Late developing cardiac lymphatic vasculature supports adult zebrafish heart function and regeneration
Figures
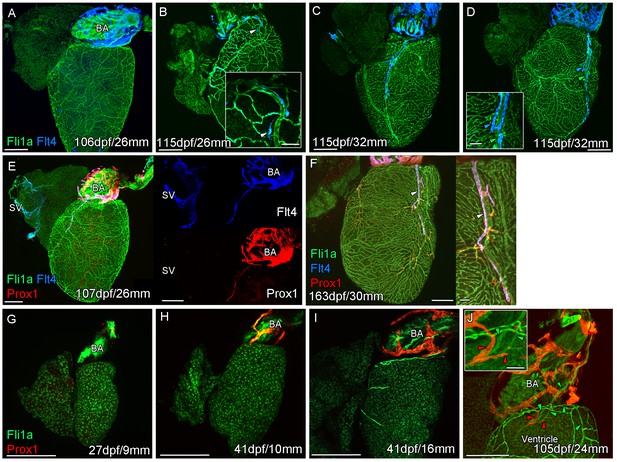
Prolonged development of the cardiac lymphatic system in juvenile and young adult zebrafish.
Whole-mount confocal imaging of non-cleared (A-D, F-I) and cleared (E,J) adult (A-F) and juvenile (G-J) transgenic zebrafish hearts expressing the pan-endothelial marker fli1a:GFP (Green), venous/lymphatic endothelial marker flt4:mCitrine (blue: A-F) and lymphatic endothelial marker prox1:Gal4-UAS:RFP (red: E-J). (A) Through juvenile and young adult stages, flt4:mCitrine-expressing endothelial cells are restricted to the surface of the BA. (B) At 26 mm standard body length, sprouts from this BA population are first observed on the ventricle surface (arrowhead and insert). (C) These sprouts then extend down the ventricle and start to form a cardiac lymphatic vessel that eventually extends the entire length of the ventricle to the apex (D). The cardiac lymphatic vessel (D, inset, blue arrowhead) bridges the blood vessel (D, inset, green arrowhead) it accompanies. (E) Distinct from the venous endothelium of the SV, the BA population and the sprouts extending from it also express prox1:Gal4-UAS:RFP. (F) Both flt4:mCitrine and prox1:Gal4-UAS:RFP are maintained in the formed cardiac lymphatic vessel (arrowhead). (G) Larval and early juvenile zebrafish lack the prox1:Gal4-UAS:RFP expressing lymphatic endothelium on the BA. (H) This population is observed in juvenile zebrafish prior to the onset of and during (I) coronary vessel development. (J) In early adult stages, alignment of single cell sprouts off the lymphatic endothelium (red arrowhead) with blood vasculature (green arrowhead) is observed within the cleft between the BA and ventricle. Scale bars 200 μm (A-J) and 50 μm (insets, B, D, F), n ≥ 3 (A-J).
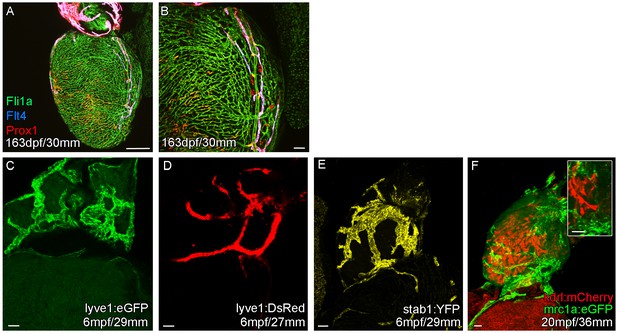
Expression of lymphatic endothelial marker genes in the cardiac lymphatic system.
Whole-mount confocal imaging of transgenic zebrafish hearts expressing the pan-endothelial fli1a:GFP (A and B, Green), venous/lymphatic flt4:mCitrine (A and B, Blue), lymphatic makers prox1:Gal4-UAS:RFP (A and B, Red), lyve1:eGFP (C, Green), lyve1:DsRed (D, Red), stab1:YFP (E, Yellow), mrc1a:eGFP (F, Green), blood endothelial marker kdrl:mCherry (F, Red). (A and B) In more mature zebrafish the cardiac lymphatic vessel extends the entire length of the ventricle expressing both prox1:Gal4-UAS:RFP and flt4:mCitrine its entire length. The Cardiac lymphatic endothelium expresses lyve1:eGFP/DsRed (C and D), stab1:YFP (E) and mrc1a:eGFP (F). Unlike blood endothelial cells, these lymphatic endothelial cells completely lack expression of kdrl:mCherry (F). Scale bars 200 μm (A) and 50 μm (B-F).
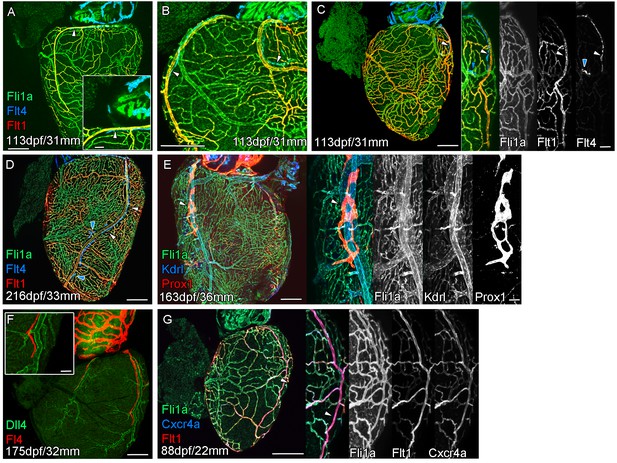
Extension of the cardiac lymphatic system along arteries in adult zebrafish.
Whole-mount confocal imaging of adult transgenic zebrafish hearts expressing the pan-endothelial fli1a:GFP (green: A-E, G), venous/lymphatic endothelial marker flt4:mCitrine (blue: A-D, red: F), arterial endothelial cell marker flt1enh:tdTomato (red: A-D, G), lymphatic endothelial marker prox1:Gal4-UAS:RFP (red: E), arterially enriched blood endothelial marker kdrl:mTurquoise (blue: E) and arterial marker dll4:GFP (F). (A-C) flt4:mCitrine-positive sprouts migrate along flt1enh:tdTomato expressing arteries not flt1enh:tdTomato-negative veins (white arrowheads). The extension appears somewhat dynamic and fluid, with gaps or dissociations observed during extension and formation (blue arrowheads). This association continues as the cardiac lymphatic vessel forms on the ventricle (D). The formed vessel endothelium is kdrl:mTurquoise-negative and is in close proximity to high-kdrl:mTurquoise (E), dll4:GFP (F) expressing arteries. flt1enh:tdTomato expressing arteries also express high levels of cxcr4a:mCitrene in young adult zebrafish (G). Scale bars 200 μm (A-G) and 50 μm (insets A, C, E, F and G), n ≥ 3 (A-G).
