Distinct neurexin-cerebellin complexes control AMPA- and NMDA-receptor responses in a circuit-dependent manner
Figures
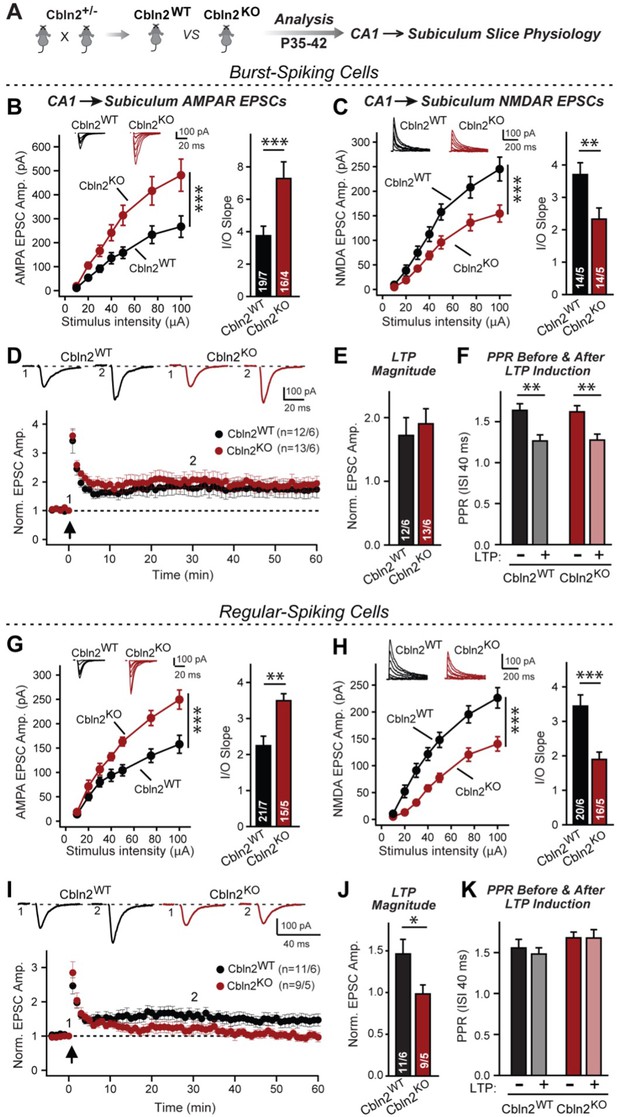
Constitutive Cbln2 deletion increases AMPAR-EPSCs and suppresses NMDAR-EPSCs at CA1→subiculum synapses formed on both burst- and regular-spiking subiculum neurons, and additionally blocks NMDAR-dependent LTP in regular-spiking neurons without affecting cAMP-dependent LTP in burst-spiking neurons.
(A) Experimental strategy for analysis of littermate wild-type and constitutive Cbln2 KO mice. (B) & (C) Input/output measurements of evoked AMPAR- and NMDAR-EPSCs recorded from burst-spiking neurons in acute subiculum slices reveal that the Cbln2 KO enhances AMPAR-EPSCs (B) but suppresses NMDAR-EPSCs (C). EPSCs were evoked by stimulation of CA1 axons in acute slices from littermate control and Cbln2 KO mice at P35-42 (left, summary plots of input-output curves with sample traces on top; right, summary graph of input/output slopes). (D–F) The Cbln2 KO had no effect on the presynaptic LTP typical for burst-spiking neurons that is induced by four 100 Hz/1 s stimulus trains with 10 s intervals under voltage-clamp (D, summary plot of AMPAR-EPSC amplitudes with sample traces on top; E, summary graph of the LTP magnitude (normalized EPSC amplitudes during the last 5 min of recordings at least 30 min after LTP induction); F, summary graph of paired-pulse ratios before and after LTP induction as a measure of the release probability). (G) (H) Same as B & C, but recorded from regular-spiking neurons. Note that the AMPAR-EPSC and NMDAR-EPSC phenotype of the Cbln2 KO is identical in burst- and regular-spiking neurons. (I–K) The Cbln2 KO abolishes NMDAR-dependent postsynaptic LTP that is typical for regular-firing subiculum neurons, and does not involve a change in PPR. Data are from experiments analogous to those described in D-F. All data are means ± SEM. Number of neurons/mice are indicated in bars. Statistical significance was assessed by unpaired two-tailed t-test or two-way ANOVA (*p≤0.05, **p≤0.01, and ***p≤0.001).
-
Figure 1—source data 1
Constitutive Cbln2 deletion increases AMPAR-EPSCs and suppresses NMDAR-EPSCs at CA1→subiculum synapses formed on both burst- and regular-spiking subiculum neurons, and additionally blocks NMDAR-dependent LTP in regular-spiking neurons without affecting cAMP-dependent LTP in burst-spiking neurons.
- https://cdn.elifesciences.org/articles/78649/elife-78649-fig1-data1-v4.xlsx

Constitutive Cbln2 deletion does not alter the coefficient of variation of AMPAR-EPSCs in burst- and regular-spiking subiculum neurons (corresponding to Figure 1).
(A & B) Left, sample traces of evoked AMPAR-EPSCs with 100 μA stimulus intensity (data from Figure 1B and G); right, summary graph of the average coefficient of variation for AMPAR-EPSCs across all 7 conditions of 10-100 μA stimulus intensity. Data are means ± SEM. Number of neurons/mice are indicated in bars. Statistical significance was assessed by unpaired two-tailed t-test revealed no significant differences.
-
Figure 1—figure supplement 1—source data 1
Constitutive Cbln2 deletion does not alter the coefficient of variation of AMPAR-EPSCs in burst- and regular-spiking subiculum neurons.
- https://cdn.elifesciences.org/articles/78649/elife-78649-fig1-figsupp1-data1-v4.xlsx

Conditional Cbln2 KO in the subiculum produces the same phenotype as the constitutive Cbln2 KO at the two different types of CA1→subiculum synapses that are formed on burst- and regular-spiking neurons.
