisons. (C) Insulin-regulated 3H-2-DOG uptake was assessed in adipose tissue explants from mice fed a HFHSD for indicated times and stimulated with 10 nM insulin where indicated. Results show mean ±S.E.M. of five mice. #p<0.05 versus unstimulated explants from mice fed a HFHSD for 0 days, *p<0.05 versus insulin-stimulated explants from mice fed a HFHSD for 0 days, t-tests corrected for multiple comparisons. (D) Quadriceps 3H-2-DOG uptake during a glucose tolerance test in mice fed a HFHSD for indicated times. Results show mean ±S.E.M. of nine to fifteen mice. *p<0.05 versus mice fed a HSHFD for 0 d, t-tests corrected for multiple comparisons. (E) 3T3-L1 adipocytes were treated with chronic insulin (CI), dexamethasone (Dex) or tumour necrosis factor-α (TNF) as described in the Materials and Methods and stimulated with 100 nM insulin for 20 min where indicated before determination of insulin sensitivity by 3H-2-DOG uptake. Results show mean ±S.E.M. of six separate experiments. #p<0.05 versus unstimulated control cells *p<0.05 versus insulin-stimulated control cells, t-tests corrected for multiple comparisons. (F–H) Venn diagrams depicting overlap between differentially expressed proteins between models of insulin resistance (F), differentially expressed pathways between models of insulin resistance (G) and between differentially expressed transcripts and proteins in insulin-resistant adipose tissue from mice fed a HFHSD for 5 or 14 d (H). (I–K) Log2 fold change relative to controls for transcripts plotted against log2 fold change data for protein from CI (I), Dex (J) and TNF (K) models. Differential expression at adj. p<0.05. Correlation between transcript and proteins indicated by correlation coefficient, r. (Grey = not differentially expressed at mRNA and protein level, blue = differentially expressed mRNA only, purple = differentially expressed protein only, green = differentially expressed mRNA and protein). Statistical analyses described in Materials and Methods. (L) Heat map of log2 fold change data for mRNA and protein data for 19 proteins found to be regulated in all models (Figure 1—figure supplement 1F, All Models). (M) Heat map of GSEA-derived p values for 10 pathways found to be regulated in all models (Figure 1—figure supplement 1G, All Models).
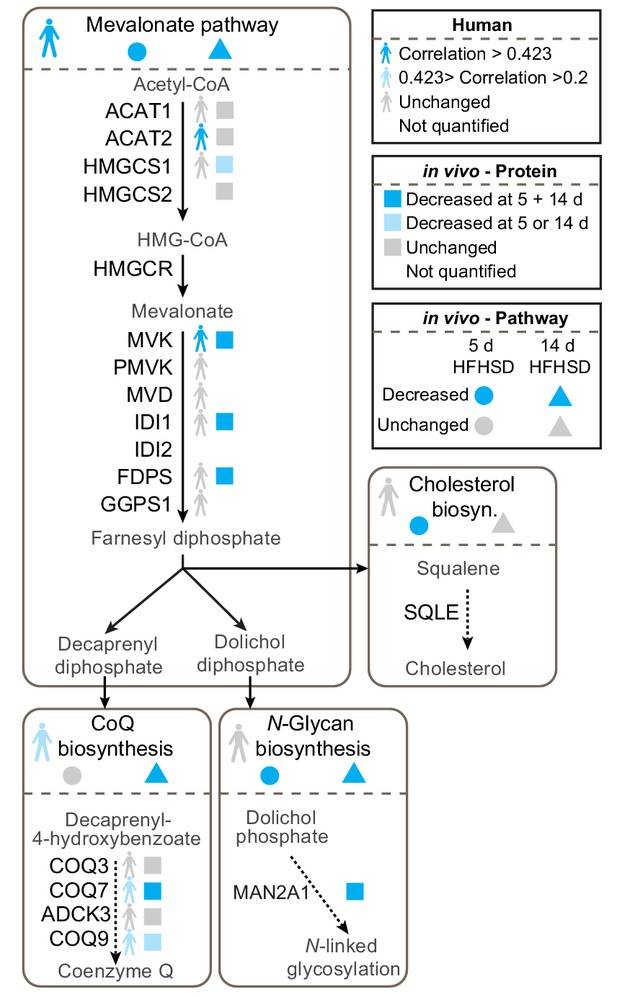
Proteomic data for the mevalonate pathway and downstream pathways in insulin-resistant adipose tissue.
Human figures represent expression of pathways or proteins in human data (Supplementary file 3). Circles and triangles represent gene set enrichment pathway analyses for proteomic analysis of tissue from mice fed a HFHSD for 5 (circle) or 14 d (triangle) as indicated in the figure legend (significance = adj. p<0.05). Squares represent individual protein data (adj. p<0.01). Statistical analyses described in Materials and Methods. All proteins in mevalonate/terpenoid backbone biosynthesis pathway are depicted along with selected proteins from CoQ biosynthesis, N-glycan biosynthesis and cholesterol/steroid biosynthesis. Colours indicate direction of change as in legend. See also Figure 2—figure supplement 1.
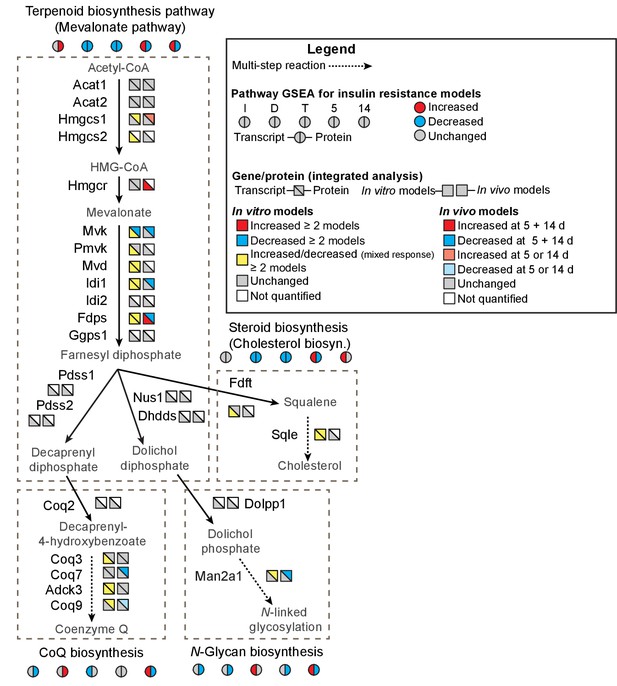
Pathway and single gene/protein data for the mevalonate pathway and downstream pathways in insulin-resistant adipose tissue and in vitro models of insulin resistance.
Circles represent gene set enrichment pathway analyses (significance = p < 0.05). Each circle represents a different model of insulin resistance, ordered as indicated in the legend. Left squares represent integrated single gene/protein data from directional analysis for in vitro models, and right square for in vivo models (3T3-L1 transcript p<0.001, 3T3-L1 protein p<0.01, adipose tissue transcript p<0.001, adipose tissue protein p<0.01). Statistical analyses described in Methods. All proteins in mevalonate/terpenoid backbone biosynthesis pathway depicted along with selected proteins from CoQ biosynthesis, N-glycan biosynthesis and cholesterol/steroid biosynthesis. Colours indicate direction of change as in legend.