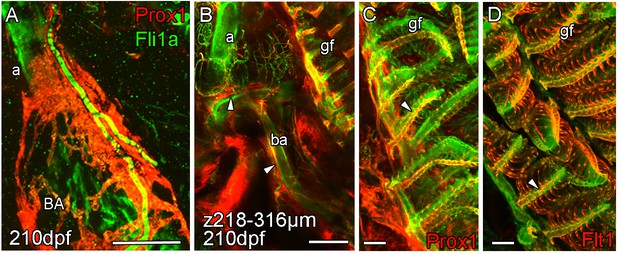
Arterial association of extra-cardiac lymphatic vasculature.
Whole-mount confocal imaging of uncleared (A) and cleared (B-D) adult transgenic zebrafish hearts expressing the pan-endothelial fli1a:GFP (green), lymphatic endothelial marker prox1:Gal4-UAS:RFP (red: A-C) and arterial marker flt1enh:tdTomato (red: D). (A) The association with artery is somewhat continued on the BA where the lymphatic vasculature extends up the aorta (a) towards the facial lymphatic vessels and also branches away from the cardiovascular system at the connection between the aorta and the 4th afferent brachial artery (ba). At this point, lymphatic vessels from the tips of the gill filaments (gf) on the arterial side (C and D, arrowheads) connect to the cardiac lymphatic system along the brachial arteries (B, arrowheads). Scale bars 200 μm (A-B) and 50 μm (C-D).
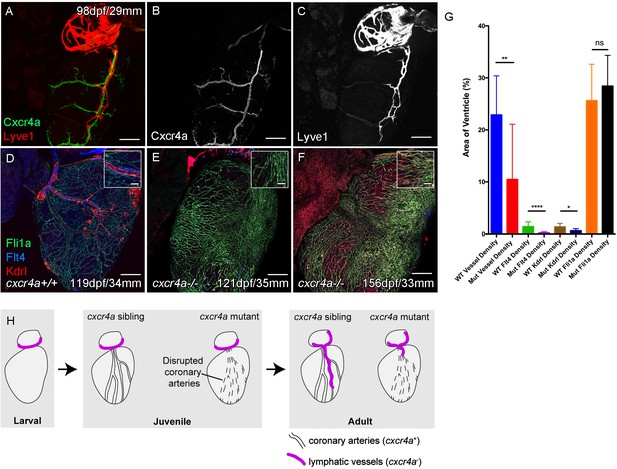
Cardiac lymphatic development requires a coronary artery scaffold.
Whole-mount confocal imaging of adult transgenic zebrafish hearts expressing arterial marker cxcr4a:mCitrine (green: A and B), and lymphatic endothelial markers lyve1:RFP (Red: A and C) and flt4:mCitrine (Blue: D-F), the pan-endothelial marker fli1a:GFP (green: D-F), and the arterially-enriched pan-endothelial marker kdrl:mTurquoise (red: D-F). (A-C, n = 8) cxcr4a:mCitrine expression is restricted to the coronary arteries and not detectable in lyve1:RFP-positive LECs. (D) Cardiac lymphatic vessels in control (cxcr4a+/+, n = 8) zebrafish. (E and F) In cxcr4a mutants (cxcr4a-/-, n = 12) that have abnormal and discontinuous coronary vasculature the cardiac lymphatic extension is stunted. (G) Graph showing significant reductions in vessel density, kdrl expression in blood vessels and flt4 expression on the surface of the cxcr4a-/- ventricle. There is comparable levels of fli1a-expressing cells on the surface of the ventricle between larger/older cxcr4a-/- and control zebrafish, but loss of the coronary structure. (H) Schematic representation of the regulation of cardiac lymphatic developmental guidance by coronary arteries. Scale bars 200 μm.
-
Figure 3—source data 1
Source data for (G).
- https://cdn.elifesciences.org/articles/42762/elife-42762-fig3-data1-v2.xlsx
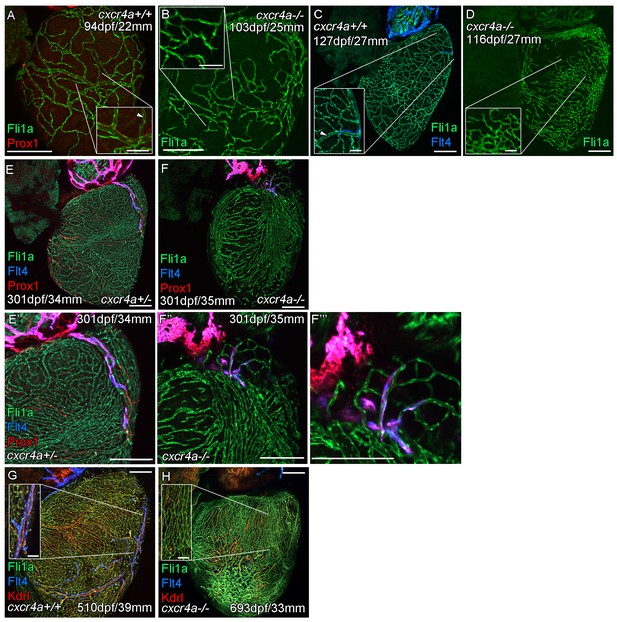
Cardiac lymphatic vessels extension is correlated with extent of the coronary arterial tree.
Whole-mount confocal imaging of adult transgenic zebrafish hearts expressing the pan-endothelial fli1a:GFP (green: A-H), Arterially and endocardially enriched endothelial marker kdrl:mTurquoise (red: G and H), lymphatic expressed flt4:mCitrine (blue: C, E-H); prox1:Gal4-UAS:RFP (red: A, E, F). (A and C) Formation of coronary vessels prior to and during the extension of the lymphatic vessel onto the ventricle in wildtype transgenic zebrafish (arrow head: lumenized vessel, A; coronary artery, B). (B) Much of the coronary vasculature is not formed in adult cxcr4a mutant zebrafish prior to the lymphatic extension and there are no identifiable lumenized vessels. (D) The formation of some coronary vessels in older adult mutants is mispatterned and abnormal during the time of lymphatic extension. (E and F) Images of hearts showing mispatterned coronary vasculature and failure of lymphatic extension in 301dpf cxcr4a mutants (E’, F’’ and F’’’ higher magnification images of the respective lymphatic vessels). (G and H) Lack of cardiac lymphatic vessels on the ventricle persists in the majority of older adult cxcr4a mutants (693dpf) that have considerably more coronary vessels than at earlier stages. (H) 693dpf cxcr4a mutant zebrafish lack identifiable coronary arteries that normally express high levels of kdrl:mTurquoise and along which flt4:mCitrine positive lymphatic vessels align (G, inset). Scale bars 200 μm and 50 μm (insets A-D, G and H).
