Regulation of pulmonary surfactant by the adhesion GPCR GPR116/ADGRF5 requires a tethered agonist-mediated activation mechanism
Figures
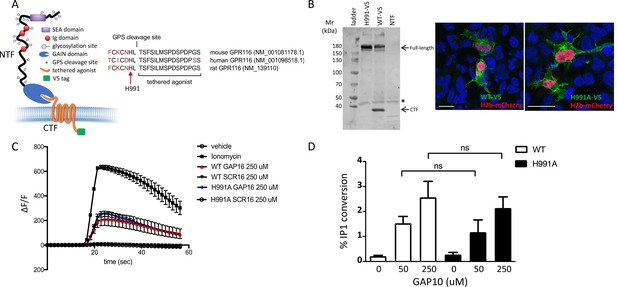
Generation and validation of a non-cleavable GPR116 mutant H991A.
(A) Schematic representation of the H991A mutation in GPR116, details of the sequence at the protein level and gene references. (B) Transient expression of V5-tagged H991A in HEK293 cells shows no cleavage at the GPS site by Western blot compared to the wild-type receptor; membrane localization by V5 immunocytochemistry shows similar localization of WT-V5 and H991A-V5. NTF, non-transfected control. Asterisk denotes non-specific band. Scale bars = 25 µm. See also Figure 1—source data 1. (C, D) Functional characterization of mouse GPR116 H991A in calcium transient assays (C) and in IP accumulation assays (D), using GAP16, GAP10, or scrambled peptide (SCR16) as the stimulus (n = 3 independent experiments performed with n = 1 technical replicate for C and n = 2 technical replicates per group for D). Data are expressed as mean ± SD (one-way ANOVA for C and D). ns, not significant.
-
Figure 1—source data 1
Transient expression of V5-tagged H991A in HEK293 cells analyzed by Western blot; original image.
- https://cdn.elifesciences.org/articles/69061/elife-69061-fig1-data1-v2.pptx
-
Figure 1—source data 2
Transient expression of V5-tagged H991A in HEK293 cells analyzed by Western blot; uncropped image.
- https://cdn.elifesciences.org/articles/69061/elife-69061-fig1-data2-v2.pdf
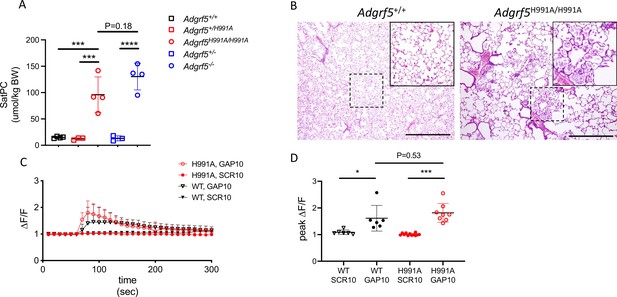
Cleavage at the GPS site is required for GPR116 function in vivo.
(A) Content of saturated phosphatidylcholine (SatPC) in the bronchoalveolar lavage fluid (BALF) of 8-week-old wild-type (GPR116+/+), GPR116+/H991A, GPR116H991A/H991A (line 2552), GPR116+/-, and GPR116-/- mice (n = 3–4 mice per group). Data are expressed as mean ± SD (one-way ANOVA). ***p<0.001, ****p<0.0001. (B) Representative histology of 4.5-month-old wild-type and homozygous H991A knock-in mice. Note accumulation of pulmonary surfactant (inset) and alveolar simplification in H991A knock-in mice compared to wild-type control. Scale bars = 500 µm. (C) GAP10-induced calcium transients in primary AT2 cells of GPR116+/+ and GPR116H991A/H991A mice (n = 3–4 independent experiments, n = 3 biological replicates per group). Data are expressed as mean ± SD. (D) Peak calcium responses in primary GPR116+/+ (WT) and GPR116H991A/H991A AT2 cells treated with SCR10 or GAP10 (n = 3 independent experiments, with n = 2–3 technical replicates per group). Data are expressed as mean ± SD (one-way ANOVA). *p<0.01, ***p<0.001.
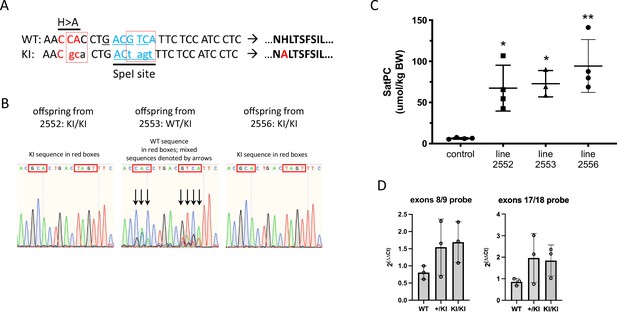
Schematic of H991A point mutation introduced into the Adgrf5 locus via CRISPR/Cas9 gene editing.
(A) Red boxes denote introduced base pair changes in the Adgrf5 locus. SpeI restriction enzyme recognition site was introduced for screening purposes without altering amino acid sequence at this position. (B) Representative sequencing traces of genomic DNA from two homozygous (2552 and 2556) and one heterozygous H991A line (2553). Line 2553 was bred to homozygosity for experiments. (C) Content of saturated phosphatidylcholine (SatPC) in the bronchoalveolar lavage fluid (BALF) of 4-week-old wild-type control and H991A homozygous knock-in mice (n = 3–4 mice per group). (D) qPCR analysis of GPR116 expression in isolated AT2 cells from WT, line 2552 H991A KI heterozygous mice (+/KI) and line 2552 H991A homozygous mice (KI/KI). Each data point represents a cell preparation from an individual animal. Data are expressed as mean ± SD (one-way ANOVA). **p<0.005, *p<0.05.