(A) Experimental design for the generation and analysis of littermate control and conditional Cbln2 KO mice. AAVs encoding Cre orΔCre (as a control) were stereotactically injected into the subiculum of conditional Cbln2 KO mice at P21, and mice were analyzed by slice physiology 2–3 weeks later. (B–E) Input/output measurements of evoked AMPAR-EPSCs (B) and NMDAR-EPSCs (D) recorded from burst-spiking neurons in acute subiculum slices reveal that the conditional Cbln2 KO enhances AMPAR-EPSCs (B) without changing the paired-pulse ratio of AMPAR-EPSCs (C) but suppresses NMDAR-EPSCs (D), again without changing the paired-pulse ratio of NMDAR-EPSCs (E), in burst-spiking neurons. Sample traces are shown above the respective summary plots and graphs. (F–I) Same as B-E, but recorded from regular-spiking neurons. Note that the conditional Cbln2 KO phenotype is identical between burst- and regular-spiking neurons. Data are means ± SEM. Number of neurons/mice are indicated in bars. Statistical significance was assessed by unpaired two-tailed t-test or two-way ANOVA (*p≤0.05, **p≤0.01, and ***p≤0.001).
-
Figure 1—figure supplement 2—source data 1
Conditional Cbln2 KO in the subiculum produces the same phenotype as the constitutive Cbln2 KO at the two different types of CA1→subiculum synapses that are formed on burst- and regular-spiking neurons.
- https://cdn.elifesciences.org/articles/78649/elife-78649-fig1-figsupp2-data1-v4.xlsx
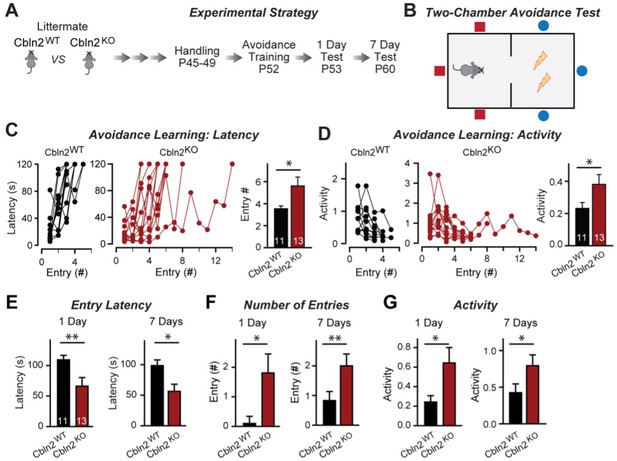
Constitutive Cbln2 deletion impairs contextual memory in the two-chamber avoidance test.
(A, B) Experimental strategy of behavioral experiments utilizing littermate Cbln2 KO and WT mice (A) and design of the two-chamber avoidance test in which mice receive mild electric foot shocks in the otherwise preferred darker chamber (B; Cimadevilla et al., 2001; Qiao et al., 2014). (C, D) Cbln2 KO mice exhibit a delayed learning curve during two-chamber avoidance training. Mice learn to stay in the safe space by remembering visual cues to avoid the foot shock (C, trials for each mouse taking to learn when they remain in safe chamber for more than 2 min) (called latency; summary graphs shows number of entries); D, graphs for the movement activity measured by four independent infrared photobeams in the safe chamber in 2 min and the summary graph shows activity level in the safe chamber for the last training trial. (E–G) Cbln2 KO severely decreases contextual memory in mice as measured by the two chamber avoidance test 1 day (left graphs) or 7 days (right graphs) after training (summary graphs of E, entry latencies; F, number of entries, and G, mouse activity). Data are means ± SEMs, the number of mice analyzed are depicted in the bars. Statistical analyses were performed by one-tail t-test (*=p ≤ 0.05; **=p ≤ 0.01).
-
Figure 2—source data 1
Constitutive Cbln2 deletion impairs contextual memory in the two-chamber avoidance test.
- https://cdn.elifesciences.org/articles/78649/elife-78649-fig2-data1-v4.xlsx
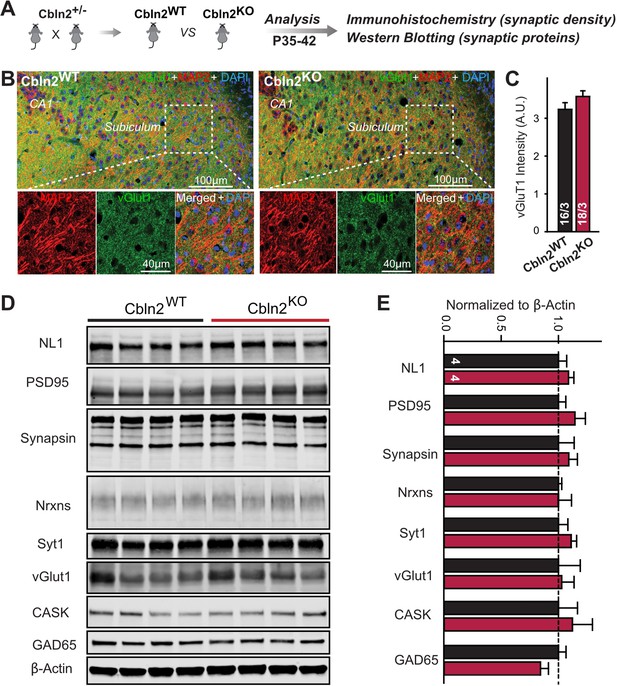
Constitutive Cbln2 deletion does not alter the overall synapse density in the subiculum.