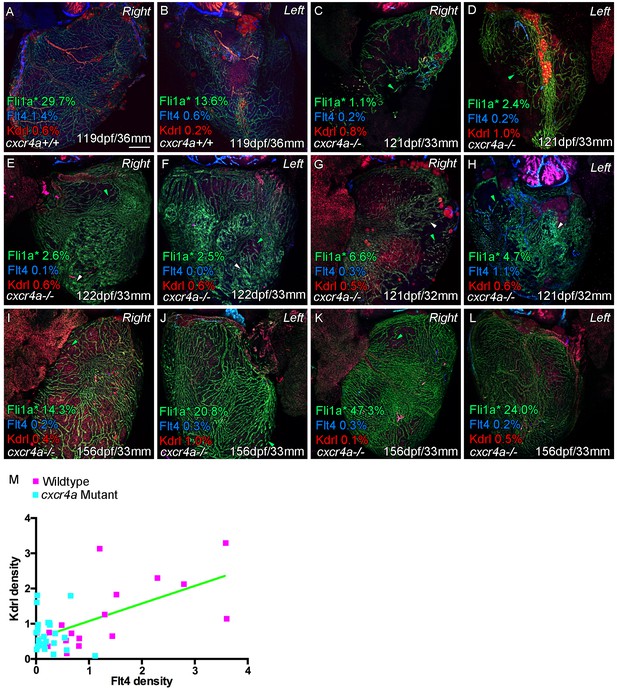
Cardiac lymphatic vessels extension is correlated with extent of the coronary arterial tree.
Whole-mount confocal imaging of adult transgenic zebrafish hearts expressing the pan-endothelial fli1a:GFP (green: A-L), Arterially and endocardially enriched endothelial marker kdrl:mTurquoise (red: A-L), lymphatic expressed flt4:mCitrine (blue: A-L). Estimated percentage coverage of surface fli1a:GFP expression within coronary vessel - as opposed to isolated cells or strings of such cells (green arrowheads) or non-lumenized conglomerates of cells (white arrowheads) - is listed for each image (Fli1a*) along with surface kdrl:mTurquoise and flt4:mCitrine coverage. Normally patterned coronary vessels show higher expression of kdrl:mTurquoise and are flanked by flt4:mCitrine-positivevessels on both sides of the heart (A and B). cxcr4a mutants show consistent abnormality in coronary vessel formation (C-L, range of mutant phenotypes including outliers). The degree of identifiably formed vessels varies in older mutants, but the spatial patterning of such vasculature over ventricle and the lack of kdrl:mTurquoise expression suggest no coronary artery tree structure. The extent of such coronary structure appears to be correlated with lymphatic extension down the ventricle, and is also largely absent in mutants. (M) Quantification of expression of surface kdrl:mTurquoise and flt4:mCitrine on individual sides of the heart (extension of arterial/lymphatic vessels is often more prominent on one or other side in wild type transgenic zebrafish, R2 = 0.36; p<0.0001). Scale bars 200 μm.
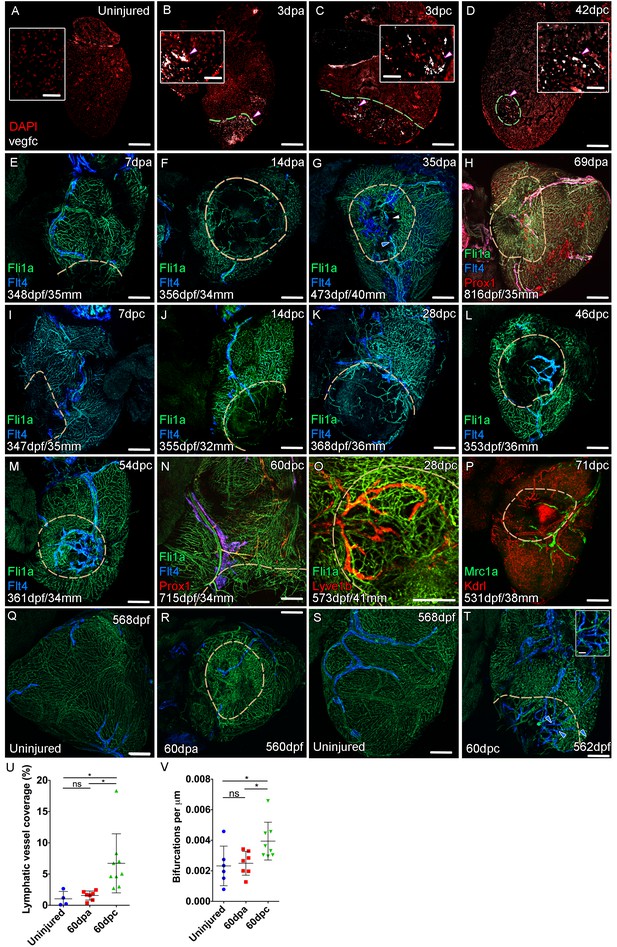
Lymphangiogenesis in response to injury of the zebrafish ventricle.
Confocal images of RNAScope section in situ hybridization to detect vegfc (white: A-D). Whole-mount confocal imaging of adult transgenic zebrafish hearts expressing the pan-endothelial marker fli1a:GFP (Green: E-O, Q-T) and lymphatic endothelial markers flt4:mCitrine (blue: E-O, Q-T), prox1:Gal4-UAS:RFP (red: H and N), lyve1:RFP (Red: O), mrc1a:eGFP (Green: P) and endothelial marker kdrl:mCherry (Red: P). (E-H) Response to amputation of flt4-expressing lymphatic vasculature during regeneration at 7 (E), 14 (F), 35 (G) and 69dpa (H). Lymphatic vasculature is observed to extent into the wound site at 35dpa to connect to the vessel on the BA (blue arrowhead) with some disconnections observed (white arrowhead). Response of flt4-expressing lymphatic vasculature to cryoinjury at 7 (I), 14 (J), 28 (K), 46 (L), 54 (M) and 60dpc (N). Expression prolife of regenerated lymphatic vasculature shows expression of prox1:Gal4-UAS:RFP (red: H and N), lyve1:RFP (Red: O) and mrc1a:eGFP (Green: P). (Q and S) Lymphatic vessels (blue arrowheads) extend to the apex in uninjured zebrafish. After amputation, there is little lymphangiogenesis and most of the regenerated vasculature lacks lymphatic marker expression with some minor extension into the wound site (R, blue arrowhead). Extensive lymphangiogenesis into and around the wound site (60dpc, T, blue arrowheads) is stimulated following damage to the myocardium with cryoinjury. (U) Quantification of the increase in area of flt4:mCitrine expression within a set 600 μm2 region centered on the wound site of sibling zebrafish subject to severe cryoinjury (n = 9) in comparison to that resulting from amputation (n = 7; *p=0.0132, unpaired t-test) or the uninjured apex (n = 4; *p=0.0407, unpaired t-test). (V) Quantification of increased branching of lymphatic vessels on the ventricle in response to cryoinjury (n = 9) in comparison to uninjured hearts uninjured hearts (n = 6; *p=0.0293, unpaired t-test) and those subjected to amputation (n = 7; *p=0.0140, unpaired t-test). Scale bars 200 μm (A-D) and 50 μm (insets).