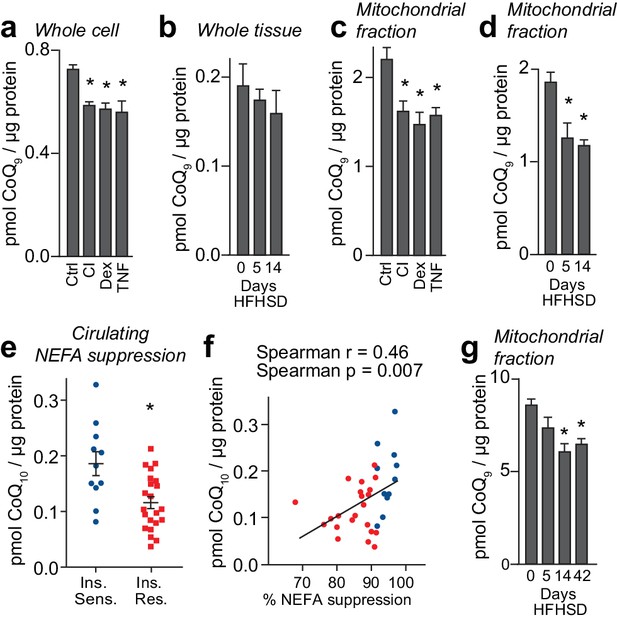
Loss of mitochondrial coenzyme Q in insulin-resistant adipose and muscle tissue.
(A) Concentrations of CoQ9 in control (Ctrl) 3T3-L1 adipocytes, and adipocytes treated to induce insulin resistance with chronic insulin treatment (CI), dexamethasone (Dex) or TNFα (TNF). (B) Total adipose tissue concentrations of CoQ9 were determined in mice fed a HFHSD for 0, 5 and 14 d. (C) Concentrations of CoQ9 in the mitochondria of 3T3-L1 adipocytes treated as specified. (D) Concentrations of CoQ9 in mitochondria of adipose tissue from mice fed a HFHSD for indicated times. (A–D) Results show mean ±S.E.M of three to five separate in vitro studies and six in vivo studies. *p<0.05 versus control samples, t-tests corrected for multiple comparisons. (E) Obese participants were stratified into insulin-sensitive (upper tertile, 11 subjects) and insulin-resistant (lower two tertiles, 22 subjects) groups based on the % reduction in circulating NEFAs during a hyperinsulinaemic-euglycaemic clamp. Mean adipose mitochondrial CoQ10 concentrations were calculated for each group, error bars are S.E.M. (Mann-Whitney, *p<0.05). (F) Correlation between rate of reduction (%) in circulating NEFA and adipose mitochondrial CoQ10 concentrations for human subjects in Figure 3E. Colours correspond to colours in Figure 3E. (G) Concentrations of CoQ9 in mitochondria of skeletal muscle (quadriceps) from mice fed a HFHSD for indicated times. Results show mean ±S.E.M of four to six in vivo studies. *p<0.05 versus control samples, t-tests corrected for multiple comparisons. See also Figure 3—figure supplement 1.
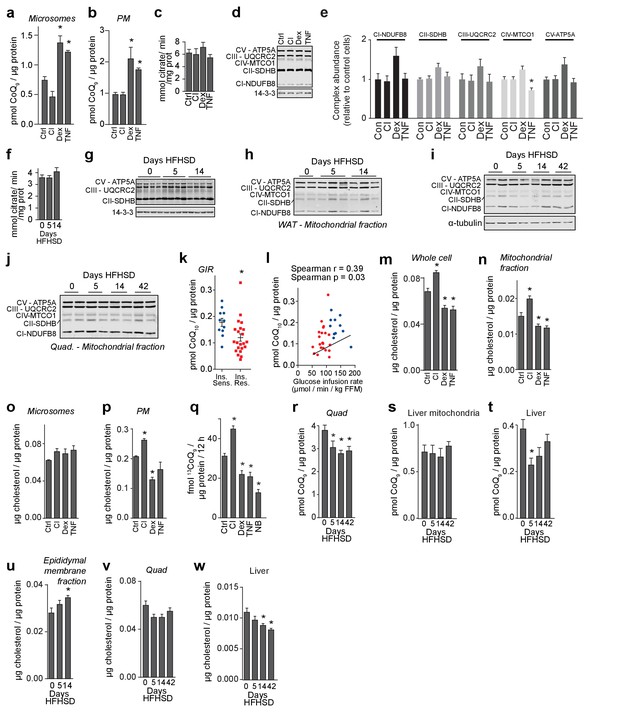
CoQ, cholesterol and mitochondria content in in vitro and in vivo models of insulin resistance.
(A–B) Concentrations of CoQ9 in the microsomal fraction (A) and plasma membrane (B) of 3T3-L1 adipocytes treated as specified. (C) Citrate synthase activity in lysates of 3T3-L1 adipocytes treated as indicated. (A–C) Results show mean ±S.E.M. of five to eight separate experiments. *p<0.05 versus control cells, t-tests corrected for multiple comparisons. (D) Immunoblot analysis of specific subunits of the mitochondrial respiratory complexes in in vitro models of insulin resistance. (E) Densitometric analysis of Figure 3—figure supplement 1D. (D–E) Results show mean ±S.E.M. of five to eight separate experiments. *p<0.05 versus control cells, t-tests corrected for multiple comparisons. (F) Citrate synthase activity in lysates of adipocyte tissue from mice fed a HFHSD diet for indicated times. Results show mean ±S.E.M. of six separate experiments. (G–J) Immunoblot analysis of specific subunits of the mitochondrial respiratory complexes in whole adipose (G) or skeletal muscle (I) tissue or in mitochondrial fractions from adipose (H) or quadriceps skeletal muscle (Quad) (J) tissue from mice fed a HFHSD for indicated times. Blots are representative of at least three separate experiments. (K) Obese participants were stratified into insulin-sensitive (upper tertile, 11 subjects) and insulin-resistant (lower two tertiles, 22 subjects) groups based on glucose infusion rate (GIR) during a hyperinsulinaemic-euglycaemic clamp. Mean adipose mitochondrial CoQ10 levels were calculated for each group (Mann-Whitney, *p=0.05). (L) Correlation between patient glucose infusion rate and adipose mitochondrial CoQ10 concentrations. Colours correspond to colours in Figure 3—figure supplement 1K. (M–P) Concentrations of non-esterified cholesterol in the whole cell homogenates (M), mitochondria (N), microsomal fraction (O) and plasma membrane (P) of 3T3-L1 adipocytes treated as specified. Results show mean ±S.E.M of three to five separate in vitro studies. *p<0.05 versus control samples, t-tests corrected for multiple comparisons. (Q) 13C6-CoQ9 concentrations in 3T3-L1 adipocyte models of insulin resistance or in 3T3-L1 adipocytes treated with the inhibitor of CoQ biosynthesis 4-nitrobezoic acid (NB) incubated with 13C-4-hydroxybenzoic acid for 12 hr. Results show mean ±S.E.M of four separate studies. *p<0.05 versus control samples, t-tests corrected for multiple comparisons. (R–T) Concentrations of CoQ9 in whole muscle (R) or liver (T) tissue or mitochondria from liver (S) of mice fed a HFHSD for 0, 5, 14 and 42 d. (U) Concentrations of non-esterified cholesterol in total cell membrane fraction obtained from epididymal adipose tissue from mice fed a HFHSD for 0, 5 and 14 d. (V–W) Concentrations of non-esterified cholesterol in skeletal muscle (V) or liver (W) from mice fed a HFHSD for 0, 5, 14 and 42 d. For R-W, results show mean ±S.E.M of four to six mice. *p<0.05 versus control samples, t-tests corrected for multiple comparisons.