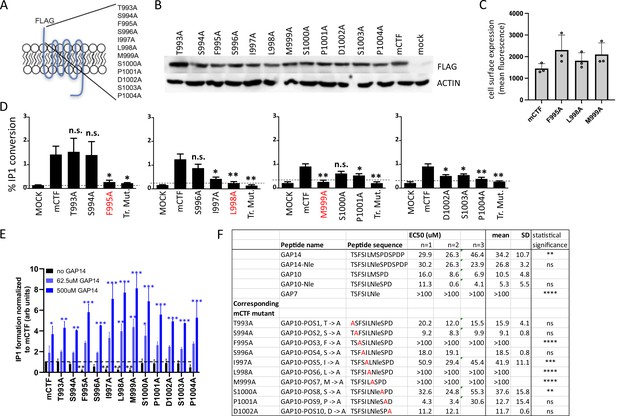
Identification of amino acids in the tethered agonist essential for GPR116 activity.
(A) Design of the 12 mGPR116 CTF (mCTF) ECD mutants. Alanine scan of individual residues in the N-terminal sequence. (B) Expression of the mCTF ECD mutants transiently expressed in HEK293 cells. Constructs were detected by Western blot of whole-cell lysates using an anti-FLAG antibody. Moledular weight of FLAG ~43kDa; molecular weight of actin ~42kDa. See also Figure 3—source data 1 and 2. (C) Quantitation of cell surface expression of mCTF ECD mutants by anti-FLAG antibody staining and flow cytometry of non-permeabilized cells. (D) Signaling of the mGPR116 CTF ECD mutants. Constructs of the ECD alanine scan and corresponding triple mutants were transiently expressed in HEK293 cells and basal activity was measured as % IP1 conversion (n = 4–5 independent experiments, with n = 2 technical replicates per group). Dashed line indicates background activity as reference. Data are expressed as mean ± SD. **p<0.01, *p<0.05. The three single-point mutants with the most potent inhibitory effect are highlighted in red. Tr. Mut., triple mutant. (E) Exogenous GAP14 treatment rescues mCTF ECD mutants with inactive tethered agonist and super-activates constructs exhibiting basal activity. Constructs were transiently expressed in HEK293 cells and stimulated with GAP14. IP1 accumulation was measured in n = 2–3 independent experiments with n = 2–4 replicates to evaluate basal and GAP14-induced activity of the receptor, respectively. Data are expressed as mean ± SD. Basal activity of mCTF (dashed line) was used as the reference. Basal or GAP14-induced activities significantly different from mCTF baseline are marked with black or blue stars, respectively. *p<0.05, **p<0.01, ***p<0.001. (F) Activation of full-length mGPR116 in stable expressing cells (HEK293 clone 3C) with exogenous GAP10 peptides that were sequentially mutated to alanine at each position. Receptor activation was measured via calcium transient assays, in n = 2–3 independent experiments, with n = 4 technical replicates per group. Quadruplicate means of each experiment and final mean ± SD are detailed. **p<0.01, ***p<0.001, ****p<0.0001. See also Figure 3—source data 3.
-
Figure 3—source data 1
Expression of the mCTF ECD mutants detected by Western blot using an anti-FLAG antibody; original image.
- https://cdn.elifesciences.org/articles/69061/elife-69061-fig3-data1-v2.pdf
-
Figure 3—source data 2
Expression of the mCTF ECD mutants detected by Western blot using an anti-FLAG antibody; uncropped image.
- https://cdn.elifesciences.org/articles/69061/elife-69061-fig3-data2-v2.pdf
-
Figure 3—source data 3
Activation of FL mGPR116 with exogenous GAP10 peptides that were sequentially mutated to alanine at each position.
- https://cdn.elifesciences.org/articles/69061/elife-69061-fig3-data3-v2.docx
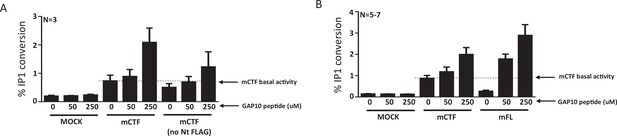
Super-activation of mGPR116 C-terminal fragment (CTF) constructs with basal activity.
(A) The N-terminal FLAG tag in the mCTF constructs does not affect the activation by GAP10. Tagged and untagged mCTF were transiently expressed in HEK293 cells and GAP10-induced IP1 conversion was measured. Dotted line indicates mCTF basal activity; n = 3 independent experiments, with n = 2 technical replicates per group. Data are expressed as mean ± SD. (B) The mCTF construct, expressed transiently in HEK293 cells, shows basal activity and can be super-activated to levels approximating that of the full-length receptor (mFL) upon addition of GAP10. Receptor signaling is measured in the IP1 conversion assay; n = 5–7 independent experiments with n = 3 technical replicates per group. Data are expressed as mean ± SD.
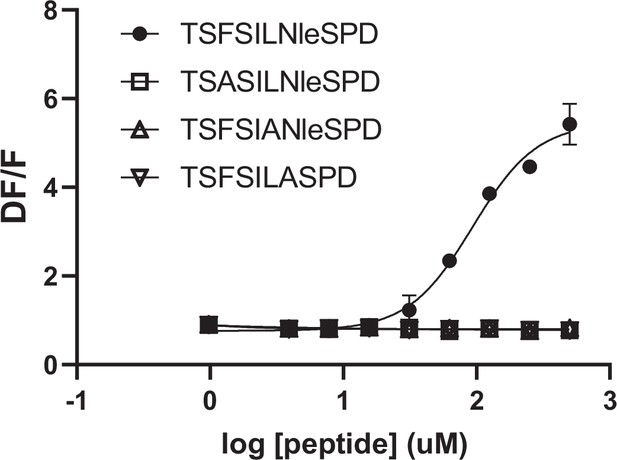
Alanine mutation of key amino acids in GAP10 inactivates the peptide for human GPR116 full-length stimulation.