-
Figure 4—source data 1
Source data for (U).
- https://cdn.elifesciences.org/articles/42762/elife-42762-fig4-data1-v2.xlsx
-
Figure 4—source data 2
Source data for (V).
- https://cdn.elifesciences.org/articles/42762/elife-42762-fig4-data2-v2.xlsx
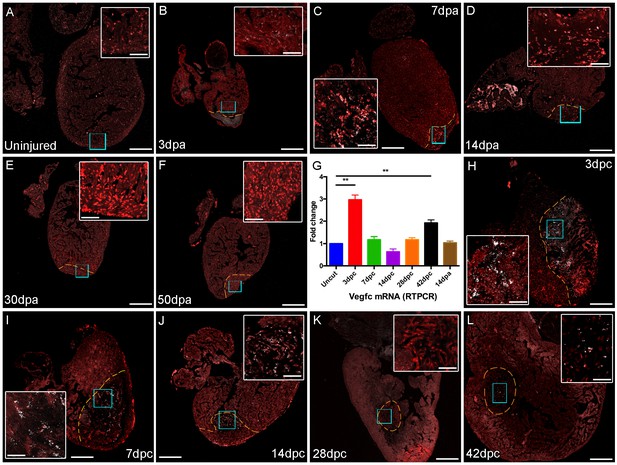
vegfc expression after cardiac injury.
Section in situ hybridization showing expression of vegfc after amputation (B-F) and cyro-injury (H-L) of adult zebrafish hearts. vegfc is elevated in the woundsite following both cryoinjury and amputation although appears to decease after 14dpa, in contrast it remains elevated after 42dpc. (G) RT-PCR of vegfc levels after injury show significantly elevated levels of vegfc in RNA extracted from ventricles at 3dpc and 42dpc. Scale bars 200 μm and 50 μm (insets).
-
Figure 4—figure supplement 1—source data 1
Source data for Supplement 1(G).
- https://cdn.elifesciences.org/articles/42762/elife-42762-fig4-figsupp1-data1-v2.xlsx
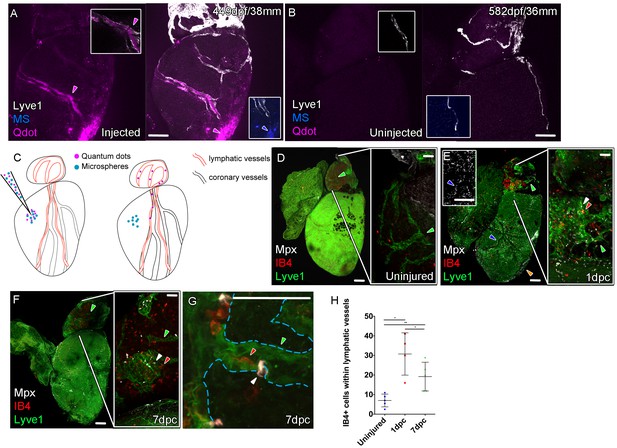
Function of the cardiac lymphatic vasculature in regeneration.
Whole-mount confocal imaging of adult transgenic zebrafish hearts expressing lyve1:RFP (White: A and B) and lyve1:GFP (Green: D-G) to mark lymphatic vessels. Additional fluorescence signal from injected Firefli Fluorescent Blue-dyed Microspheres (MA; blue: A and B), Quantum dots (Qdot; magenta: A and B) and immuno-labeled neutrophils (Mpx; white: D-G) and Macrophage (IB4; red:D-G). (A) Intra-myocardial injection of 200 nm microspheres (cyan) and 10 nm Qdots (magenta) results in both being deposited at the injection site (magenta/cyan arrowheads). Qdots, but not MS are also highly concentrated within the lymphatic vessels within the lyve1:RFP positive endothelium (arrowheads, A; uninjected control, B). (C) Schematic representation of nanoparticle uptake by the cardiac lymphatic system. (D, n = 5) Uninjured zebrafish heart labeled with antibodies against GFP (lyve1:GFP) and Mpx and lectinB4 (IB4), showing relatively few Mpx-positive neutrophils or IB4-positive macrophage on the ventricle or BA, including within the lymphatic vessels on the BA (green arrowheads). (E, n = 4) 1 day post-cryo-injured (dpc) heart (mild injury) labeled at the same time shows a massive increase in Mpx-expressing neutrophils and more moderate increases IB4-positive macrophage including within the lymphatic vasculature (green arrowhead (GFP) white arrowhead (Mpx) red arrowhead (IB4). Mpx-positive cells are concentrated along the visibly darker blood vessels (inset, blue arrowhead) away from the wound site (orange arrowhead). (F, n = 5) 7dpc heart showing persistent elevation of Mpx-positive and IB4-positive cells on the ventricle and BA, where they are associated with lymphatic vessels. (G) High resolution imaging of lyve1:GFP -positive vessels (green arrowhead, outlined in blue) on the BA of a 7dpc heart IB4-positive macrophage (red arrowhead) within the vessel and Mpx-positive neutrophil (white arrowhead) on the surface of the vessel (H) Graph showing quantification of increase in IB4-positive cells within the BA lymphatic vessels of 1dpc (n = 4) and 7dpc (n = 5) zebrafish hearts compared to those of uninjured hearts (n = 5). *p<0.05; **p<0.01, unpaired t-test, Mean and SD. Scale bars 200 μm (A-G) and 50 μm (right panels, D-F).
-
Figure 5—source data 1
Source data for (H) and Supplement 1(G).
- https://cdn.elifesciences.org/articles/42762/elife-42762-fig5-data1-v2.xlsx
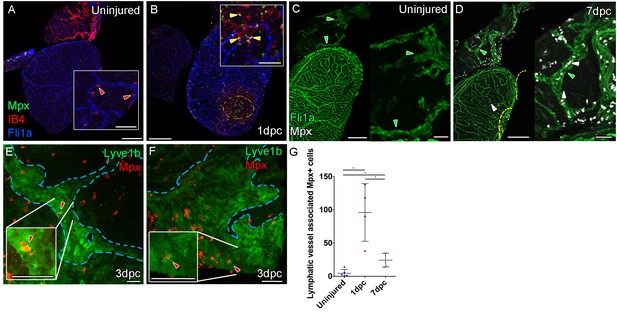
Immune response by macrophage and neutrophils to mild cyro-injury Uninjured zebrafish heart.