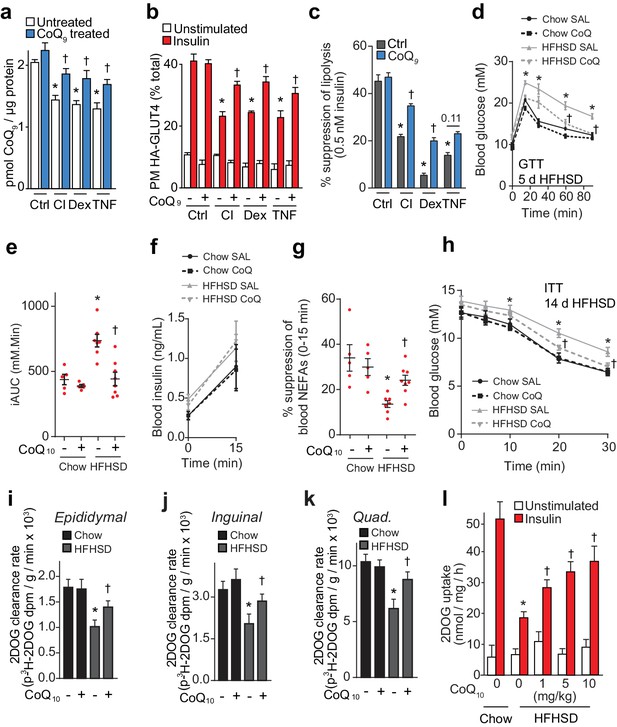
CoQ supplementation improves insulin sensitivity in vitro and in vivo models of insulin resistance.
(A) Concentrations of CoQ9 in mitochondria of control (Ctrl) and insulin-resistant 3T3-L1 adipocyte models supplemented with 10 µM CoQ9 as indicated. Results show mean ±S.E.M. of three to four independent experiments. *p<0.05 versus control cells, †p<0.05 versus respective cells without CoQ supplementation, t-tests corrected for multiple comparisons. (B–C) 3T3-L1 adipocytes were treated to induce insulin resistance with and without 10 µM CoQ9 supplementation as indicated before determination of insulin sensitivity by HA-GLUT4 abundance at the plasma membrane (PM) in unstimulated cells and in response to 100 nM insulin (B) or by suppression of lipolysis by 0.5 nM insulin (C; calculated from Figure 4—figure supplement 1C–E as described in the Materials and Methods). Results show mean ±S.E.M. of three to four independent experiments. *p<0.05 versus insulin-stimulated control cells, †p<0.05 versus respective insulin-stimulated cells without CoQ supplementation, t-tests corrected for multiple comparisons. (D) Blood glucose concentrations during a glucose tolerance test (GTT) in mice treated as indicated (5 d HFHSD; saline (SAL) or 10 mg/kg CoQ10). Results show mean ±S.E.M. of five to nine mice. *p<0.05 versus chow-fed saline treated mice at the same time-point, †p<0.05 versus HFHSD-fed saline-treated mice at the same time point, t-tests corrected for multiple comparisons. (E) Incremental area under the curve (iAUC) for blood concentrations during GTT in Figure 4D. *p<0.05 versus chow-fed saline treated mice, †p<0.05 versus HFHSD-fed saline-treated mice, t-tests corrected for multiple comparisons. (F) Blood insulin concentrations at 0 and 15 min of the GTT in Figure 4D. (G) Suppression of circulating non-esterified fatty acids (NEFAs) between 0 and 15 min of the GTT in Figure 4D. *p<0.05 versus chow-fed saline treated mice, †p<0.05 versus HFHSD-fed saline-treated mice, t-tests corrected for multiple comparisons. (H) Blood glucose concentrations during an ITT in mice treated as indicated (14 d HFHSD; 10 mg/kg CoQ10). Results show mean ±S.E.M. of five to nine mice. *p<0.05 versus chow-fed saline treated mice at the same time-point, †p<0.05 versus HFHSD-fed saline-treated mice at the same time point, t-tests corrected for multiple comparisons. (I–K) Epididymal (I) and inguinal (J) adipose tissue and quadriceps (K) 3H-2-DOG uptake during the ITT in Figure 4H. Results show mean ±S.E.M. of five to nine mice. *p<0.05 versus chow-fed saline treated mice at the same time-point, †p<0.05 versus HFHSD-fed saline-treated mice at the same time point, t-tests corrected for multiple comparisons. (L) Adipose tissue explants from mice fed a chow diet or a HFHSD for 14 d and supplemented with CoQ10 at specified doses were stimulated with 10 nM insulin where indicated and 2-DOG uptake was assessed. Results show mean ±S.E.M. of five mice. *p<0.05 versus insulin-stimulated explants from mice fed a chow diet and †p<0.05 versus insulin-stimulated explants from HFHSD-fed mice without CoQ supplementation, t-tests corrected for multiple comparisons. See also Figure 4—figure supplement 1.