(A) Experimental strategy for the analysis of littermate wild-type and constitutive Cbln2 KO mice. (B) Representative images of subiculum sections stained for vGluT1 as a proxy of synapse density, MAP2 as a proxy of neuronal density, and DAPI. (C) The Cbln2 KO does not cause a major loss of excitatory synapses in the subiculum as indicated by the vGluT1 staining intensity. (D& E) The Cbln2 KO also does not significantly alter the levels of synaptic proteins in the hippocampus. Protein levels were measured in hippocampal lysates by quantitative immunoblotting using fluorescent secondary antibodies (D) representative blots, please also see original full-sized immunoblots in Figure 3—source data 1; (E), summary graph (levels are normalized for β-actin as an internal standard, and then to the controls to render results from multiple experiments comparable; n=3 independent experiments). Data are means ± SEMs, the number of slices/mice or number of mice analyzed are depicted in the bars; statistical analyses by unpaired two-tailed t-test revealed no significant differences.
-
Figure 3—source data 1
Constitutive Cbln2 deletion does not alter the overall synapse density in the subiculum.
- https://cdn.elifesciences.org/articles/78649/elife-78649-fig3-data1-v4.xlsx
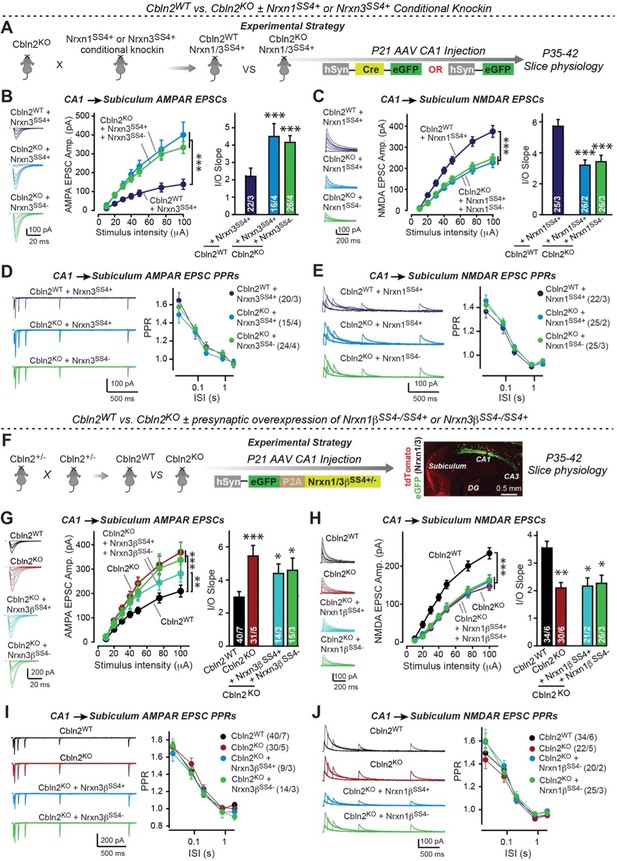
Constitutive Cbln2 deletion occludes regulation of postsynaptic AMPAR- and NMDAR-EPSCs by presynaptic Nrxn1SS4+ and Nrxn3SS4+, respectively.
(A) Experimental strategy for testing whether the Cbln2 deletion blocks the effects of Nrxn1SS4+ and Nrxn3SS4+ signaling. Constitutive Cbln2 KO mice (Cbln2KO) were crossed with Nrxn1SS4+ and Nrxn3SS4+ knockin mice that constitutively express Nrxn1SS4+ and Nrxn3SS4+ splice variants, but that are converted into constitutively expressing Nrxn1SS4- and Nrxn3SS4- splice variants by Cre-recombinase (Dai et al., 2019). Three groups of mice were compared: 1. Cbln2WT mice expressing Nrxn1SS4+ or Nrxn3SS4+, 2. Cbln2KO mice expressing Nrxn1SS4+ and Nrxn3SS4+ in which presynaptic CA1 neurons were infected stereotactically at P21 with AAVs expressing inactive ΔCre (retains presynaptic Nrxn1SS4+ and Nrxn3SS4+ genotype); and 3. Nrxn1SS4+ and Nrxn3SS4+ in which presynaptic CA1 neurons were infected stereotactically at P21 with AAVs expressing active Cre (generates presynaptic Nrxn1SS4- and Nrxn3SS4- genotype). CA1→subiculum synapses were then analyzed in acute slices from these mice at P35-42. (B) On the background of the Cbln2 KO, knockin of Nrxn3SS4+ no longer suppresses AMPAR-ESPCs, nor does it reverse the increase in AMPAR-EPSCs induced by the Cbln2 KO at CA1→subiculum synapses (left, representative traces; middle, summary plot of the input/output relation; right, summary graph of the slope of the input/output relations). (C) Similarly, Nrxn1SS4+ no longer enhances NMDAR-ESPCs on the background of the Cbln2 KO, nor does it reverse the decrease in NMDAR-EPSCs induced by the Cbln2 KO (left, representative traces; middle, summary plot of the input/output relation; right, summary graph of the slope of the input/output relations). (D & E) Constitutive expression of Nrxn1SS4+ and Nrxn3SS4+ alone or in combination with the Cbln2 KO have no effect on the paired-pulse ratio of evoked AMPAR-EPSCs (D) or NMDAR-EPSCs (E) at CA1→subiculum synapses (left, sample traces; right, summary plots of PPRs). (F) Alternative experimental strategy for testing whether the Cbln2 deletion blocks the effects of Nrxn1SS4+ and Nrxn3SS4+ signaling. Analysing the epistatic relation of neurexin alternative splicing at SS4 with the Cbln2 KO at CA1→subiculum synapses using viral overexpression of Nrxn1βSS4+ or Nrxn3βSS4+ in Cbln2 KO mice. The CA1 region of constitutive Cbln2 KO mice was bilaterally infected at P21 by stereotactic injections with AAVs expressing Nrxn1βSS4+, Nrxn1βSS4-, Nrxn3βSS4+, or Nrxn3βSS4-, and subiculum neurons were analyzed 2–3 weeks later. The representative image on the right depicts the signal for eGFP (which is co-expressed with the neurexins) in CA1 neurons after 2 weeks infection. (G) On the background of the Cbln2 KO, overexpression of Nrxn3βSS4+ again no longer suppresses AMPAR-ESPCs, nor does it reverse the increase in AMPAR-EPSCs induced by the Cbln2 KO at CA1→subiculum synapses (left, representative traces; middle, summary plot of the input/output relation; right, summary graph of the slope of the input/output relations). (H) Similarly, overexpressed Nrxn1βSS4+ no longer enhances NMDAR-ESPCs on the background of the Cbln2 KO, nor does it reverse the decrease in NMDAR-EPSCs induced by the Cbln2 KO (left, representative traces; middle, summary plot of the input/output relation; right, summary graph of the slope of the input/output relations). (I & J ) Overexpression of any neurexin has no effect on the paired-pulse ratio of evoked AMPAR-EPSCs (I) or NMDAR-EPSCs (J) (left, sample traces; right, summary plots of PPRs). Data are means ± SEM. Number of neurons/mice are indicated in bars. Statistical significance was assessed by unpaired two-tailed t-test comparing to control and two-way ANOVA (*p≤0.05, **p≤0.01, and ***p≤0.001).