(A) labeled with antibodies against GFP (fli1a:GFP, blue) IB4 (red) and Mpx (green) showing no Mpx-positive neutrophils on the ventricle only resident IB4-positive macrophage (red arrowhead). (B) 1 day post cryoinjured (dpc) heart (mild injury) labeled at the same time shows a massive increase in Mpx-expressing neutrophils and IB4-positive macrophage on the ventricle and concentrated at the wound site (demarked with yellow dashed line). In many cases, IB4-positive macrophage have engulphed Mpx-positive cells or debris as indicated with green staining within red-labeled macrophage cells (yellow arrowhead, inset within the wound site). (C, n = 8) Uninjured zebrafish heart labeled with antibodies against GFP (Fli:GFP, green) and Mpx showing no Mpx-positive neutrophils on the ventricle or BA, including within the lymphatic vessels on the BA (green arrowheads). (D, n = 6) 7dpc heart showing Mpx-positive cells (white, white arrowheads) on the ventricle, in the wound site (yellow dashed line) and on the BA, within and on the lymphatic vessels. (E and F) High resolution imaging of lyve1:GFP -positive vessels (green, blue outline) on the BA of a 3dpc heart with Mpx-positive neutrophil (red, red arrowhead) within the lymphatic vessel (see Figure 5—videos 4 and 5). (G) Graph showing quantification of increase in Mpx-positive cells within the BA lymphatic vessels of 1dpc (n = 4) and 7dpc (n = 5) zebrafish hearts compared to those of uninjured hearts (n = 5). *p<0.05, unpaired t-test, Mean and SD. Scale bars 200 μm and 50 μm (insets).
Concentrated Qdot signal within the cardiac lymphatic lumen after intermyocardial injection.
Vision4D rendered whole-mount confocal image of an adult transgenic zebrafish heart expressing lyve1:RFP to mark lymphatic vessels and additional fluorescence signal from myocardial injected Firefli Fluorescent Blue-dyed Microspheres (MA; blue) and Quantum dots (Qdot; magenta). Injects results in Qdot uptake into the ventricular cardiac lymphatic vessels to become highly concentrated within the lyve1:RFP positive endothelial lumen and can be seen on both sides of an unlabeled optically dense blood vessel. Though commonly observed bridge points, the vessel containing Qdots can also be seen on top of the blood vessel to connect with discontinuous lymphatic vessel on the other side.
IB4-positive macrophage within the a cardiac lymphatic vessel.
Vision4D rendered high-resolution image of Lyve1-positive vessels (green) on the BA of a 7dpc heart IB4-positive macrophage (red) within the vessel and Mpx-positive neutrophil (white) on the surface of the vessel.
IB4-positive macrophage within the a cardiac lymphatic vessel.
Optical progression through the Z-plane of high-resolution image of Lyve1-positive vessels (green) on the BA of a 7dpc heart IB4-positive macrophage (red) within the vessel and Mpx-positive neutrophil (white) on the surface of the cardiac lymphatic vessel.
Mpx-positive neutrophils within the a cardiac lymphatic vessel.
Vision4D rendered high-resolution image of Lyve1-positive vessels (green) on the BA of a 3dpc heart with an Mpx-positive neutrophil (red) within the cardiac lymphatic vessel on the surface of the BA.
Mpx-positive neutrophils within the a cardiac lymphatic vessel.
Vision4D rendered high-resolution image of Lyve1-positive vessels (green) on the BA of a 3dpc heart with an Mpx-positive neutrophil (red) within the cardiac lymphatic vessel on the surface of the BA.
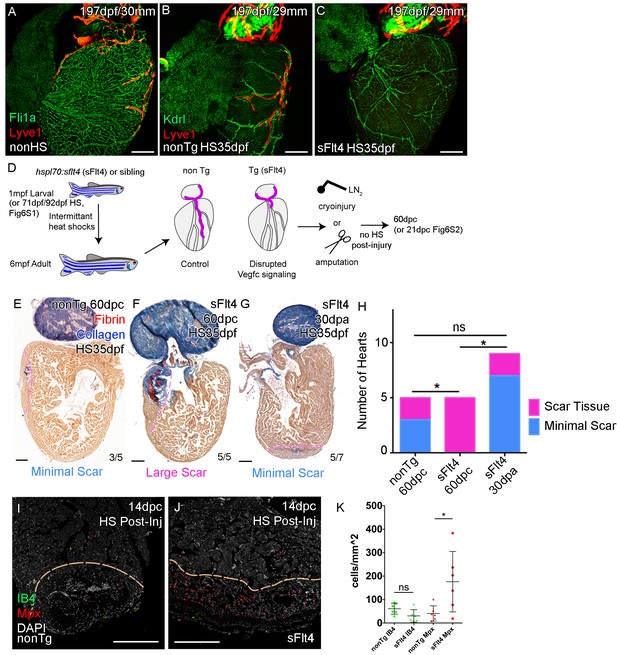
Cardiac lymphatic development requires Vegfc signaling and helps reduce scar volume in response to cryoinjury.
Whole-mount confocal imaging of adult transgenic zebrafish hearts expressing the pan-endothelial fli1a:GFP (green: A), Arterially and endocardially enriched endothelial marker kdrl:GFP (green: B, C), lymphatic endothelial marker lyve1:RFP (Red: A-C). (A) Heart with normal cardiac lymphatic vessels from stage and size-matched control zebrafish (nonHS, n = 7). (B) Hearts from non-Transgenic zebrafish (nonTg, n = 3) that are exposed to heat shock with cardiac lymphatic vessels on the heart that extend further and cover more of the ventricle. (C) In contrast, hearts from hsp70l:sflt4 zebrafish (sFlt4, n = 7), heat shocked in the same way lack extension of the cardiac vasculature onto the ventricle. (D) Schematic representation of the experimental procedure and regulation of cardiac lymphatic development by inducing sFlt4. AFOG-histology images showing fibrin in red and collagen in blue in developmentally heat shocked (1 to 6mpf) non-transgenic (nonTg; E) and transgenic (hsp70l:sflt4; sFlt4; F and G) zebrafish 60 days following severe cryoinjury (60dpc, 8mpf, E and F) or 30 days after apex resection (30dpa, 7mpf, G). Scar volume was estimated and grouped based on severity for cryoinjured hearts (minimal scar,<0.005 mm3, E; large scar,>0.005 mm3, F) and amputated hearts (minimal scar, G). (H) Graph showing the distribution of tissue scaring between non-transgenic (nonTg, n = 5) and transgenic (hsp70l:sflt4) zebrafish at 60dpc (sFlt4, n = 5) and 30dpa (n = 7), *p<0.05, two-tailed Chi-Squared. Fluorescence signal from immuno-labeled neutrophils (Mpx; Red: I and J) and Macrophage (IB4; green: I and J) in and near the 14dpc woundsite (demarked orange; Post-injury sFlt4 induction, n = 6 per group). (J) Elevated level of Mpx-positive immune cells at the 14dpc woundsite of fish with post-injury induced sFlt4 (n = 6). (K) Separate quantification of Mpx and IB4-positive cells in and within 100 μm of the 14dpc woundsite (average of at least three images through woundsite, individually normalized to woundsite area, n = 6 per group). *p<0.05; **p<0.01; ***p<0.001, unpaired t-test, Mean and SD. Scale bars 200 μm.