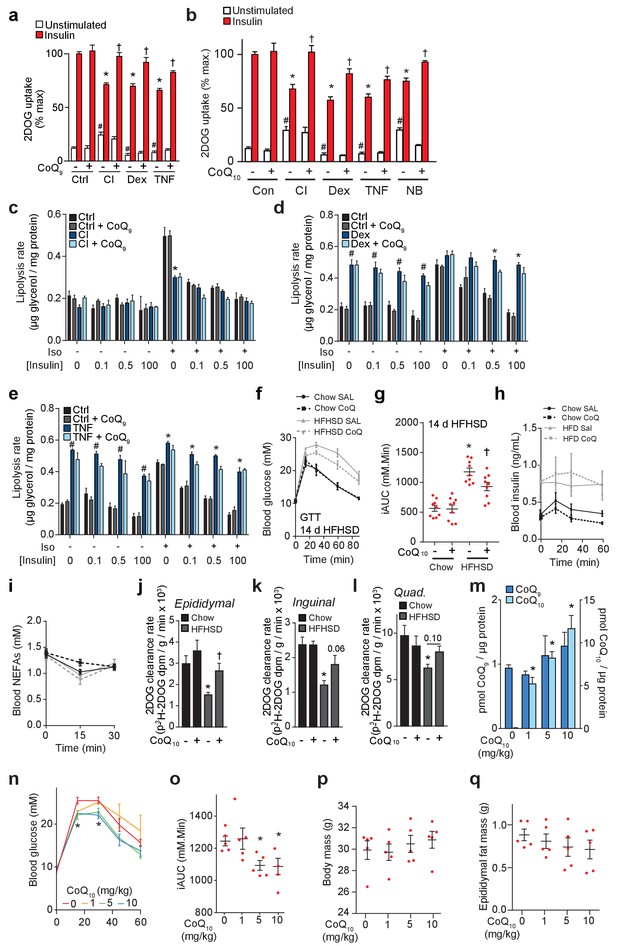
Addtional data on the effect of CoQ supplementation on insulin sensitivity in models of insulin resistance
(A) 3T3-L1 adipocytes were treated to induce insulin resistance with and without 10 µM CoQ9 supplementation as indicated before determination of insulin sensitivity by 3H-2-DOG uptake in unstimulated and insulin-stimulated cells. (B) 3T3-L1 adipocytes were treated to induce insulin resistance in the presence and absence of 10 µM CoQ10 as indicated before determination of insulin sensitivity by 3H-2-DOG uptake in non-stimulated and insulin-stimulated cells. Results show mean ±S.E.M. for three to four in vitro studies. #p<0.05 versus unstimulated control cells, *p<0.05 versus insulin-stimulated control cells, †p<0.05 versus insulin-resistant model without CoQ supplementation, t-tests corrected for multiple comparisons. (C–E) 3T3-L1 adipocytes were treated to induce insulin resistance with and without 10 µM CoQ9 supplementation as indicated before insulin sensitivity was determined by inhibition of glycerol release as a measure of lipolysis. Lipolysis was stimulated with 1 nM isoproterenol. Results show mean ±S.E.M. for three separate studies. #p<0.05 versus control cells without isoproterenol, *p<0.05 versus isoproterenol-stimulated control cells, t-tests corrected for multiple comparisons. (F) Blood glucose concentrations during a GTT in mice treated as indicated (14 d HFHSD; 10 mg/kg CoQ10). (G) Incremental area under the curve (iAUC) for blood concentrations during GTT in Figure 4—figure supplement 1F. (H) Blood insulin concentrations during GTT in Figure 4—figure supplement 1G. (I) Blood NEFA concentrations during the first 30 min of the GTT in Figure 4D. (J–L) Epididymal (J) and inguinal (K) adipose tissue and quadriceps (L) 3H-2-DOG uptake during the glucose tolerance test in Figure 4—figure supplement 1F. Results show mean ±S.E.M. of nine mice. *p<0.05 versus mice fed a chow diet, †p<0.05 versus HFHSD-fed mice without CoQ supplementation, t-tests corrected for multiple comparisons. (M) Concentrations of CoQ9 and CoQ10 in mitochondria isolated from adipose tissue from mice fed a HFHSD for 14 d and supplemented with CoQ10 at specified dose. Results show mean ±S.E.M. of five mice. *p<0.05 versus mice without CoQ supplementation, t-tests corrected for multiple comparisons. (N) Blood glucose levels during a GTT in mice fed a HFHSD for 14 d and supplemented with stated dose of CoQ10. Results show mean ±S.E.M. of five mice. *p<0.05 versus HFHSD-fed mice without CoQ supplementation, t-tests corrected for multiple comparisons. (O) iAUC for blood glucose concentrations during a GTT in Figure 4—figure supplement 1N. Results show mean ±S.E.M. of five mice. *p<0.05 versus mice without CoQ10 supplementation, t-tests corrected for multiple comparisons. (P–Q) Body mass (P) and epididymal fat pad mass (Q) for mice fed a HFHSD for 14 d and supplemented with CoQ10 at specified dose. Results show mean ±S.E.M. of five mice.
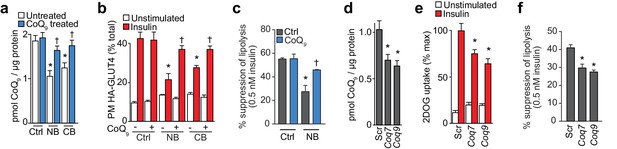
Inhibition of CoQ synthesis is sufficient for insulin resistance in vitro.
(A) Concentrations of CoQ9 in mitochondria of control (Ctrl) 3T3-L1 adipocytes and adipocytes treated with 2.5 mM 4-nitrobenzoic (NB) or 4-chlorobenzoic acid (CB) with or without 10 µM CoQ9. Results show mean ±S.E.M. for five-six separate in vitro experiments. *p<0.05 versus control cells, †p<0.05 versus respective model without CoQ supplementation, t-tests corrected for multiple comparisons. (B–C) 3T3-L1 adipocytes were treated with NB or CB before sensitivity was determined by HA-GLUT4 abundance at the plasma membrane (PM) before and after stimulation with 100 nM insulin (B) or suppression of lipolysis by 0.5 nM insulin (Figure 5—figure supplement 1B as described in the Materials and Methods). Results show mean ±S.E.M. for four separate in vitro experiments. *p<0.05 versus insulin-stimulated control cells, †p<0.05 versus respective insulin-stimulated cells without CoQ supplementation, t-tests corrected for multiple comparisons. (D) Concentrations of CoQ9 in mitochondria of 3T3-L1 adipocytes treated with control scrambled (Scr) siRNA, or pooled siRNA targeted to Coq7 or Coq9. Results show mean ±S.E.M. for four separate in vitro experiments. *p<0.05 versus control cells, t-tests corrected for multiple comparisons. (E–F) Insulin sensitivity of 3T3-L1 adipocytes treated with Scr or siRNA targeted to Coq7 or Coq9 as measured by 2DOG uptake (E) or suppression of lipolysis by 0.5 nM insulin (F; calculated from Figure 5—figure supplement 1E as described in the Materials and Methods). Results show mean ±S.E.M. for four separate in vitro experiments. *p<0.05 versus insulin-stimulated control cells, t-tests corrected for multiple comparisons. See also Figure 5—figure supplement 1.
; calculated from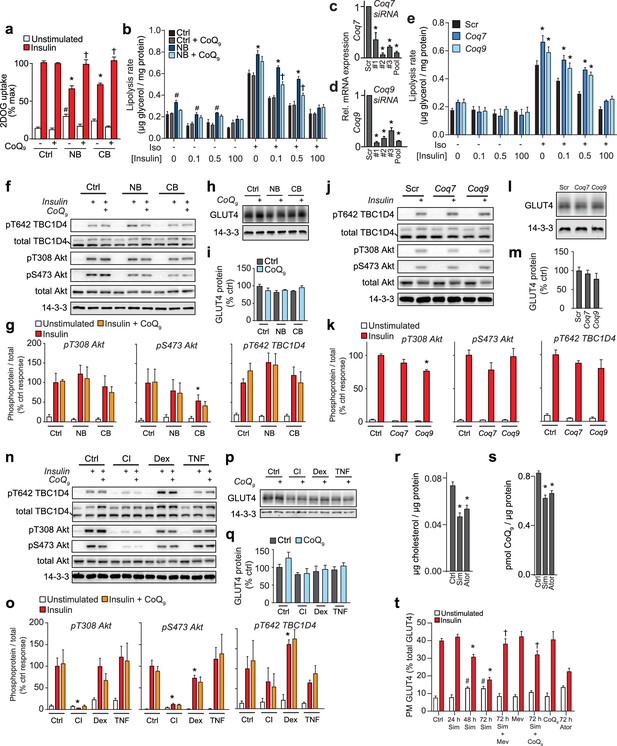
Loss of CoQ causes insulin resistance independently of consistent defects in insulin signalling or changes in GLUT4 expression.