-
Figure 4—source data 1
Constitutive Cbln2 deletion occludes regulation of postsynaptic AMPAR- and NMDAR-EPSCs by presynaptic Nrxn1SS4+ and Nrxn3SS4+, respectively.
- https://cdn.elifesciences.org/articles/78649/elife-78649-fig4-data1-v4.xlsx

Analysis of neurexin SS4 alternative splicing reveals that whereas all neurexins are almost exclusively expressed as SS4 +variants in the cerebellum, a mixture of SS4 +and SS4- variants is observed in other brain regions.
(A) Sample gels of amplified DNA obtained by RT-PCR of mRNAs from the indicated brain regions. RT-PCR was carried out with primers flanking SS4; as a result, in most brain regions two bands are observed that correspond to mRNAs containing (upper bands) and lacking SS4 (lower bands). Please also see original full-sized gels in Figure 4—figure supplement 1—source data 2. (B) Quantification of the prevalence of SS4 +variants of the three neurexins in the indicated brain regions. Data are means ± SEM (n=3).
-
Figure 4—figure supplement 1—source data 1
Analysis of neurexin SS4 alternative splicing reveals that whereas all neurexins are almost exclusively expressed as SS4 +variants in the cerebellum, a mixture of SS4 +and SS4- variants is observed in other brain regions.
- https://cdn.elifesciences.org/articles/78649/elife-78649-fig4-figsupp1-data1-v4.xlsx
-
Figure 4—figure supplement 1—source data 2
Analysis of neurexin SS4 alternative splicing reveals that whereas all neurexins are almost exclusively expressed as SS4 +variants in the cerebellum, a mixture of SS4 +and SS4- variants is observed in other brain regions.
- https://cdn.elifesciences.org/articles/78649/elife-78649-fig4-figsupp1-data2-v4.pdf
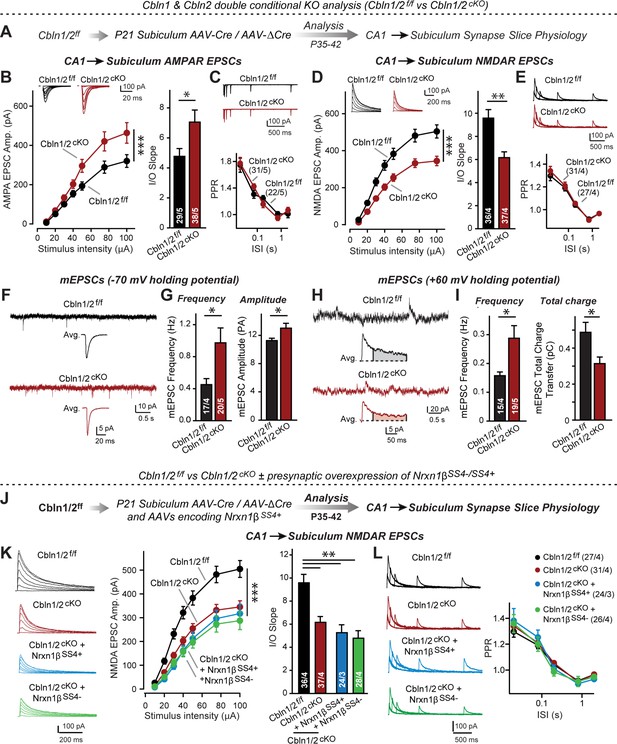
Cbln1 and Cbln2 double KO in the subiculum phenocopies the Cbln2 single KO at CA1→subiculum synapses.
(A) Experimental strategy. AAVs encoding Cre or ΔCre (as a control) were stereotactically injected into the subiculum of conditional KO mice at P21, and mice were analyzed by slice physiology 2–3 weeks later. (B-E) Input/output measurements of evoked EPSCs recorded from combined burst- and regular-spiking neurons in acute subiculum slices reveal that the conditional Cbln2 KO enhances AMPAR-EPSCs (B) without changing the paired-pulse ratio of AMPAR-EPSCs (C) but suppresses NMDAR-EPSCs (D), again without changing the paired-pulse ratio of NMDAR-EPSCs (E). Sample traces are shown above the respective summary plots and graphs. (F-I) Analyses of mEPSCs recorded at –70 mV and +60 mV holding potentials from burst- and regular-firing neurons in the subiculum after deletion of both Cbln1 and Cbln2 reveal an increase in mEPSC frequency measured at both holding potentials, but a decrease in charge transfer only of mEPSCs monitored at a+60 mV holding potential consistent with the decreased NMDAR-EPSC amplitude detected during input/output measurements (F, sample traces; G, bar graphs of the mEPSC frequency and amplitude, respectively; H & I, same as F & G but for recordings at +60 mV). (J) Experimental strategy. The subiculum region of Cbln1/2cKO was bilaterally infected at P21 by stereotactic injections of AAVs expressing ΔCre-eGFP (Cbln1/2f/f) or Cre-eGFP (Cbln1/2cKO), and then two weeks later cohorts of mice injected with Cre were further injected into the CA1 region with AAVs expressing Nrxn1βSS4+ or Nrxn1βSS4-. Mice were then analyzed at P49-P56 by acute slice electrophysiology. (K) Overexpressed Nrxn1βSS4+ no longer enhances NMDAR-ESPCs on the background of the double Cbln1/2 cKO, nor does it reverse the decrease in NMDAR-EPSCs induced by the double Cbln1/2 cKO (left, representative traces; middle, summary plot of the input/output relation; right, summary graph of the slope of the input/output relations). (L) Conditional deletion of both Cbln1 and Cbln2 without or with presynaptic overexpression of Nrxn1βSS4+ or Nrxn1βSS4- does not alter paired-pulse ratios of NMDAR EPSCs. Left panels show sample traces; right panels summary plots of the paired-pulse ratio as a function of the interstimulus interval. Data are means ± SEMs; the number of cells/mice are depicted in the bars. Statistical analyses were performed by two-way ANOVA or unpaired two-tailed t-test comparing KOs to WT (*p≤0.05; **p≤0.01, and ***p≤0.001).