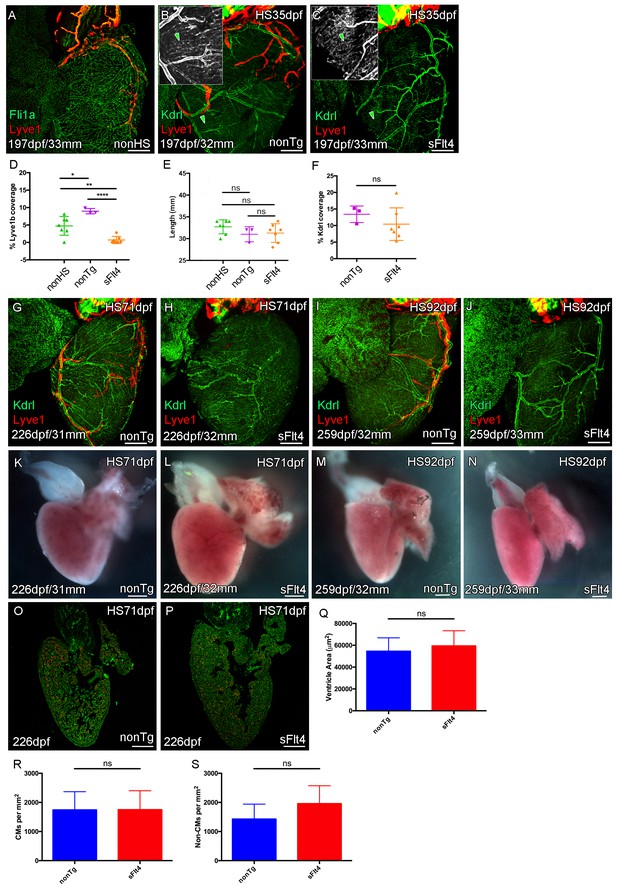
Juvenile Vegfc signaling is required for cardiac lymphatic development.
Whole-mount confocal and reflected brightfield imaging of adult transgenic zebrafish hearts expressing the pan-endothelial fli1a:GFP (green: A), arterially and endocardially enriched endothelial marker kdrl:GFP (green: B, C, G-J; green arrowhead: B and C), lymphatic endothelial marker lyve1:RFP (red: A-C, G-J). (A) Non-heat shock control (nonHS) zebrafish raised in otherwise identical conditions show normal cardiac lymphatic growth on the ventricle. (B) Increased ventricle lymphatic vasculature in response to heat shock (from 1 m/35dpf) in non-hsp70l:sflt4 transgenic zebrafish (nonTg). (C) Loss of cardiac lymphatic outgrowth onto the ventricle in heat-shocked hsp70l:sflt4 transgenic zebrafish (sFlt4) with no discernable effect on the coronary vessels (green arrowheads) (D) Quantification of lymphatic vessel coverage on the ventricle shows significant changes in coverage; increased in non- hsp70l:sflt4 (n = 3) and decreased in hsp70l:sflt4 (n = 7) fish heat shocked from 35dpf compared to non-heat shocked controls (n = 7; *p=0.0311; **p=0.0028; ****p<0.0001). (E) Heat shocked fish are not significantly (ns) different in length to non-heat shocked controls or each other. (F) Quantification showing there is no significant difference in ventricle coverage of kdrl:GFP with sFlt4 induction. (G and I) Non-transgenic zebrafish have extensions of the cardiac lymphatic vessel (red) onto the ventricle with heart shock from 71 (G) and 92dpf (I). (H and J) Hearts from hsp70l:sflt4 zebrafish heat shocked from 71 (H) and 92dpf (J) completely lack significant extension of cardiac lymphatic vessels onto the ventricle, but show no defects in the coronary vasculature. (K-N) Reflected brightfield images of the same hearts shown in G-J suggest no effect on gross morphology or heart size with heat-shock nonTg (K and M) or heat-shock-induced sFlt4 (L and N). (O and P) Examples images of developmentally heat-shocked (HS 71dpf) hsp70l:sflt4 and nonTg zebrafish with labeled cardiomyocytes (CM, red) and DAPI (green). (Q-S) Quantification of ventricle tissue area (Q), cardiomyocytes number (R), and non-cardiomyocytes number (S) show no significant difference with induction of the sFlt4 transgene in juvenile (HS 71dpf) zebrafish.
-
Figure 6—figure supplement 1—source data 1
Source data for Supplement 1(D).
- https://cdn.elifesciences.org/articles/42762/elife-42762-fig6-figsupp1-data1-v2.xlsx
-
Figure 6—figure supplement 1—source data 2
Source data for Supplement 1(F).
- https://cdn.elifesciences.org/articles/42762/elife-42762-fig6-figsupp1-data2-v2.xlsx
-
Figure 6—figure supplement 1—source data 3
Source data for Supplement 1(Q–S).
- https://cdn.elifesciences.org/articles/42762/elife-42762-fig6-figsupp1-data3-v2.xlsx
-
Figure 6—figure supplement 1—source data 4
Source data for Supplement 1(J and K).
- https://cdn.elifesciences.org/articles/42762/elife-42762-fig6-figsupp1-data4-v2.xlsx
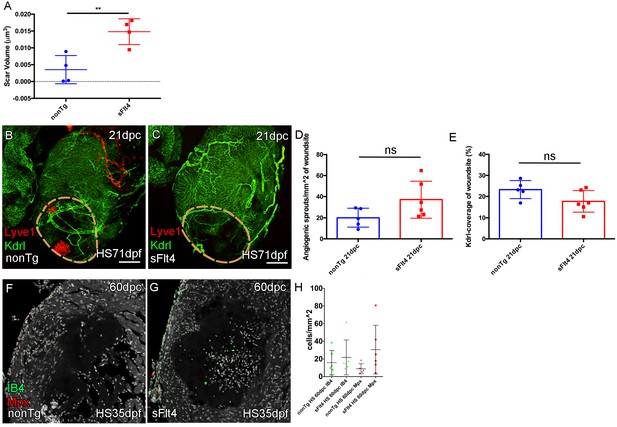
Blocking juvenile cardiac lymphatic development results increased scaring after cryoinjury at adult stages.
(A) The volume of scar in hearts lacking ventricle cardiac lymphatic vessels (after hsp70l:sflt4 induction from 1mpf; no induction post injury) is significantly larger at 60dpc (n = 4; **p=0.0075; one outlier with abnormally large scar, 0.036 μm3 and 0.051 μm3, removed from nonTg and sFlt4 groups respectively). (B–C) Whole-mount confocal imaging of 21dpc adult transgenic zebrafish hearts (heat shocked from 71dpf to 230dpf) expressing arterially and endocardially enriched endothelial marker kdrl:GFP (green: B, C) and lymphatic endothelial marker lyve1:RFP (red: B, C). Pre-injury induction of hsp70l:sflt4 (from 71dpf) does not inhibit angiogenesis into the woundsite after injury. (D) Non-significant (ns) slight increase in angiogenic sprouts into the woundsite is observed at 21dpc with juvenile-induced pre-injury hsp70l:sflt4. (E) A reciprocal, but also non-significant, small decrease in average vessel coverage of the wound site is observed in the same zebrafish. (F–H) Some hearts lacking ventricle lymphatic vessels show higher numbers of regeneration site immune cells at 60dpc, but overall there is no significant difference. Scale bars 200 μm.