(A) 3T3-L1 adipocytes were treated with nitrobenzoic acid (NB) or chlorobenzoic acid (CB) and/or CoQ9 before sensitivity was determined by 3H-2-DOG uptake. Results show mean ±S.E.M. for four separate studies. #p<0.05 versus unstimulated control cells, *p<0.05 versus insulin-stimulated control cells, †p<0.05 versus respective insulin-stimulated cells without CoQ supplementation, t-tests corrected for multiple comparisons. (B) 3T3-L1 adipocytes were treated with NB and/or CoQ9 before insulin sensitivity was determined by inhibition of glycerol release as a measure of lipolysis. Lipolysis was stimulated with 1 nM isoproterenol. Results show mean ±S.E.M. for three separate studies. #p<0.05 versus control cells without isoproterenol, *p<0.05 versus isoproterenol -stimulated control cells, †p<0.05 versus isoproterenol- and insulin-stimulated NB-treated cells without CoQ supplementation, t-tests corrected for multiple comparisons. (C–D) 3T3-L1 adipocytes treated with scrambled (Scr) or a panel of three different separate or pooled siRNAs against either anti-Coq7 (C) or anti-Coq9 (D) and mRNA levels of target genes were analysed by qPCR to determine the extent of knockdown. Results show mean ±S.E.M. for three separate studies. *p<0.05 versus control cells, t-tests corrected for multiple comparisons. (E) 3T3-L1 adipocytes were treated with scrambled siRNA or pooled siRNA to Coq7 or Coq9 before insulin sensitivity was determined by inhibition of glycerol release as a measure of lipolysis. Lipolysis was stimulated with 1 nM isoproterenol. Results show mean ±S.E.M. for three separate studies. #p<0.05 versus control cells without isoproterenol, *p<0.05 versus isoproterenol -stimulated control cells, t-tests corrected for multiple comparisons. (F–Q) Insulin signalling responses and GLUT4 expression was determined by imunnoblot and densitometry in 3T3-L1 adipocytes treated with pharmacological inhibitors of CoQ biosynthesis with and without CoQ9 supplementation (F–I), siRNA to Coq7 or Coq9 (J–M) or with treatments to induce insulin resistance with and with CoQ9 supplementation (N–Q). Results show mean ±S.E.M. of three independent experiments. *p<0.05 versus insulin-stimulated control cells, t-tests corrected for multiple comparisons. (R–S) Concentrations of non-esterified cholesterol (R) and CoQ9 (S) in 3T3-L1 adipocytes treated with 10 µM simvastatin (Sim) or 20 µM atorvastatin (Ator) for 72 hr. Results show mean ±S.E.M. for four separate studies. *p<0.05 versus control cells, t-tests corrected for multiple comparisons. (T) 3T3-L1 adipocytes were treated with 10 µM simvastatin (Sim) or 20 µM atorvastatin (Ator) for indicated times with and without 100 µM mevalonate or 10 µM CoQ9 supplementation as indicated before determination of insulin sensitivity by HA-GLUT4 abundance at the plasma membrane (PM) in unstimulated and insulin-stimulated cells. Results show mean ±S.E.M. for four separate studies. #p<0.05 versus control cells, *p<0.05 versus insulin-stimulated control cells, †p<0.05 versus respective statin-treated cells without CoQ supplementation, t-tests corrected for multiple comparisons.
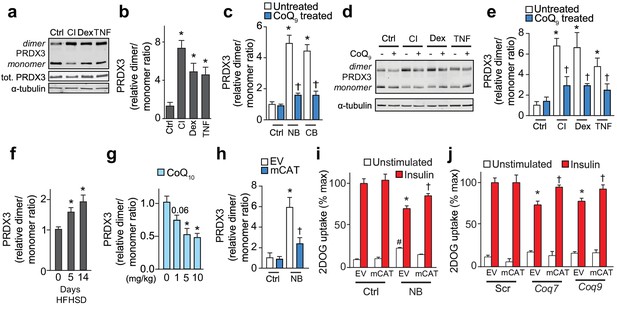
Loss of mitochondrial CoQ causes insulin resistance via mitochondrial oxidants.
(A–E) PRDX3 dimerisation was assessed by immunoblot in 3T3-L1 adipocytes treated as indicated, and the PRDX3 dimer/monomer ratio was measured by densitometry and expressed relative to control cells (B, C, E). Results show mean ±S.E.M. for four to eight separate in vitro experiments. For B, *p<0.05 versus control cells, t-tests corrected for multiple comparisons. For C and E, *p<0.05 versus control cells, †p<0.05 versus respective treatment without CoQ supplementation, t-tests corrected for multiple comparisons. (F) PRDX3 dimer/monomer ratio in adipose tissue obtained from mice fed a HFHSD (relative to ratios from adipose tissue from mice fed a HFHSD for 0 d). Results show mean of six mice, *p<0.05 versus control, t-tests corrected for multiple comparisons. (G) PRDX3 dimer/monomer ratio in adipose tissue from mice fed a HFHSD for 14 d and supplemented with CoQ10 at the specified dose. Results show mean of six mice (relative to ratios from adipose tissue from mice without CoQ10 supplementation). *p<0.05 versus control, t-tests corrected for multiple comparisons. (H) PRDX3 dimer/monomer ratio in adipocytes expressing empty vector (EV) or mitochondria-targeted catalase (mCAT) treated with and without NB. Ratios expressed relative to control EV cells. Results show mean ±S.E.M. for four separate in vitro experiments. *p<0.05 versus untreated EV control cells, †p<0.05 versus NB-treated EV cells, t-tests corrected for multiple comparisons. (I) 3T3-L1 adipocytes expressing EV or mCAT treated were treated with NB before insulin sensitivity was determined by 3H-2-DOG uptake. (J) 3H-2-DOG uptake in EV or mCAT expressing 3T3-L1 adipocytes treated with scrambled siRNA (Scr) or siRNA targeted to Coq7 or Coq9 and stimulated with insulin where indciated. Results show mean ±S.E.M. for four separate in vitro experiments. *p<0.05 versus insulin-stimulated EV control cells, †p<0.05 versus respective insulin-stimulated EV cells, t-tests corrected for multiple comparisons.