-
Figure 5—source data 1
Cbln1 and Cbln2 double KO in the subiculum phenocopies the Cbln2 single KO at CA1→subiculum synapses.
- https://cdn.elifesciences.org/articles/78649/elife-78649-fig5-data1-v4.xlsx

Analyses of the region-specific expression patterns of Cbln1 and Cbln2 using single-molecule RNA in situ hybridization (A & B).
(A & B) Single-molecule in-situ hybridization analysis of Cbln1 (A) and Cbln2 mRNAs (B) reveals highly restricted expression patterns in brain (left, overview of horizontal mouse brain sections hybridized for Cbln1 or Cbln2 mRNAs; right, representative images for Cbln1 and Cbln2 in the cerebellum (i), hippocampal formation (ii), and mPFC (iii)). Note that Cbln1 is highly expressed only in the cerebellum, whereas Cbln2 is most abundant in the subiculum and PFC (abbreviations used: RSA, retrosplenial agranular cortex; IC, inferior colliculus; PPT, posterior pretectal nucleus; sm, stria medullaris; Au1, primary autitory area; S1 & S2, primary and secondary somatosensory cortex; OB, olfactory bulb; gr, granular layer; mo, molecular layer; pcl, purkinje cell layer; S, subiculum; PrS, presubiculum; Pas, parasubiculum; CA1, 2, 3, cornu ammonis 1, 2, 3; DG, dentate gyrus; CPu, caudate putamen (striatum); cg, cingulum; IL, infralimbic cortex; VO, ventro orbital cortex; MO, medial orbital cortex; PrL, prelimbic cortex). The extremely high expression levels of Cbln1 in the cerebellum causes the Cbln1 signal to become dim in other brain regions if all images are analyzed with the same settings. To compensate for this, the Cbln1 signal strength was enhanced in the A (ii) and A (iii) images, and the strength of the Cbln1 signal in the images cannot be directly compared to that of A (i).

The constitutive Cbln2 deletion does not alter the overall expression levels of Nrxn1, Nrxn2, Nrxn3, Cbln1, Cbln2, GluD1 and GluD2 mRNAs in the PFC or subiculum.
(A & B) Left, sample microdissection of the PFC and subiculum indicated by black dashed boxes from Cbln2 WT and KO brain sections; right, quantification of the relative mRNA levels of the three neurexins, two cerebellins, and two GluDs in the indicated brain regions using qRT-PCR (normalized to β-actin). Data are means ± SEM (n=3).
-
Figure 5—figure supplement 2—source data 1
The constitutive Cbln2 deletion does not alter the overall expression levels of Nrxn1, Nrxn2, Nrxn3, Cbln1, Cbln2, GluD1 and GluD2 mRNAs in the PFC or subiculum.
- https://cdn.elifesciences.org/articles/78649/elife-78649-fig5-figsupp2-data1-v4.xlsx
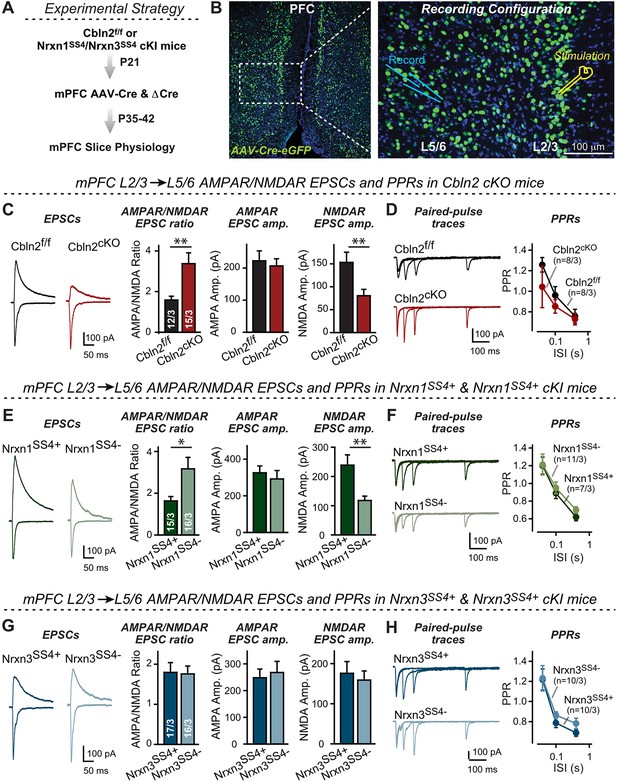
Nrxn1SS4+-Cbln2 signaling controls NMDAR-EPSCs but not AMPAR-EPSCs in the PFC, whereas Nrxn3SS4+-Cbln2 signaling does not regulate either AMPAR- or NMDAR-EPSCs in the PFC.