Full-length hGPR116 (HEK293 clone A6) stimulated with GAP10 and peptide variants corresponding to alanine changes identified in mGPR116 as being critical for the activation by the tethered agonist (n = 5–7 independent experiments, with n = 2 technical replicates per group). Data are expressed as mean ± SD. See Supplementary file 2 for EC50 and statistical details.
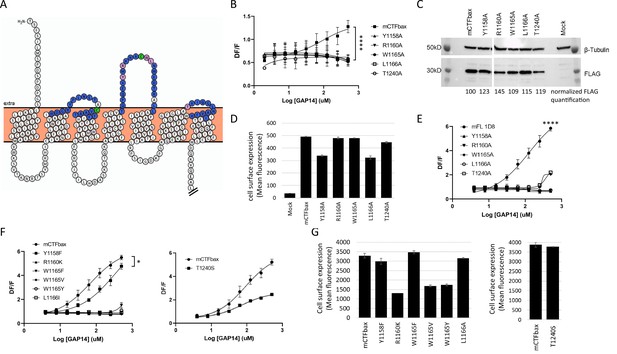
Identification of key extracellular loop (ECL) amino acids involved in GPR116 activation by the tethered agonist.
(A) Snake plot model of mouse GPR116 C-terminal fragment (CTF). ECL residues individually mutated in the alanine scan of mouse GPR116 CTF constructs tested for functional activity are colored in blue or pink; the five residues shown in pink were identified as strongly modulating receptor activity. The two cysteine residues forming the disulfide bridge are shown in green. The Ct tail was truncated for visualization purposes as indicated by double hash lines. (B) Alanine scan of ECLs in mCTFbax, a CTF construct without basal activity. Calcium transient assays were performed in transiently transfected HEK293 cells with exogenous GAP14 as the stimulus. mCTFbax mutants that were completely inactive following GAP14 stimulation are shown. Data are from n = 3 independent experiments, with n = 4 technical replicates per group. Data are expressed as mean ± SD (****p<0.0001 for the reference construct vs. mutants). See Figure 4—figure supplement 1A for further alanine scan data, including mutants with no effect. See Supplementary file 2 for potency and statistical details. (C, D) Expression of the mCTFbax mutants not activated by GAP14, in transiently transfected HEK293 cells. (C) Proteins were detected by Western blot using an anti-FLAG antibody. For quantification, levels of FLAG signal were normalized to tubulin expression and expressed as % of mCTFbax levels. See also Figure 4—source data 1–3. (D) Surface levels of receptor expression was measured by flow cytometry using an anti-FLAG antibody on non-permeabilized cells. Data are expressed as mean ± SD from an experiment with duplicates. (E) GAP14-induced calcium transients of mFL mutants for five key ECL residues, stably expressed HEK293 cells, as compared to the WT mGPR116 1D8 clone. Data shown are from n = 3 independent experiments with n = 4 technical replicates per group. Data are expressed as mean ± SD (****p<0.0001 for the reference construct vs. mutants). See Supplementary file 2 for potency and statistical details. (F) Mutation of key ECL residues in the mCTFbax construct to amino acids with functional relevance. mCTFbax and mutants thereof were stably expressed in HEK293 cells and GAP14-induced calcium transients were measured. Data are from n = 3 independent experiments, with n = 4 technical replicates per group. Data are expressed as mean ± SD (*p<0.05). See Supplementary file 2 for potency and statistical details. (G) Surface levels of receptor expression for mCTFbax mutants in HEK293 stable populations were measured by flow cytometry using an anti-FLAG antibody. Data are expressed as mean ± SD from an experiment with duplicates.
-
Figure 4—source data 1
Expression of the mCTFbax mutants not activated by GAP14, detected by Western blot using an anti-FLAG antibody; original image.
- https://cdn.elifesciences.org/articles/69061/elife-69061-fig4-data1-v2.pdf
-
Figure 4—source data 2
Expression of the mCTFbax mutants not activated by GAP14, Western blot of the tubulin control; original image.
- https://cdn.elifesciences.org/articles/69061/elife-69061-fig4-data2-v2.pdf
-
Figure 4—source data 3
Expression of the mCTFbax mutants not activated by GAP14, detected by Western blot using an anti-FLAG antibody; uncropped images.
- https://cdn.elifesciences.org/articles/69061/elife-69061-fig4-data3-v2.pptx
-
Figure 4—source data 4
Alignment of the human and mouse GPR116 CTF sequences.
- https://cdn.elifesciences.org/articles/69061/elife-69061-fig4-data4-v2.docx
-
Figure 4—source data 5
Expression of hFL WT and mutants, detected by Western blot using an anti-V5 antibody; original image.
- https://cdn.elifesciences.org/articles/69061/elife-69061-fig4-data5-v2.pdf
-
Figure 4—source data 6
Expression of hFL WT and mutants, detected by Western blot using an anti-V5 antibody; uncropped image.
- https://cdn.elifesciences.org/articles/69061/elife-69061-fig4-data6-v2.pptx
-
Figure 4—source data 7
Expression of mCTFbax mutants, analyzed by Western blot using the FLAG tag; original image.
- https://cdn.elifesciences.org/articles/69061/elife-69061-fig4-data7-v2.pdf
-
Figure 4—source data 8
Expression of mCTFbax mutants; Western blot of the tubulin control; original image.