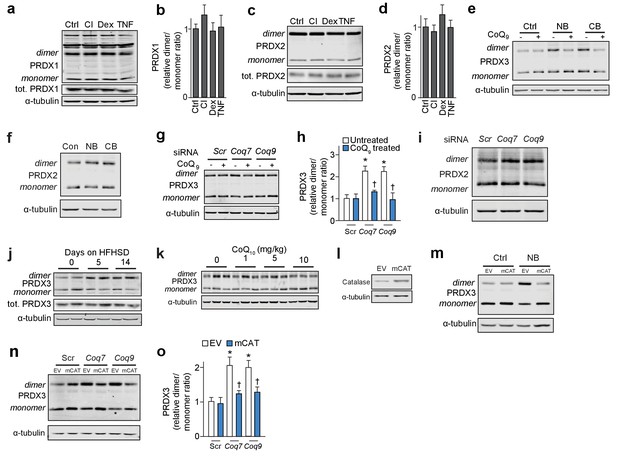
Adipocyte insulin resistance and loss of CoQ is associated with increased oxidants specifically in mitochondria.
(A–D) Western blot and densitometry analysis of the PRDX1 (A, B) and PRDX2 (C, D) dimerisation status in 3T3-L1 adipocytes treated as indicated. Ratios expressed relative to control cells. (E–I) PRDX3 (E, G, H) and PRDX2 (F, I) dimerisation was assessed by immunoblot in 3T3-L1 adipocytes treated as indicated to inhibit CoQ synthesis and the PRDX3 dimer/monomer ratio measured by densitometry and expressed relative to control cells (H). Blots are representative of at least three separate experiments. Results show mean ±S.E.M. of four independent experiments. *p<0.05 versus control cells, †p<0.05 versus siRNA-treated cells without CoQ supplementation, t-tests corrected for multiple comparisons. (J–K) Representative PRDX3 immunoblots in adipose tissue obtained from mice fed a chow or HFHSD for indicated times (J) or from mice fed a HFHSD and supplemented with CoQ10 as indicated (K) (quantified in Figure 6F and G). (L) Immunoblot validation of overexpression of catalase in 3T3-L1 expressing mitochondrial-targeted catalase (mCAT). (M–O) Immunoblot analysis of PRDX3 dimer/monomer ratio in 3T3-L1 adipocytes expressing EV or mCAT treated as indicated to inhibit CoQ synthesis with NB (M, quantified in Figure 6H) or siRNA to Coq7 or Coq9 (N–O). Ratios expressed relative to control cells in (O). Results show mean ±S.E.M. of three to four independent experiments. *p<0.05 versus control cells, †p<0.05 versus siRNA-treated cells without CoQ supplementation, t-tests corrected for multiple comparisons.
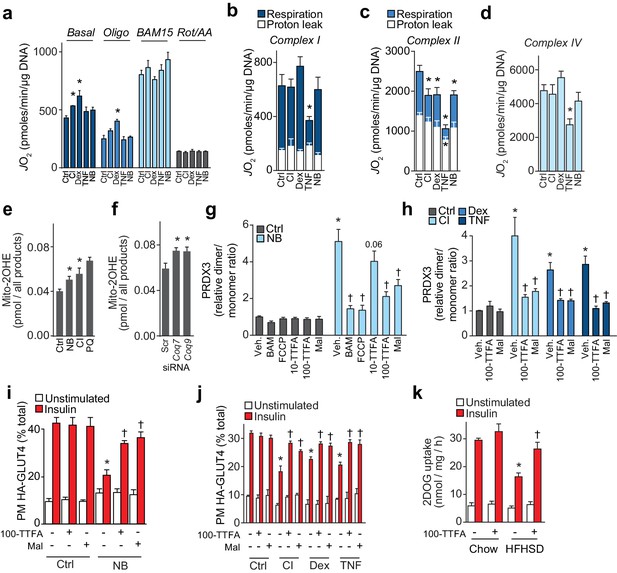
Loss of CoQ causes insulin resistance via complex II-derived superoxide/hydrogen peroxide.
(A) 3T3-L1 adipocytes were incubated in galactose and respiration was assessed using the XFp Analyzer. Following basal measurements, cells were sequentially treated with, oligomycin (Oligo), BAM15 (uncoupler), and rotenone/antimycin A (Rot/AA). (B–D) Mitochondrial respiration in 3T3-L1 adipocytes treated to induce insulin resistance as indicated. Cells were permeabilised with digitonin and specific substrates and inhibitors were added to assess complex I (B), II (C) and IV (D) activity as described in the Material and Methods. Results show mean ±S.E.M. of five to eight separate in vitro experiments. *p<0.05 versus control, t-tests corrected for multiple comparisons. (E–F) Concentration of 2-hydroxyethidium derivative of MitoSox in cells treated (Ctrl, control; NB, nitrobenzoic acid; CI; chronic insulin; PQ; paraquat) as indicated and incubated with MitoSox for 1 hr. Results show mean ±S.E.M. for three to eight separate in vitro experiments. *p<0.05 versus control cells, t-tests corrected for multiple comparisons. (G) Relative PRDX3 dimer/monomer ratio in adipocytes treated with or without NB and uncouplers (BAM15, FCCP) or inhibitors of complex II (10 or 100 µM TTFA (10-TTFA; 100-TTFA) and malonate (Mal). Results show mean ±S.E.M. of PRDX3 dimer/monomer ratio relative to control 3T3-L1 adipocytes across four separate in vitro experiments. *p<0.05 versus control cells, †p<0.05 versus NB-treated cells without additional treatment, t-tests corrected for multiple comparisons. (H) Relative PRDX3 dimer/monomer ratio in control or insulin-resistant adipocytes treated with or without inhibitors of complex II (100 µM TTFA or malonate). Results show mean ±S.E.M. of PRDX3 dimer/monomer ratio relative to control 3T3-L1 adipocytes across three separate in vitro experiments. *p<0.05 versus control cells, †p<0.05 versus insulin-resistant cells without additional treatment, t-tests corrected for multiple comparisons. (I) 3T3-L1 adipocytes were treated with NB and TTFA or malonate where indicated before sensitivity was determined by HA-GLUT4 abundance at the plasma membrane (PM). Results show mean ±S.E.M. for four separate in vitro experiments. *p<0.05 versus insulin-stimulated control cells, †p<0.05 versus insulin-stimulated NB-treated cells without additional treatment, t-tests corrected for multiple comparisons. (J) 3T3-L1 adipocytes were treated to induce insulin resistance and with 100 µM TTFA or 10 mM malonate where indicated before sensitivity was determined by HA-GLUT4 abundance at the plasma membrane (PM). Results show mean ±S.E.M. for three to four separate in vitro experiments. *p<0.05 versus insulin-stimulated control cells, †p<0.05 versus insulin-stimulated insulin-resistant cells without additional treatment, t-tests corrected for multiple comparisons. (K) Adipose tissue explants from mice fed a chow diet or a HFHSD for 14 d were incubated with or without TTFA prior to assessment of insulin-regulated 2-DOG uptake with 10 nM insulin. Results show mean ±S.E.M. of data from five mice. *p<0.05 versus insulin-stimulated explants from mice fed a chow diet, and †p<0.05 versus insulin-stimulated explants from HFHSD-fed mice without TTFA treatment, t-tests corrected for multiple comparisons. See also Figure 7—figure supplement 1.