(A & B) Experimental strategy (left, flow diagram of the experiments); middle and right, Analysis strategies of Cbln2/Nrxn1-SS4/Nrxn3-SS4 conditional KO. Right, the mPFC region of Cbln2cKO was bilaterally infected at P21 by stereotactic injections of AAVs expressing ΔCre-eGFP (Cbln2f/f) or Cre-eGFP (Cbln2cKO), and L5/6 pyramidal neurons in the prelimbic cortex (PL) region were analyzed 2–3 weeks later (A) The stimulation electrode was placed in L2/3 as indicated and applied same stimulation intensity/duration for all groups of mice to keep the consistency (B) C. Left, sample traces of evoked AMPAR- and NMDAR-EPSCs at Cbln2f/f and Cbln2cKO mPFC brain slices; Right, statistics of AMPA/NMDA ratios, AMPAR-EPSCs amplitude, and NMDAR-EPSCs amplitude. (D) Left, sample traces of paired-pulse measurements from each condition; Right, summary plots of PPRs. (E & F) Same as (C & D), but recorded from Nrxn1SS4+ knockin mice in which ΔCre retains a constitutive expression of Nrxn1-SS4+splice variants, whereas Cre converts the Nrxn1-SS4+variants into constitutive Nrxn1-SS4- variants. (G & H) Same as (C & D) but recorded from Nrxn3SS4+ knockin mice in which ΔCre retains a constitutive expression of Nrxn3-SS4+splice variants, whereas Cre converts the Nrxn3-SS4+variants into constitutive Nrxn3-SS4- variants. Data are means ± SEM. Number of neurons/mice are indicated in bars. Statistical significance was assessed by unpaired two-tailed t-test or two-way ANOVA (*P≤0.05, **P≤0.01, and ***p≤0.001).
-
Figure 6—source data 1
Nrxn1SS4+-Cbln2 signaling controls NMDAR-EPSCs but not AMPAR-EPSCs in the PFC, whereas Nrxn3SS4+-Cbln2 signaling does not regulate either AMPAR- or NMDAR-EPSCs in the PFC.
- https://cdn.elifesciences.org/articles/78649/elife-78649-fig6-data1-v4.xlsx
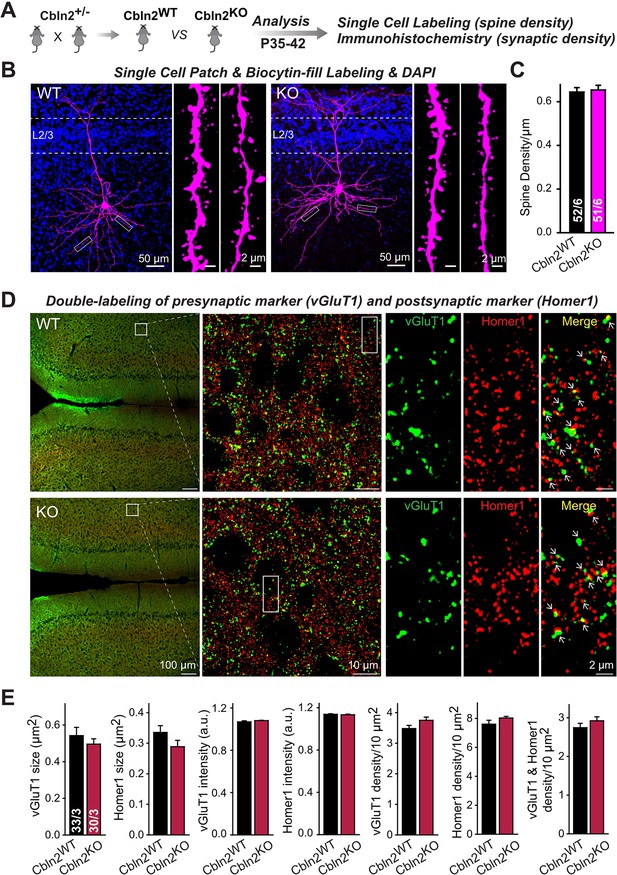
Constitutive Cbln2 deletion does not alter the overall synapse density in the PFC.
(A) Experimental strategy for the analysis of littermate wild-type and constitutive Cbln2 KO mice. (B) Representative images of PFC sections with a single biocytin-filled neuron (left, 20 x images of PFC sections with biocytin labeling and DAPI staining; right, 100 x images of dendrite spines). (C) The Cbln2 KO does not cause a change of dendrite spine density in the PFC as quantified in biocytin-filled neurons. (D) Representative images of PFC sections stained for vGluT1 as a presynaptic marker and Homer1 as a postsynaptic maker (left, 10 x images of PFC sections with vGluT1 and Homer1 staining; middle, 100 x images of the prelimbic cortex (PL), right, enlarged images of vGluT1 staining, Homer1 staining, and their colocalization). (E) The Cbln2 KO also does not significantly alter the size of synaptic puncta, the intensity of synaptic markers, and the density of synapses in the PFC. Data are means ± SEMs, the number of dendrites/cells or sections/mice analyzed are depicted in the bars; statistical analyses by unpaired two-tailed t-test revealed no significant differences.
-
Figure 7—source data 1
Constitutive Cbln2 deletion does not alter the overall synapse density in the PFC.