- https://cdn.elifesciences.org/articles/69061/elife-69061-fig4-data8-v2.pdf
-
Figure 4—source data 9
Expression of mCTFbax mutants, analyzed by Western blot using the FLAG tag; uncropped images.
- https://cdn.elifesciences.org/articles/69061/elife-69061-fig4-data9-v2.pptx
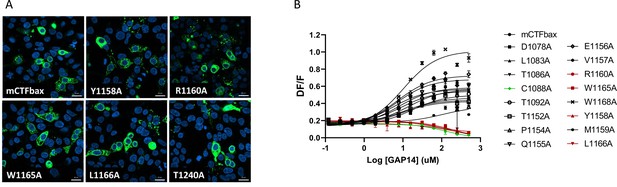
Alanine scan of GPR116 C-terminal fragment (CTF) extracellular loops (ECLs).
(A) Expression of the mCTFbax mutants not activated by GAP14, in transiently transfected HEK293 cells; detection by V5 tag immunocytochemistry (scale bars = 20 um). (B) Representative data from mutants with or without functional effect. Calcium transient assays were performed in transiently transfected HEK293 cells with exogenous GAP14 as the stimulus. mCTFbax mutants with varying effects of the mutation are shown. Mutants exhibiting no activity upon GAP14 stimulation and selected for further analysis are highlighted in red, and the construct with one of the cysteines from the disulfide bridge mutated to alanine is in green. Data are from n = 1 experiment, with n = 4 technical replicates per group. Data are expressed as mean ± SD.
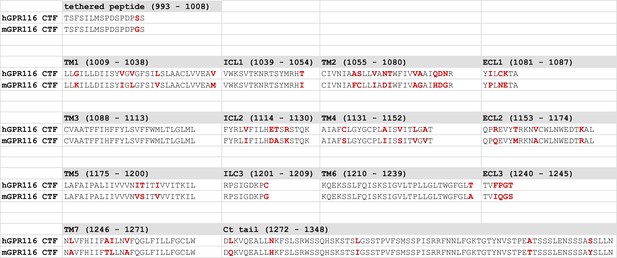
Alignment of the human and mouse GPR116 C-terminal fragment (CTF) sequences.
Residues that are not conserved between the two species are highlighted in red and bold.
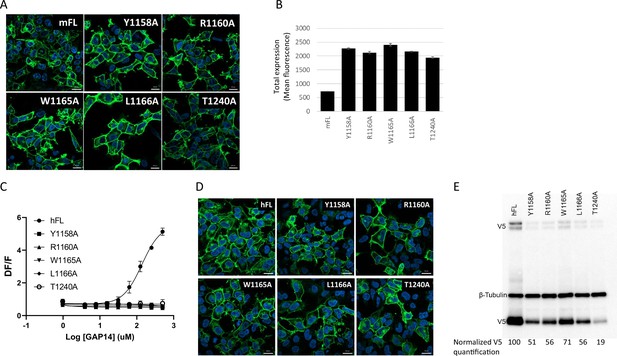
Mutation of the key extracellular loop (ECL) amino acids in mGPR116 full-length (mFL) WT (clone 1D8) and ECL mutants, as well as in human GPR116 full-length leads to inactive constructs.
(A) Expression of the mGPR116 full-length (mFL) WT (clone 1D8) and ECL mutants assessed by V5 tag immunocytochemistry upon stable expression in HEK293 (scale bars = 20 um). (B) Expression of mFL ECL mutants stably expressed in HEK293 cells. Total expression levels were quantified in permeabilized cells by flow cytometry using an anti-V5 antibody. Data are expressed as mean ± SD from an experiment with duplicates. (C) Activity of the full-length hGPR116 mutated for key ECL2/TM6 residues compared to the WT hGPR116 A6 clone (hFL). Mutants were stably expressed in HEK293 cells and GAP14-induced calcium transients were measured. Representative data of n = 3 independent experiments with n = 4 technical replicates per group. Data are expressed as mean ± SD. See Supplementary file 2 for potency and statistical details. (D) Expression of hFL WT (clone A6) and mutants was tested as HEK293 stable populations by V5 tag immunocytochemistry (scale bars = 20 um). (E) Expression of hFL WT (clone A6) and mutants in stably transfected HEK293 cells. Constructs were detected by Western blot using an anti-V5 antibody. The major band ( ~43 kDa) corresponds to the cleaved C-terminal fragment (CTF) and the minor bands at high molecular weight (~185 kDa) correspond to the full-length receptor. The levels of CTF V5 signal were quantified, normalized to tubulin expression (~50 kDa) and expressed as % of mCTFbax levels. See also Figure 4—source data 5 and 6.
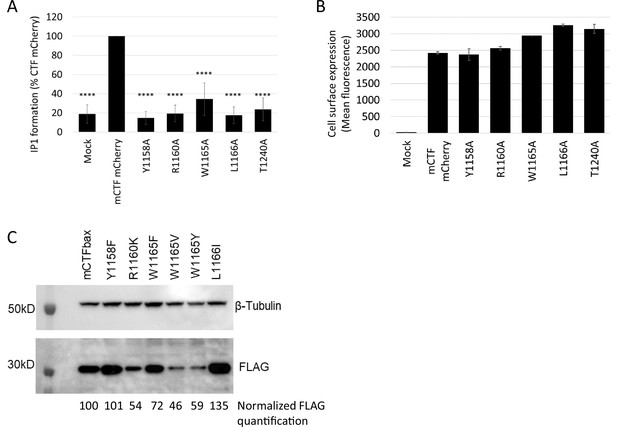
Alanine mutation of key extracellular loop (ECL) residues in mGPR116 C-terminal fragment (CTF) constructs with basal activity and non-conservative mutations in mCTFbax.