-
Figure 6—figure supplement 2—source data 1
Source data for Supplement 2(D) and (E).
- https://cdn.elifesciences.org/articles/42762/elife-42762-fig6-figsupp2-data1-v2.xlsx
-
Figure 6—figure supplement 2—source data 2
Source data for (H) and Supplement 2(A).
- https://cdn.elifesciences.org/articles/42762/elife-42762-fig6-figsupp2-data2-v2.xlsx
-
Figure 6—figure supplement 2—source data 3
Source data for (K), Supplement 2(H) and Supplement 4(C).
- https://cdn.elifesciences.org/articles/42762/elife-42762-fig6-figsupp2-data3-v2.xlsx
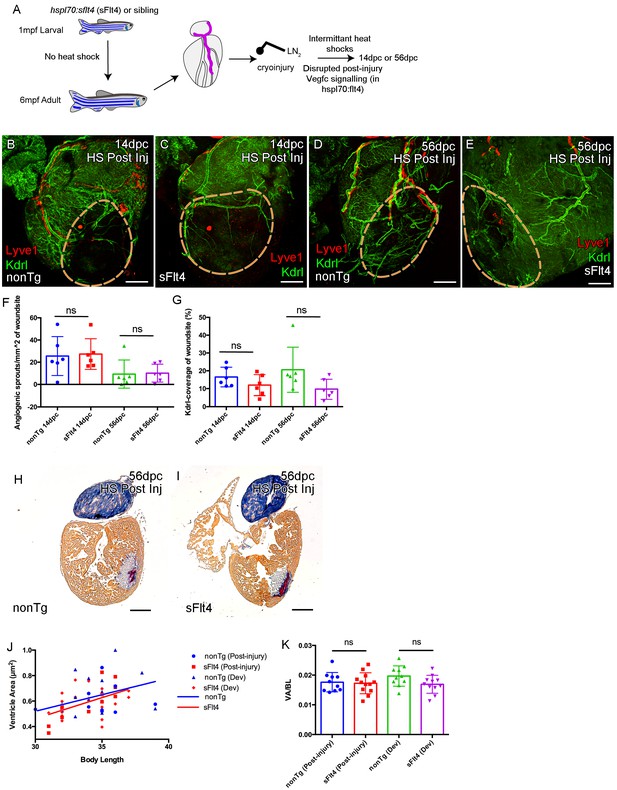
Induction of sFlt4 after cryoinjury has no significant effect on coronary vessels or heart morphology.
(A) Schematic representation of the experimental inhibition of post-injury signaling through lymphatic endothelial receptor Flt4. Whole-mount confocal (B–E) and brightfield AFOG-histology imaging (H and I) of post-injury heat shocked non-transgenic (B, D, H), hsp70l:sflt4 (C, E, I) adult transgenic zebrafish hearts that express arterially and endocardially enriched endothelial marker kdrl:GFP (green: B-E), lymphatic endothelial marker lyve1:RFP (red: B-E). (B and C) Hearts from both hsp70l:sflt4and non-transgenic post-injury heat shocked zebrafish show angiogenic sprouts and formed vessels within the woundsite (demarked in orange) at 14dpc. (D and E) Hearts from both hsp70l:sflt4 and non-transgenic post-injury heat shocked zebrafish show increased coronary vessel coverage by 56dpc. (F) Quantification of normalized coronary vessel angiogenic sprouts at 14 and 56dpc in the wound site of hearts from both hsp70l:sflt4 and non-transgenic post-injury heat shocked zebrafish. (G) Quantification of coronary vessel coverage of the wound site at 14 and 56dpc in hearts from both hsp70l:sflt4 and non-transgenic post-injury (HS 242dpf, cryoinjury 244dpf) heat shocked zebrafish. (H and I) Example images of largest AFOG stained tissue section used for ventricle quantification. (J) Linear regression analysis showing increasing max ventricle area to body length of AFOG-stained nonTg and hsp70l:sflt4 zebrafish heart sections with either post-injury heat shock (14 and 56dpc, HS from 242dpf, cryoinjured at 244dpf, see A) or developmental heat shock (Dev; 21dpc or 60dpc, HS from 35 to 205dpf or 71dpf to 229dpf, cryoinjury at 206 or 230dpf, see Figure 6D). (K) Quantification of max ventricle area (VA) to body length (BL) of nonTg and hsp70l:sflt4 zebrafish (post-injury HS: 14 and 56dpc and developmental (Dev) HS: 21 and 60dpc) showing no significant effect on post injury ventricle size with sFlt4 induction.
-
Figure 6—figure supplement 3—source data 1
Source data for Supplement 3(F) and (G).
- https://cdn.elifesciences.org/articles/42762/elife-42762-fig6-figsupp3-data1-v2.xlsx
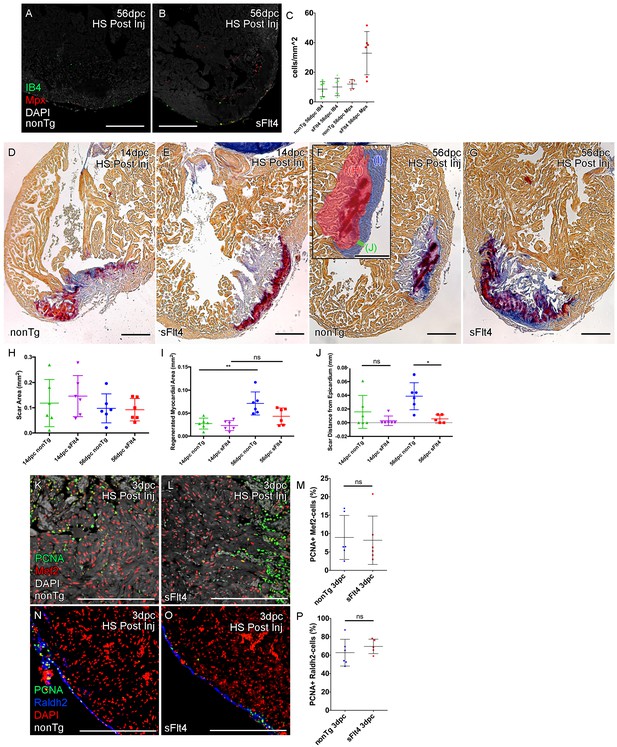
Post-injury induction of sFlt4 limits myocardial wall expansion and scar internalization.