- https://cdn.elifesciences.org/articles/78649/elife-78649-fig7-data1-v4.xlsx
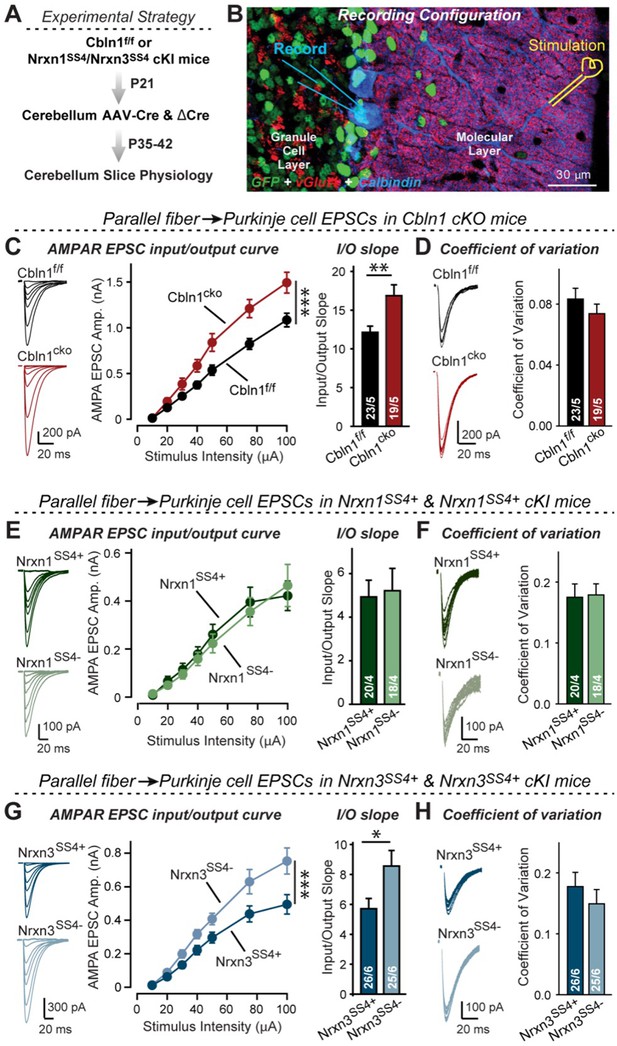
Nrxn3SS4+-Cbln1 signaling controls AMPAR-EPSCs in the cerebellum, but in this brain region Nrxn1SS4+-Cbln1 signaling has no effect.
(A) Experimental workflow for analyzing the effect of the Cbln1 cKO or of the conditional Nrxn1SS4+ or Nrxn3SS4+ knockin on parallel-fiber synaptic transmission in the cerebellum. Note that the expression of ΔCre in Nrxn1SS4+ or Nrxn3SS4+ knockin mice retains the constitutive expression of their SS4 +splice variants, whereas the expression of Cre converts SS4 +into a constitutive SS4- splice variant. (B) Image of a cerebellar cortex section (lobes 4–5) from Cbln1 cKO mouse in which these lobes were infected at P21 by stereotactic injections of AAVs expressing ΔCre-eGFP (Cbln1f/f) or Cre-eGFP (Cbln1cKO). Sections were analyzed at P35 by slice physiology; the positions of the recording electrode in the patched Purkinje cells and of the stimulation electrode in the granule cell layer are indicated. (C) The Cbln1 deletion in cerebellum significantly increases the amplitude of AMPAR-EPSCs at parallel-fiber synapses (left, sample traces of evoked AMPAR-EPSCs; middle, summary plot of AMPAR-EPSCs input-output curves; right, summary graph of the slope of AMPAR-EPSC input/output curves). (D) The Cbln1 deletion in cerebellum has no major effect on the coefficient of variation at parallel-fiber synapses, suggesting that it does not greatly change the release probability (left, sample traces of evoked AMPAR-EPSCs with 50 μA stimulus intensity; right, summary graph of the coefficient of variation of AMPAR-EPSCs). (E & F) Same as (C & D) but recorded from Nrxn1SS4+ knockin mice in which ΔCre retains a constitutive expression of Nrxn1-SS4+splice variants, whereas Cre converts the Nrxn1-SS4+variants into constitutive Nrxn1-SS4- variants. (G & H) Same as (E & F) but for Nrxn3SS4+ knockin mice in which ΔCre retains a constitutive expression of Nrxn1-SS4+splice variants, whereas Cre converts the Nrxn1-SS4+variants into constitutive Nrxn1-SS4- variants. Data are means ± SEM. Number of neurons/mice are indicated in bars. Statistical significance was assessed by two-way ANOVA or unpaired two-tailed t-test (*p≤0.05, **p≤0.01, and ***p≤0.001).
-
Figure 8—source data 1
Nrxn3SS4+-Cbln1 signaling controls AMPAR-EPSCs in the cerebellum, but in this brain region Nrxn1SS4+-Cbln1 signaling has no effect.
- https://cdn.elifesciences.org/articles/78649/elife-78649-fig8-data1-v4.xlsx
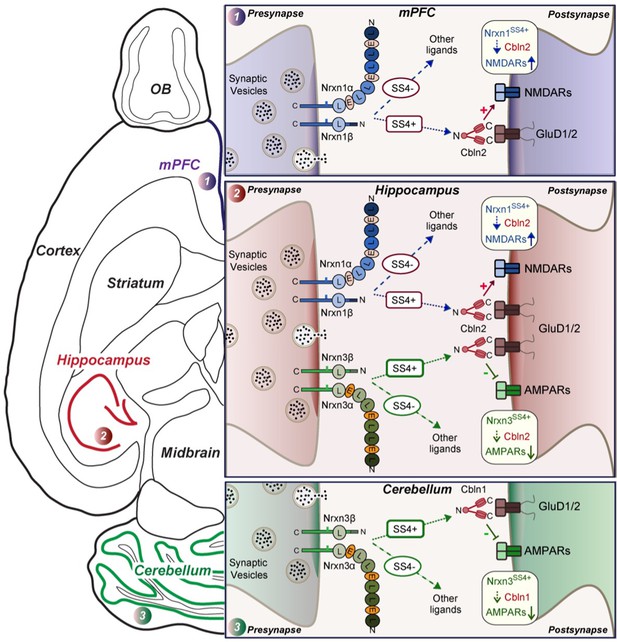
Schematic illustration of how Nrxn1SS4+-Cbln1/2 and Nrxn3SS4+-Cbln1/2 signaling complexes control postsynaptic AMPARs and NMDARs in subicular, prefrontal, and cerebellar circuits.