(A) Basal activity of ECL mutants in the mCTF mCherry parent construct. ECL residues important for activation were mutated to alanine and IP1 formation was measured in transiently transfected HEK293 cells. Data, normalized to the parent construct, are expressed as mean ± SD (n=8 independent experiments, with n = ? (Marie?) technical replicates per group; ****p<0.0001). (B) mCTF mCherry constructs were transiently expressed in HEK293 cells, and expression was evaluated by flow cytometry analysis of the mCherry signal. Data are expressed as mean ± SD from an experiment with duplicates. (C) Expression of mCTFbax mutants in HEK293 stable populations was analyzed by Western blot using the FLAG tag. For quantification, levels of FLAG signal were normalized to tubulin expression and expressed as % of mCTFbax levels. See also Figure 4—source data 7–9.
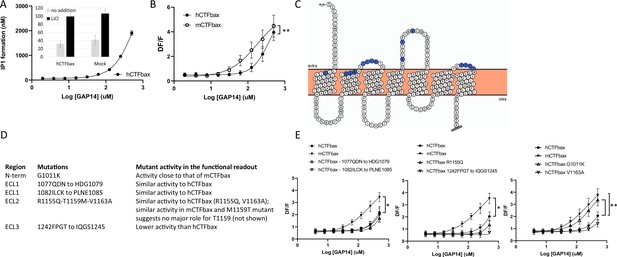
Evaluation of the role of extracellular loop (ECL) amino acids not conserved between human and mouse GPR116.
(A) Characterization of hCTFbax, a C-terminal fragment (CTF) construct without basal activity and that can be activated by GAP14. The hCTF with deletion of the N-terminal six amino acids was stably expressed in HEK293 cells and stimulated with GAP14 in an IP1 accumulation assay (n = 3 independent experiments, with n = 2 technical replicates per group). Data are expressed as mean ±-SD. The basal level of IP1 formation in presence of LiCl is similar to that of parental HEK cells (inset). (B) Stable HEK293 populations for mCTFbax and hCTFbax were stimulated with GAP14 and analyzed for induction of calcium transients. Data from n = 3 independent experiments, with n = 2 technical replicates per group. Data are expressed as mean ± SD (**p<0.01). See Supplementary file 2 for potency and statistical details. (C) Overview of the mouse-specific residues introduced in the hCTFbax construct. Snake plot of the human GPR116 CTF (including the full tethered agonist sequence); amino acids highlighted in blue, located towards to extracellular side, are not conserved between human and mouse GPR116. The corresponding mouse sequence was introduced in human GPR116. The Ct tail was truncated for visualization purposes as indicated by double hash lines. (D) Details of the residues exchanged in the hCTFbax with the corresponding mouse sequence. Each cluster of changes was tested for its signaling capacity and the functional assay outcome is described. (E) GAP14-induced calcium transients were not further increased in the mutants as compared to the reference construct hCTFbax with the exception of G1011K (right panel). Constructs were stably expressed in HEK293 cells and tested with n = 2–4 technical replicates (n = 4–5 independent experiments; data are expressed as mean ± SD; *p<0.05, **p<0.01). See Supplementary file 2 for potency and statistical details.
-
Figure 5—source data 1
Expression of hCTFbax and mutants thereof, detected by Western blot using an anti-V5 antibody; original image.
- https://cdn.elifesciences.org/articles/69061/elife-69061-fig5-data1-v2.pdf
-
Figure 5—source data 2
Expression of hCTFbax and mutants thereof, detected by Western blot using an anti-V5 antibody; uncropped image.
- https://cdn.elifesciences.org/articles/69061/elife-69061-fig5-data2-v2.pptx
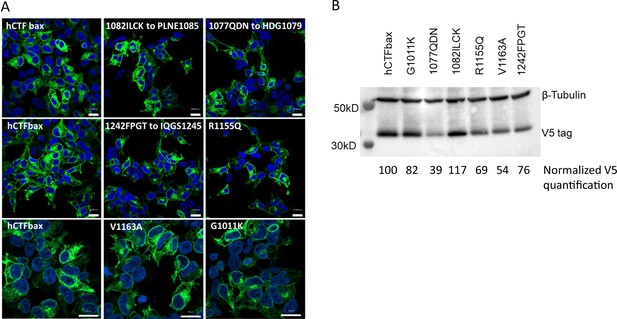
Evaluation of the role of extracellular loop (ECL) and N-terminus amino acids not conserved between human and mouse GPR116 C-terminal fragment (CTF).
(A) Expression of hCTFbax mutants as HEK293 stable populations was tested by V5 tag immunocytochemistry (scale bars = 20 um). (B) Expression of hCTFbax and mutants thereof in stably transfected HEK293 cells. Constructs were detected by Western blot using an anti-V5 antibody. For quantification, levels of V5 signal were normalized to tubulin expression and expressed as % of hCTFbax levels. See also Figure 5—source data 1 and 2.
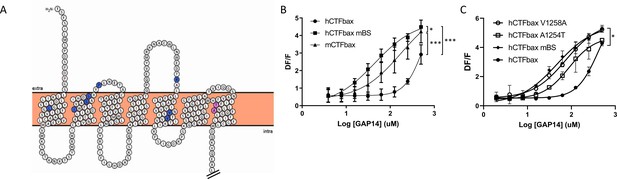
Engineering the predicted mouse GPR116 binding site (mBS) into human GPR116 leads to increased peptide activation.