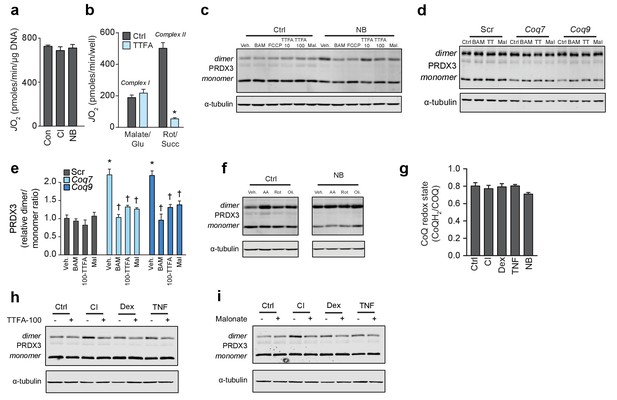
Additional data on the effect of loss of CoQ on oxidant production from mitochondrial complex II.
(A) Mitochondrial respiration was assessed in 3T3-L1 adipocytes treated to induce insulin resistance as indicated. Cells were permeabilised with digitonin and medium chain fatty acid oxidation assessed. Results show mean ±S.E.M. of four to eight separate in vitro experiments. (B) Mitochondrial respiration was assessed in digitonin permeabilised 3T3-L1 adipocytes. Complex I activity was assessed in the absence of the complex II inhibitor TTFA as a control for mitochondrial function. Complex II activity was assessed in the absence and presence of the complex II inhibitor TTFA (100 µM). Results show mean ±S.E.M. of three to four in vitro experiments. *p<0.05 versus control, t-tests corrected for multiple comparisons. (C) PRDX3 dimer/monomer ratio assessed by immunoblot in adipocytes treated with or without NB and uncouplers (BAM15, FCCP) or inhibitors of complex II (10 or 100 µM TTFA and 10 mM malonate) (quantified in Figure 7G). (D–E) PRDX3 dimerisation was assessed by immunoblot (D) in 3T3-L1 adipocytes treated siRNA to Coq7 or Coq9 and uncoupler (BAM15) or inhibitors of complex II (100 µM TTFA or 10 mM malonate) and the PRDX3 dimer/monomer ratio measured by densitometry (E). Results show mean ±S.E.M. of PRDX3 dimer/monomer ratio relative to control 3T3-L1 adipocytes across four independent experiments. *p<0.05 versus control cells, †p<0.05 versus siRNA-treated cells without additional treatment, t-tests corrected for multiple comparisons. (F) PRDX3 dimer/monomer ratio in adipocytes treated with or without NB and inhibitors of complex I (rotenone, 10 µM), complex III (antimycin A, 50 nM) or complex V (oligomycin, 10 µg/mL) was assessed by immunoblot. Images from same gel, lanes removed for clarity. Representative of three independent experiments. (G) CoQ redox status expressed as the ratio of reduced (CoQH2) to oxidised CoQ (CoQ). Results show mean ±S.E.M. of three to four independent experiments. (H–I) PRDX3 dimer/monomer ratio assessed by immunoblot in adipocytes treated to induce insulin resistance and treated with 100 µM TTFA (H) or 10 mM malonate (I) for 2 hr as indicated. Results representative of three independent experiments (quantified in Figure 7I).
Tables
Anthropometric, clinical and metabolic characteristics of obese females stratified into a upper tertile and lower two tertiles based on adipose tissue mitochondrial CoQ content (CoQhigh vs CoQlow).
All p values calculated by Mann Whitney test, p>0.05 in bold.
Characteristics | CoQhigh (n = 11) | CoQlow (n = 22) | P value |
---|---|---|---|
Age, y | 53 ± 11 | 51 ± 13 | 0.70 |
BMI, kg/m2 | 36.6 ± 4.2 | 36.9 ± 5.1 | 0.92 |
Subcutaneous fat, cm2 a | 588 ± 132 | 546 ± 114 | 0.33 |
Visceral fat, cm2 a | 210 ± 37 | 264 ± 74 | 0.07 |
Liver fat, % a | 6.8 ± 4.5 | 13.6 ± 10.3 | 0.06 |
Mean adipocyte size, μm b | 71.3 ± 8.9 | 72.6 ± 9.0 | 0.90 |
Total cholesterol, mmol/L | 5.0 ± 0.8 | 4.8 ± 0.7 | 0.87 |
LDL cholesterol, mmol/L | 3.0 ± 0.8 | 2.9 ± 0.6 | 0.89 |
HDL cholesterol, mmol/L | 1.5 ± 0.2 | 1.4 ± 0.3 | 0.19 |
Triacylglycerides, mmol/L | 0.8 ± 0.3 | 1.0 ± 0.4 | 0.15 |
Fasting insulin, mU/L | 14.2 ± 4.9 | 16.0 ± 7.4 | 0.71 |
Fasting NEFA, mmol/L | 0.46 ± 0.14 | 0.43 ± 0.13 | 0.63 |
NEFA during low dose insulin infusion, mmol/L | 0.03 ± 0.02 | 0.06 ± 0.03 | 0.03 |
NEFA suppression during low dose insulin infusion, % | 92.1 ± 4.5 | 86.5 ± 6.9 | 0.01 |
Glucose infusion rate during high dose insulin infusion, μmol/kg fat free mass/min | 117.8 ± 26.6 | 98.5 ± 29.4 | 0.02 |
Endogenous glucose production suppression during low-dose insulin infusion, % | 73.6 ± 12.1 | 60.5 ± 15.7 | 0.03 |
HOMA-IR score | 2.9 ± 1.1 | 3.5 ± 1.7 | 0.40 |
-
a –CoQhighn = 10, CoQlown = 22.
b - CoQhighn = 9, CoQlown = 18.