(A–C) Imaged sections of a 56dpc hearts immune-labeled for macrophage (IB4, green) and neutrophil (Mpx, Red). Hearts were heart shocked just prior to injury and during regeneration to induce sFlt4 (B) or control for this induction (A). (C) Separate quantification of Mpx and IB4-positive cells in and within 100 μm of the woundsite (average of at least three images through woundsite, n = 6 per group). There is no difference in macrophage, but elevation of neutrophils is observed in some sFlt4-induced hearts despite the late stage (4/6), however this elevation across the group is not significantly different from the control. (D–G) AFOG-histology images showing fibrin in red and collagen in blue in post-injury heat shocked (non-transgenic (nonTg; D and F) and transgenic (hsp70l:sflt4; sFlt4; E and G) zebrafish 14 (D and E) and 56 days following cryoinjury (F and G). There is persistence of scar tissue even in nonTg zebrafish, but regeneration of cardiac myocardial wall in significantly reduced with induction of sFlt4. (F, insert) Quantified regions of the wound area. (H) Quantification of scar area (red, F insert; largest sectioned scar area, threshold to select tissue) shows no significant reduction in both nonTg and sFlt4 zebafish. (I) Quantification of the regenerated myocardial area (blue, F insert; thickened myocardial wall proximal to the scar) shows significant increase nonTg zebrafish between 14dpc and 56dpc, but no such increase is observed with sFlt4 induction (n = 6; **p=0.0022) (J) Quantification of the minimum distance of scar tissue from the epicardium (green, F insert; scar internalization with regeneration of the myocardial wall) is significantly larger nonTg zebrafish compared to sFlt4 induced siblings (n = 6, *p=0.0152; one outlier removed). (K and L) Immunostaining of PCNA and Mef2 showing cardiomyoctye proliferation 3 day post cryoinjury. (M) Quantification of PCNA/Mef2c-positive cells shows no effect on the induction of cardiomyocyte proliferation with expression of sFlt4 (average of at least three images through wounded region, double positive and Mef2c-only cells counted within 500 μm2 window proximal to woundsite, n = 6 per group). (N and O) Immuno-staining of PCNA and Raldh2 showing epicardial proliferation 3 day post cryoinjury. (P) Quantification of PCNA/Raldh2-positive cells shows no inhibition of epicardium activation with induction of sFlt4 (average of at least three images through wounded region all double positive and Raldh2-only cells within the entire 708 μm2 imaging window were counted, n = 6 per group). Scale bars 200 μm.
-
Figure 6—figure supplement 4—source data 1
Source data for Supplement 4(H-J).
- https://cdn.elifesciences.org/articles/42762/elife-42762-fig6-figsupp4-data1-v2.xlsx
-
Figure 6—figure supplement 4—source data 2
Source data for Supplement 4(M).
- https://cdn.elifesciences.org/articles/42762/elife-42762-fig6-figsupp4-data2-v2.xlsx
-
Figure 6—figure supplement 4—source data 3
Source data for Supplement 4(P).
- https://cdn.elifesciences.org/articles/42762/elife-42762-fig6-figsupp4-data3-v2.xlsx
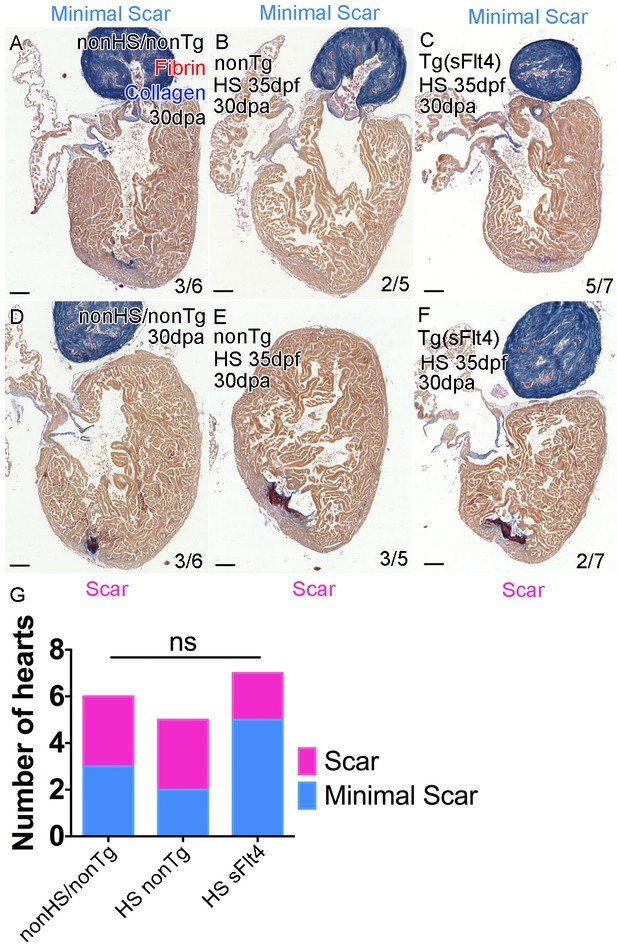
Loss of lymphatic vessels does not effect scar formation after amputation injury.
AFOG-histology images showing fibrin in red and collagen in blue in developmentally heat shocked (HS; 35 to 206dpf; B, C, E, F) or non-heat shocked (nonHS; A, D) non-transgenic (nonTg; A, B, D, E) and transgenic (hsp70l:sflt4; sFlt4; C and F) zebrafish 30 days (237dpf) following apex resection injury (30dpa, 8mpf). In all conditions at 30dpa, some hearts show little or no scar tissue (A-C) while other hearts of the same batch have detectable scar tissue still (D-F). (G) Graph showing the non-significant distribution (ns, Chi-Squared) of the presence or absence of scar tissue (examples images, A-F) between non-transgenic control (both nonHS, n = 6, and HS, n = 5) and transgenic (hsp70l:sflt4, n = 7) zebrafish at 30dpa. Scale bars 200 μm.
Tables
Table of antibodies used.
Antigen | Species | Concentration | Supplier |
---|---|---|---|
Mpx | Rabbit | 1:100 | GeneTex, GTX128379 |
IB4 (conjugated protein) | n/a (Griffonia Simplicifolia) | 1:100 | Vector Laboratories, DL-1207 |
GFP | Chicken | 1:500 | Aves Labs, GFP-1010 |
Rabbit IgG-647 | Goat | 1:500 | ThermoFisher Scientific, A21245 |
Mouse IgG-488 | Goat | 1:500 | ThermoFisher Scientific, A11001 |
Chicken IgG-FITC | Goat | 1:500 | Aves Labs, F1005 |
Raldh2 | Rabbit | 1:200 | GeneTex, GTX124302 |
Mef2c | Rabbit | 1:200 | Santa Cruz, sc-313 |
PCNA | Mouse | 1:200 | Vector Labs, VP-P980 |