The schematic is based on data shown previously (Aoto et al., 2013; Dai et al., 2019 and Dai et al., 2021) and described here. Alternative splicing of presynaptic Nrxn1 and Nrxn3 at SS4 that controls their interactions with Cbln1/2 and thereby with postsynaptic GluD1/2 differentially regulates the postsynaptic content of AMPARs and NMDARs in different brain region. In the hippocampus, Nrxn1SS4+-Cbln1/2 complexes enhance NMDAR-EPSCs, whereas Nrxn3SS4+-Cbln1/2 complexes suppress AMPAR-EPSCs, with both types of complexes acting via GluD1/2. In the PFC, Nrxn1SS4+-Cbln1/2 complexes also enhance NMDAR-EPSCs, but Nrxn3SS4+-Cbln1/2 complexes have no effect. In the cerebellum, conversely, Nrxn3SS4+-Cbln1/2 complexes suppress AMPAR-EPSCs, whereas now Nrxn1SS4+-Cbln1/2 complexes have no effect. These results indicate that Nrxn1SS4+-Cbln1/2 and Nrxn3SS4+-Cbln1/2 complexes perform universal functions in regulating AMPARs and NMDARs, respectively, but that these regulatory signaling pathways are differentially expressed in different types of synapses.
Tables
Reagent type (species) or resource | Designation | Source or reference | Identifiers | Additional information |
---|---|---|---|---|
Antibody | Anti-vGluT1 | Millipore | Cat. No. AB5905 | 1:1000 |
Antibody | Anti-Homer1 | Millipore | Cat. No. ABN37 | 1:1000 |
Antibody | Anti-GAD65 | DSHB | Cat. No. mGAD6-a | 1:500 |
Antibody | Anti-MAP2 | Millipore | Cat. No. AB5622 | 1:1000 |
Antibody | Anti-Synaptotagmin 1 | Südhof lab | CL41.1 | 1:1000 |
Antibody | Anti-Neurexin | Südhof lab | G394 | 1:500 |
Antibody | Anti-CASK | BD Transduction Laboratories | Cat. No. 610782 | 1:1000 |
Antibody | Anti-PSD95 | Südhof lab | L667 | 1:500 |
Antibody | Anti-Synapsin | Südhof lab | E028 | 1:1000 |
Antibody | Anti-Neuroligin-1 | Südhof lab | 4F9 | 1:500 |
Antibody | Anti-β-actin | Sigma | Cat. No. A1978 | 1:10000 |
Antibody | Anti-Calbindin | Sigma | Cat. No. C9848 | 1:2000 |
Sequence- based reagent | Cbln1 in-situ probe | Advanced Cell Diagnostics | Cat. No. 538491-C2 | |
Sequence- based reagent | Cbln2 in-situ probe | Advanced Cell Diagnostics | Cat. No. 428551 | |
Recombinant DNA reagent | Lenti-hSyn-Cre-eGFP | Aoto et al., 2013 | N/A | Lentiviral construct to express Cre and eGFP |
Recombinant DNA reagent | Lenti-hSyn-eGFP | Aoto et al., 2013 | N/A | Lentiviral construct to express eGFP |
Recombinant DNA reagent | Lenti-CAG-Cre-eGFP | This paper | N/A | Lentiviral construct to express Cre and eGFP |
Recombinant DNA reagent | Lenti-CAG-eGFP | This paper | N/A | Lentiviral construct to express eGFP |
Recombinant DNA reagent | pAAV-hSyn-Cre-eGFP | Aoto et al., 2015 | N/A | AAV construct to express Cre and eGFP |
Recombinant DNA reagent | pAAV-hSyn-eGFP | Aoto et al., 2015 | N/A | AAV construct to express eGFP |
Recombinant DNA reagent | pAAV-hSyn-eGFP-p2A-Nrxn1βSS4+/- | Dai et al., 2019 | N/A | AAV construct to express eGFP and Nrxn1βSS4+/- |
Recombinant DNA reagent | pAAV-hSyn-eGFP-p2A-Nrxn3βSS4+/- | Dai et al., 2019 | N/A | AAV construct to express eGFP and Nrxn3βSS4+/- |
Chemical compound, drug | CNQX | Tocris | Cat. No. 0190 | |
Chemical compound, drug | Picrotoxin | Tocris | Cat. No. 1128 | |
Chemical compound, drug | TTX | Fisher Scientific | Cat. No. 50-753-2807 | |
Chemical compound, drug | Biocytin | Sigma | Cat. No. B4261 | |
Chemical compound, drug | Streptavidin Alexa 647 | Thermo Fisher | Cat. No. S32354 | 1:1000 |
Genetic reagent (Mus musculus) | Mouse: C57BL/6J wildtype | The Jackson Laboratory | Jax Stock no: 000664 | |
Genetic reagent (Mus musculus) | Mouse: Nrxn1-SS4+, Nrxn3-SS4+cKI | Dai et al., 2019; Aoto et al., 2013 | N/A | |
Genetic reagent (Mus musculus) | Mouse: Cbln1, Cbln2, Cbln12 cKO, Cbln2 KO | Seigneur and Südhof, 2017 | N/A | |
Software, algorithm | Clampfit 10 | Molecular Devices | https://www.moleculardevices.com/products/axon-patch-clamp-system/acquisition-and-analysis-software/pclamp-software-suite | |
Software, algorithm | Igor software | Wavemetrics | https://www.wavemetrics.com/downloads | |
Software, algorithm | Image Studio | LI-COR Biosciences | https://www.licor.com/bio/image-studio/ | |
Software, algorithm | NIS-Elements AR Analysis | Nikon | https://www.microscope.healthcare.nikon.com/products/software/nis-elements/nis-elements-advanced-research | |
Software, algorithm | Viewer III | Bioserve | http://www.biobserve.com/behavioralresearch/products/viewer/ | |
Software, algorithm | Prism | GraphPad Software | https://www.graphpad.com/scientific-software/prism/ | |
Software, algorithm | SigmaPlot | Systat Software | https://systatsoftware.com/sp/download.html |