(A) Snake plot of the mouse GPR116 CTF highlighting mouse-specific amino acids in the putative binding site in blue and pink. Amino acids responsible for mGPR116-specific aspects of receptor activation are shown in pink. The Ct tail was truncated for visualization purposes as indicated by double hash lines. (B) Stable HEK293 populations for m/hCTFbax and hCTFbax mBS were stimulated with GAP14 and analyzed for the induction of calcium transients (n = 3 independent experiments, with n = 2 technical replicates per group; data are expressed as mean ± SD; *p<0.05, ***p<0.001). See Supplementary file 2 for potency and statistical details. (C) Characterization of the key amino acids transmitting the effects of the mouse binding site sequence. hCTFbax single mutants were compared to hCTFbax and hCTFbax mBS in calcium transient assays. Stable HEK293 populations were stimulated with GAP14 (n = 3 independent experiments, with n = 2 duplicates per group; data are expressed as mean ± SD; *p<0.05 between hCTFbax and hCTFbax mBS). See Supplementary file 2 for potency and statistical details.
-
Figure 6—source data 1
Expression of hCTFbax and related hCTFbax murine-specific binding site (mBS) A1254T and V1258A mutants; Western blot analysis using an anti-V5 antibody; original image.
- https://cdn.elifesciences.org/articles/69061/elife-69061-fig6-data1-v2.pdf
-
Figure 6—source data 2
Expression of hCTFbax and related hCTFbax murine-specific binding site (mBS) A1254T and V1258A mutants; Western blot analysis using an anti-V5 antibody; uncropped image.
- https://cdn.elifesciences.org/articles/69061/elife-69061-fig6-data2-v2.pptx
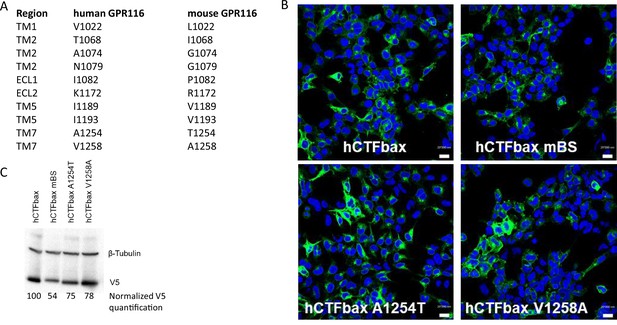
Details of the putative binding site and expression of corresponding mutants.
(A) Amino acids defining the potential binding site in mouse GPR116 (mBS). Corresponding residues in human GPR116 are given. (B, C) Expression of hCTFbax, the related hCTFbax mBS, A1254T, and V1258A mutants stably expressed in HEK293 cells. (B) The C-terminally tagged constructs are detected by immunocytochemistry using an anti-V5 antibody (scale bars = 20 um). (C) Western blot of the corresponding constructs using an anti-V5 antibody and quantification; levels of V5 signal were normalized to tubulin expression and expressed as % of hCTFbax levels. Molecular weight of V5 ~43kDA; molecular weight of tubulin ~50kDa. See also Figure 6—source data 1 and 2.
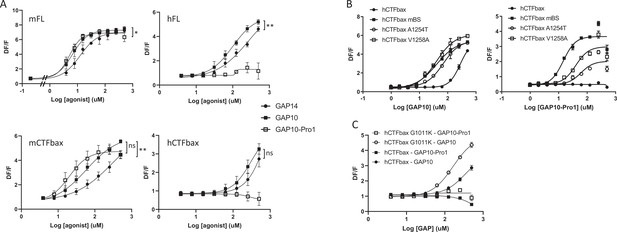
Characterization of a mouse-specific agonistic peptide.
(A) GAP10-Pro1 only activates mouse GPR116. Full-length mouse and human GPR116 (mFL clone 3C and hFL clone A6, respectively), mCTFbax and hCTFbax, all stably expressed in HEK293 cells, were stimulated with GAP14, GAP10, and GAP10-Pro1. Calcium transients are measured as a signaling readout in n = 3–4 independent experiments, with n = 2–4 technical replicates per group. Data are expressed as mean ± SD (*p<0.05, **p<0.01). See Supplementary file 2 for potency and statistical details. (B) Identification of the key amino acids mediating the mouse-specific effects in the mouse binding site. The hCTFbax construct, the hCTFbax mBS chimera, and the single-point mutants A1254T and V1258A in hCTFbax were stimulated with GAP10 or GAP10-Pro1 upon stable expression in HEK293 cells. Activation was measured in calcium transient assays, in n = 2 technical replicates. Data, expressed as mean ± SD, represent n = 4 independent experiments, with n = 2–4 technical replicates per group. See Supplementary file 2 for potency and statistical details. (C) Calcium transients evoked in HEK293 cells stably expressing hCTFbax or the mutated construct G1011K upon stimulation with GAP10 or GAP10-Pro1. Data represent n = 4 independent experiments, with n = 3 technical replicates per group. Data are expressed as mean ± SD. See Supplementary file 2 for potency and statistical details.
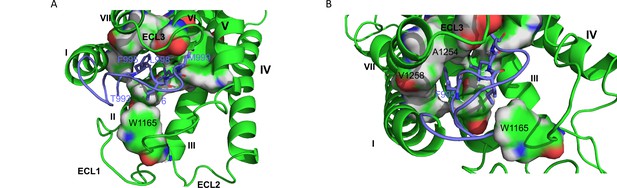
hGPR116 7TM homology model.
(A) Binding model of the tethered agonist peptide (T993-L1010, C atoms in purple) to the transmembrane domain of human GPR116 homology model (C atoms in green). For clarity, only the four amino acids of the tethered agonist peptide that form most of the interactions with the transmembrane domain of GPR116 are displayed as sticks (F995, I997, L977, and M999). The amino acids of GPR116 transmembrane domain that interact with the four amino acids of the tethered agonist peptide mentioned above are displayed as surface color-coded by atom type (C in green, H in gray, N in blue, and O in red). (B) Zoom on A1254 and V1258, which form van der Waals contacts with F995.