Reagent type (species) or resource | Designation | Source or reference | Identifiers |
---|---|---|---|
Strain, strain background (mouse) | C57/Bl6J mice | Animal Resources Centre (Perth, Australia) or Australian BioResources (Moss Vale, Australia) | RRID:IMSR_JAX:000664 |
Cell line (mouse) | Mouse: 3T3-L1 adipocytes | Dr Howard Green, Harvard Medical School | RRID:CVCL_0A20 |
Biological sample (human) | Human adipose tissue | Clinical details in PMID: 26378474 | |
Antibody | Mitochondrial complex subunits (OXPHOS) | Thermo Fisher Scientific | Cat# 45–8099 |
Antibody | PRDX1 | Thermo Fisher Scientific | Cat# PA3-750 |
Antibody | PRDX2 | Abcam | Cat# ab109367, clone: EPR5154 |
Antibody | catalase | Abcam | Cat# ab52477 |
Antibody | PRDX3 | Ab Frontier | Cat# LF-PA0030 |
Antibody | 14-3-3 | Santa Cruz | Cat# sc-629, clone K19 |
Antibody | pT642 TBC1D4 | Cell Signaling Technologies | Cat# 4288 |
Antibody | TBC1D4 | Cell Signaling Technologies | Cat# 2670 |
Antibody | pT308 Akt | Cell Signaling Technologies | Cat# 9275 |
Antibody | pS473 Akt | Cell Signaling Technologies | Cat# 4051 |
Antibody | Akt | Cell Signaling Technologies | Cat# 4685 |
Antibody | α-Tubulin | Sigma Aldrich | Cat# T9026 |
Antibody | GLUT4 | In-house | NA |
Antibody | anti-HA antibody | Covance | clone 16B1 |
Sequence-based reagent | qPCR primers: mCoq7_F; tttggaccatagctgcattg and mCoq7_R; tgaggcctcttccatactctg, | Sigma Aldrich | NA |
Sequence-based reagent | qPCR primers: mCoq9_F; tcagcagcattctgagacaca and mCoq9_R; gtgctgtagctgctcctcact, | Sigma Aldrich | NA |
Sequence-based reagent | qPCR primers: mCypB-F; ttcttcataaccacagtcaagacc; mCypB-R, accttccgtaccacatccat. | Sigma Aldrich | NA |
Sequence-based reagent | Scrambled siRNA: (sense 5′- CAGTCGCGTTTGCGACTGGTT-3′) | Shanghai Genepharma | NA |
Sequence-based reagent | anti-Coq7 siRNA (sense 5′- GGGAUCACGCUGGUGAAUAUTT-3′, 5′- GGAUGACCUUAGACAAUAUTT-3′, 5′- GCCUUGUUGAAGAGGAUUAUTT-3′) | Shanghai Genepharma | NA |
Sequence-based reagent | anti-Coq9 siRNA (sense 5′- GCAGCAUUCUGAGACACAGTT-3′, 5′- GCUGGUGAUGAUGCAGGAUTT-3′, 5′- GCAAUGAACAUGGGCCACATT-3′) | Shanghai Genepharma | NA |
Commercial assay or kit | Glycerol assay kit | Sigma Aldrich | Cat#: FG0100-1KT |
Commercial assay or kit | NEFA kit | Waki Pure Chemical Industries | Cat#: 279–75104 |
Commercial assay or kit | Insulin ELISA | Crystal Chem | Cat#: 90080 |
Commercial assay or kit | RNeasy kit | QIAGEN | Cat#: 74104 |
Commercial assay or kit | PrimeScript first strand cDNA synthesis kit | Clontech | Cat#: 6110A |
Chemical compound, drug | 2-[1,2-3H(N)]-deoxy-D-glucose | Perkin Elmer | Cat# NET328001MC |
Chemical compound, drug | Insulin from bovine pancreas | Sigma-Aldrich | Cat# I5500-1G; CAS 11070-73-8 |
Chemical compound, drug | Coenzyme Q9 | Sigma Aldrich | Cat# 27597–1 MG CAS 303-97-9 |
Chemical compound, drug | CoQ10 liposomal formulation | Tichson Corp | LiQsorb |
Chemical compound, drug | 4-Chlorobenzoic acid | Sigma Aldrich | Cat# 135585–50G CAS 74-11-3 |
Chemical compound, drug | 4-Nitrobenzoic acid | Sigma Aldrich | Cat# 461091–250G CAS 62-23-7 |
Chemical compound, drug | NEM | Sigma Aldrich | Cat# 04259–5G CAS 128-53-0 |
Chemical compound, drug | FCCP | Sigma Aldrich | Cat# C2920-10MG CAS 370-86-5 |
Chemical compound, drug | BAM15 | Dr Kyle Hoehn | PMID: 24634817 |
Chemical compound, drug | TTFA | Sigma Aldrich | Cat# T27006-25G CAS 326-91-0 |
Chemical compound, drug | Malonate | Sigma Aldrich | Cat# M1296-100G CAS 141-82-2 |
Chemical compound, drug | Isoproterenol | Sigma Aldrich | Cat# I6504-100MG CAS 5984-95-2 |
Chemical compound, drug | MitoSOX | Thermo Fisher Scientific | Cat# M36008 |
Software, algorithm | MaxQaunt | https://www.biochem.mpg.de/5111795/maxquant | Versions 1.5.7.0, 1.4.0.8, 1.3.0.5 |
Software, algorithm | Affeymetrix GeneChip Command Console Software | Thermo Fisher Scientific | NA |
Software, algorithm | R-programming environment – affy package | http://bioconductor.org/packages/affy/ | Version 1.52.0 |
Software, algorithm | R-programming environment – limma package | http://bioconductor.org/packages/limma/ | Version 3.30.3 |
Software, algorithm | R-programming environment – directPA package | https://CRAN.R-project.org/package=directPA | Version 1.3 |
Software, algorithm | R-programming environment – Re-Fraction package | https://github.com/PengyiYang/Re-Fraction (copy archived at https://github.com/elifesciences-publications/Re-Fraction) | Version 1.2 |
Software, algorithm | Image lab 5.2.1 | BioRad | NA |
Software, algorithm | Image Studio | LiCOR | NA |
Software, algorithm | Graphpad Prism | GraphPad Software Inc. | NA |
Deposited Data | Mouse and cell line mass spectrometry data. | ProteomeXchange Consortium | PXD005128. |
Deposited Data | Human mass spectrometry data. | ProteomeXchange Consortium | PXD006891 |
Deposited Data | Mouse and cell line microarray data. | https://www.ncbi.nlm.nih.gov/geo/query/acc.cgi?acc=GSE87853 | GSE87853 |
Deposited Data | Mouse and cell line microarray data. | https://www.ncbi.nlm.nih.gov/geo/query/acc.cgi?acc=GSE87854 | GSE87854 |
Additional files
-
Supplementary file 1
Proteomic analysis of insulin-resistant mouse adipose tissue and 3T3-L1 adipocytes.
(A-D) Differential expression and directional analyses of proteomic data from adipose tissue (A, B) and 3T3-L1 adipocytes (C, D). Values in directional analysis sheets are p values. E, Pathway analysis of tissue and cell proteomic data.
- https://doi.org/10.7554/eLife.32111.018
-
Supplementary file 2
Transcriptomic analysis of insulin-resistant mouse adipose tissue and 3T3-L1 adipocytes.
(A-D) Differential expression and directional analyses of transcript data from adipose tissue (A, B) and 3T3-L1 adipocytes (C, D). Values in directional analysis sheets are p values. E, Pathway analysis of tissue and cell transcript data.
- https://doi.org/10.7554/eLife.32111.019
-
Supplementary file 3
Correlation of the human adipose tissue proteome with clinical traits.
Correlation of protein (A) and pathway (B) expression with specified clinical measures. Significant r value = <−0.423 or >0.423. Tables contain combined z-score for proteins and pathway from in vivo and in vitro analyses.
- https://doi.org/10.7554/eLife.32111.020
-
Transparent reporting form
- https://doi.org/10.7554/eLife.32111.021