-
Figure 8—source data 1
Homology model of human GPR116 7TM.
- https://cdn.elifesciences.org/articles/69061/elife-69061-fig8-data1-v2.txt
Tables
Reagent type (species) or resource | Designation | Source or reference | Identifiers | Additional information |
---|---|---|---|---|
Gene (Mus musculus) | Adgrf5 | GenBank | NM_001081178.1 | |
Gene (Homo sapiens) | ADGRF5 | GenBank | NM_001098518.1 | UniProt Q8IZF2-1 |
Genetic reagent (M. musculus) | Adgrf5 H991A | This paper | NM_001081178.1 | Germline knock-in of H991A mutation |
Genetic reagent (M. musculus) | Adgrf5-/- | PMID:28570277 | NM_001081178.1 | Germline knock-out |
Cell line (H. sapiens) | HEK293; HEK293T; HEK293H | ATCC; ATCC Gibco | CRL-1573; CRL-3216; 11631-017 | |
Sequence-based reagent | sgRNA #1 Adgrf5 locus (M. musculus) | This paper | TGAGGATGGAGAATGACGTC | |
Sequence-based reagent | PCR primers Adgrf5 WT allele (M. musculus) | This paper | FWD-CCACCTGACGTCATTCTCCA; REV- GGCGCATATAGGAAGTTCGG | |
Sequence-based reagent | PCR primers Adgrf5 H991A allele (M. musculus) | This paper | FWD-CACACAGGCTGTTTCGTTGA; REV- CGCACTGACTAGTTTCTCCATC | |
Sequence-based reagent | PCR primers site-directed mutagenesis Y1158A (M. musculus) | This paper | FWD-CAGGAAGTCGCCATGAGGAAG; REV-CTTCCTCATGGCGACTTCCTG | |
Sequence-based reagent | PCR primers site-directed mutagenesis R1160A (M. musculus) | This paper | FWD-GTCTACATGGCGAAGAACGCG; REV-CGCGTTCTTCGCCATGTAGAC | |
Sequence-based reagent | PCR primers site-directed mutagenesis W1165A (M. musculus) | This paper | FWD-AACGCGTGTGCGCTCAACTGG; REV-CCAGTTGAGCGCACACGCGTT | |
Sequence-based reagent | PCR primers site-directed mutagenesis L1166A (M. musculus) | This paper | FWD-GCGTGTTGGGCCAACTGGGAG; REV-CTCCCAGTTGGCCCAACACGC | |
Sequence-based reagent | PCR primers site-directed mutagenesis T1240A (M. musculus) | This paper | FWD-GGTCTTGCCGCAGTGATCCAG; REV-CTGGATCACTGCGGCAAGACC | |
Sequence-based reagent | PCR primers site-directed mutagenesis Y1158F (M. musculus) | This paper | FWD-CAGGAAGTCTTCATGAGGAAG; REV-CTTCCTCATGAAGACTTCCTG | |
Sequence-based reagent | PCR primers site-directed mutagenesis R1160K (M. musculus) | This paper | FWD-GTCTACATGAAGAAGAACGCG; REV-CGCGTTCTTCTTCATGTAGAC | |
Sequence-based reagent | PCR primers site-directed mutagenesis W1165Y (M. musculus) | This paper | FWD-GCGTGTTACCTCAACTGGGAGG; REV-CCTCCCAGTTGAGGTAACACGC | |
Sequence-based reagent | PCR primers site-directed mutagenesis W1165V | This paper | FWD-CGCGTGTGTGCTCAACTGGGAGG; REV-CCTCCCAGTTGAGCACACACGCG | |
Sequence-based reagent | PCR primers site-directed mutagenesis W1165F (M. musculus) | This paper | FWD-AACGCGTGTTTTCTCAACTGG; REV-CCAGTTGAGAAAACACGCGTT | |
Sequence-based reagent | PCR primers site-directed mutagenesis L1166I (M. musculus) | This paper | FWD-GCGTGTTGGATCAACTGGGAG; REV-CTCCCAGTTGATCCAACACGC | |
Sequence-based reagent | PCR primers site-directed mutagenesis Y1158A (H. sapiens) | This paper | FWD-CGGGAAGTCGCTACGAGGAAGA; REV-TCTTCCTCGTAGCGACTTCCCG | |
Sequence-based reagent | PCR primers site-directed mutagenesis R1160A (H. sapiens) | This paper | FWD-GTCTATACGGCGAAGAATGTCTG; REV-CAGACATTCTTCGCCGTATAGAC | |
Sequence-based reagent | PCR primers site-directed mutagenesis W1165A (H. sapiens) | This paper | FWD-GAATGTCTGTGCGCTCAACTGG; REV-CCAGTTGAGCGCACAGACATTC | |
Sequence-based reagent | PCR primers site-directed mutagenesis L1166A (H. sapiens) | This paper | FWD-GTCTGTTGGGCCAACTGGGAGG; REV-CCTCCCAGTTGGCCCAACAGAC | |
Sequence-based reagent | PCR primers site-directed mutagenesis T1240A (H. sapiens) | This paper | FWD-GGTCTCACCGCTGTGTTCCCAGG; REV-CCTGGGAACACAGCGGTGAGACC | |
Sequence-based reagent | PCR primers site-directed mutagenesis A1254T (H. sapiens) | This paper | FWD-CATCATCTTCACTATCCTGAACG; REV-CGTTCAGGATAGTGAAGATGATG | |
Sequence-based reagent | PCR primers site-directed mutagenesis V1258A (H. sapiens) | This paper | FWD-CCATCCTGAACGCCTTCCAGGGCC; REV-GGCCCTGGAAGGCGTTCAGGATGG | |
Sequence-based reagent | PCR primers site-directed mutagenesis G1011K (H. sapiens) | This paper | FWD-CTCTCTGCTGAAGATCCTGCTGG; REV-CCAGCAGGATCTTCAGCAGAGAG | |
Sequence-based reagent | PCR primers site-directed mutagenesis 1077QDN to HDG1079 (H. sapiens) | This paper | FWD-GCCGCCATCCATGACGGCCGGTACATC; REV-GATGTACCGGCCGTCATGGATGGCGGC | |
Sequence-based reagent | PCR primers site-directed mutagenesis 1082ILCK to PLNE1083 (H. sapiens) | This paper | FWD-AACCGGTACCCCCTGAACGAGACCGCCTGC; REV-GCAGGCGGTCTTGCACAGGATGTACCGGTT | |
Sequence-based reagent | PCR primers site-directed mutagenesis R1153Q- T1157M- V1161A (H. sapiens) | This paper | FWD-CCAGCCCCAGGAAGTCTATATGA GGAAGAATGCGTGTTGGC; REV-GCCAACACGCATTCTTCCTCAT ATAGACTTCCTGGGGCTGG | |
Sequence-based reagent | PCR primers site-directed mutagenesis 1242FPGT to IQGS1245 (H. sapiens) | This paper | FWD-ACCACCGTGATCCAAGGCTCCAACCTGGTG; REV-CACCAGGTTGGAGCCTTGGATCACGGTGGT | |
Recombinant DNA reagent | H2b-mCherry | Addgene | #20972 | Transfection |
Peptide, recombinant protein | GAP14 | Peptides and Elephants | PMID:28570277 | TSFSILMSPDSPDP; TSFSILNleSPDSPDP |
Peptide, recombinant protein | GAP10; GAP10-Pro1 | Peptides and Elephants or Thermo Fisher | PMID:28570277; this paper | TSFSILNleSPD; PSFSILNleSPD |
Peptide, recombinant protein | GAP16 mouse | Thermo Fisher | PMID:28570277 | TSFSILMSPDSPDPSS |
Antibody | Anti-V5 mouse monoclonal | Invitrogen | R96025 | Immunocytochemistry; 1/500; Western blot 1/1000 |
Antibody | Anti-FLAG mouse monoclonal | Sigma | F1365, clone M2 | Immunocytochemistry, 1/100; Western blot, 1/1500 |
Antibody | Anti-mouse secondary antibody | Invitrogen | A21200 | Immunocytochemistry, 1/500; flow cytometry, 1/500 |
Antibody | Anti-mouse secondary antibody | Invitrogen | A11004 | Immunocytochemistry, 1/200 |
Antibody | Anti-V5 mouse monoclonal | Sigma | V8012 | Western blot, 1/1000 |
Antibody | Anti-beta tubulin rabbit monoclonal | Cell Signaling | 2128, clone 9 F3 | Western blot, 1/1000–1/5000 |
Antibody | Anti-actin mouse monoclonal | Seven Hills Bioreagents | Clone C4 | Western blot, 1/5000 |
Antibody | Anti-mouse secondary antibody | Jackson ImmunoResearch | 715-035-150 | Western blot, 1/5000–1/12,000 |
Antibody | Anti-rabbit secondary antibody | Jackson ImmunoResearch | 711-035-152 | Western blot, 1/12,000–1/15,000 |
Antibody | Anti-FLAG mouse monoclonal | Sigma | F1804 | Flow cytometry, 1/200; Western blot 1/1500 |
Antibody | Anti-mouse secondary antibody | Invitrogen | 12-4015-82 | Flow cytometry, 1/500 |
Commercial assay or kit | Metafectene-Pro, Lipofectamine 2000, PEI max | Biontex Invitrogen Polysciences | T040-0.2 11668019 24765-100 | Transfection |
Commercial assay or kit | Amaxa Cell Line Nucleofector Kit V | Lonza | VCA-1003 | Electroporation |
Commercial assay or kit | Cytofix/Cytoperm | BD | 51-2091KZ | |
Commercial assay or kit | Calcium 6 | Molecular Devices | R8191 | Calcium transients assay |
Commercial assay or kit | Fluo-4 AM | Thermo Fisher | F14201 | Calcium transients assay |
Commercial assay or kit | 3H-myo-inositol | PerkinElmer Life Sciences | NET1177001MC | Radiometric IP assay |
Commercial assay or kit | IP-One | Cisbio | 62IPAPEC | HTRF IP1 assay |
Software, algorithm | Maestro 2019-1 | Schrödinger | Modeling | |
Software, algorithm | Prism 9.2.0 | GraphPad | Statistics |
Additional files
-
Supplementary file 1
Alignment of the human aGPCR tethered agonist and ECL2 sequences.
Conserved residues mentioned in the text are highlighted in red and bold. Asterisks indicate aGPCRs for which GPS cleavage has not been shown to date.
- https://cdn.elifesciences.org/articles/69061/elife-69061-supp1-v2.docx
-
Supplementary file 2
Potency of the various constructs activated by GAPs.
EC50s, measured in the calcium transients assay, are given in µM. Number of data points, agonistic peptide used, mean ± SD, and statistical significance are detailed. n.d., not determined; ns, not significant.
- https://cdn.elifesciences.org/articles/69061/elife-69061-supp2-v2.docx
-
Transparent reporting form
- https://cdn.elifesciences.org/articles/69061/elife-69061-transrepform1-v2.docx