Ecological lipidology
Figures
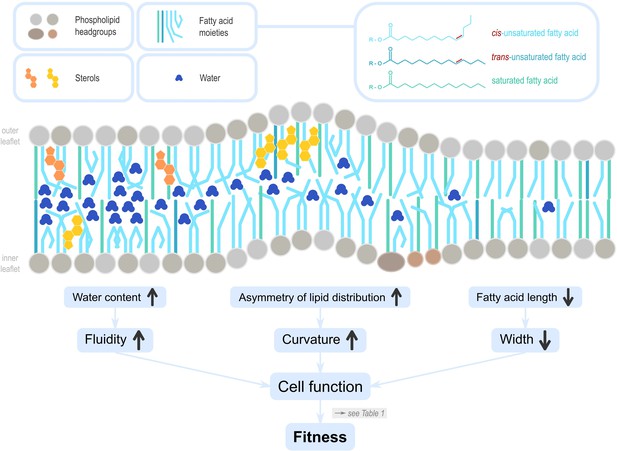
Lipids influence cell membrane properties.
Dietary lipids (DLs) integrate into cell membranes where they have vital cellular functions that ultimately influence reproductive fitness (see Table 1). Phosphoglycerolipids are polar, with a hydrophilic ‘head’ and hydrophobic ‘tail’. In cell membranes, they are oriented with heads on the outside to the extracellular environment, the tails on the inside towards the cell interior. Sterols are also incorporated, and can be locally enriched to affect membrane properties in specific areas. The lipid profile differs between the inner and outer leaflets, reflecting their different biological functions. The lipid profile of a membrane determines its diffusion properties, fluidity, curvature, and width (Harayama and Riezman, 2018). The quantity of membrane sterols, as well as the amount, chain length, and saturation, of fatty acids that form phospholipids and sphingolipids influence the amount of water interspersed in the membrane leaflets. For example, cis unsaturation in the tails of fatty acids change the angle between neighbouring carbons and generate a kink, creating space for water molecules to intersperse, which in turn increases membrane fluidity. Similarly, the unequal distribution of sterols in the leaflets can result in asymmetries (Steck and Lange, 2018), and facilitate membrane curvature. Curvature determines molecular transport and endocytosis but reduces stiffness and integrity, and its maintenance likely incurs energetic costs (Stachowiak et al., 2013). Membrane width is mostly defined by fatty acid chain length, however, their saturation type and abundance is also important. Membrane width influences the insertion of transmembrane proteins and is thus a critical variable for the protein sorting machinery in the cellular organelles, including the Golgi apparatus or the endoplasmatic reticulum.
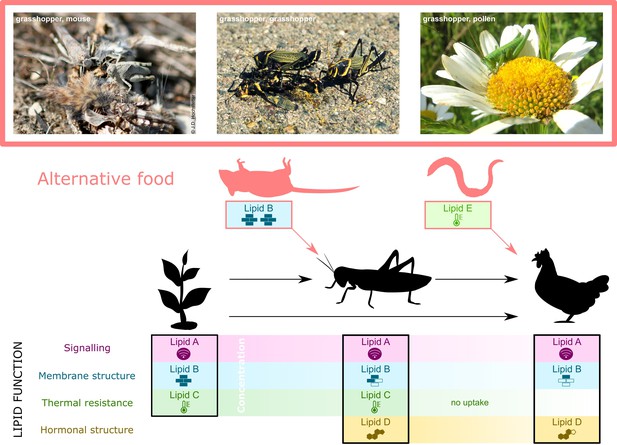
Specific lipid requirements may alter lipid flux through food webs.
Producer dietary lipids (DLs) (e.g., plant lipids A to C, left panel, lower section) are the source that consumers use in tailoring their lipid profiles to meet lipid-mediated body functions (black solid lines and icons) other than mere energy provision. For instance, a herbivorous invertebrate acquires three types of DL from plants (Lipids A to C) and produces one itself (Lipid D, middle panel, lower section). The lipid identity may change if, as in some herbivorous insects, dietary plant sterols are converted to cholesterol (reviewed in Behmer and Nes, 2003; Jing and Behmer, 2020). A consumer that feeds on the herbivore and/or the plant acquires three lipids (A, B, and D) but is either unable to take up lipid C, or lipid C is available in insufficient concentration (right panel, lower section). Quantitative DL availability changes throughout food webs; in this example, the concentration of all lipids (x-axis, lower section) decreases up the food chain. Qualitative DL availability also changes because Lipids C, D, and E are only found in some species. Variation in quantitative and qualitative DL demand is driven by ecological changes – any resulting deficiencies must be countered by alternative sources of DLs (red arrows and icons). In this example, the low concentration of lipid A in the consumer suffices to enable its role in signalling and no additional uptake is needed (Lavrynenko et al., 2015). Lipid D is a hormone precursor in the consumer but requires structural change to function as a hormone. Under changing environment, such as temperatures, Lipid E, substitutes for the lack of Lipid C due to absorption problems, is needed by the consumer to enable thermal resistance (qualitative limitation). If the quantity of Lipid B is insufficient in the herbivore under an ecological change, an alternative source for Lipid B must be found (quantitative limitation). In nature, many example of such changes in food webs exist, such as scavenging on animal corpses or feeding on pollen by otherwise herbivorous insects (see photographs top section). Ecological Lipidology predicts that acquiring the same lipid from different sources (Lipid B from mouse carcass) or different but functionally similar lipids (Lipid E replacing Lipid C in the chicken) will mainly occur when the environment changes.
Tables
Examples of specific dietary lipids affecting animal health and fitness traits.
Potential fitness effects for cellular and metabolic traits as well as the given or putative lipid activity (signalling S, membrane property M, or unknown ? changes) are given in brackets. Rat – Rattus rattus, mouse – Mus musculus, fly – Drosophila melanogaster, worm – Caenorhabditis elegans. * indicates that studies provide evidence for the precise molecular mechanism. See bottom of table for abbreviations of lipids.
DL treatment (vs. control) | EFFECT of TREATMENT | SPECIES | SOURCE |
---|---|---|---|
CELLULAR TRAITS and METABOLISM | |||
DHA C22:6 (vs. cholesterol) | Modulates enterocyte miRNAi 107 expression (alteration of circadian rhythm), (S) | Human Caco2 cell culture | Daimiel-Ruiz et al., 2015 |
5-PAHSA (vs. 9-PAHSA) | Stimulates insulin secretion in the pancreas, facilitates glucose transport, anti-inflammatory (higher metabolic rate), (S) | Mouse | Yore et al., 2014* |
DHA C22:6/EPA C20:5 (vs. other FAs incl. C14:0, C16:0) | Stimulated insulin secretion, facilitates glucose transport, anti-inflammatory (higher metabolic rates), (S) | Mouse | Oh et al., 2013* |
DHA C22:6 (vs. LA C18:2) | Reduces mitochondrial activity (reduced metabolic rate, reduced oxidative stress), (S) | Mammalian cells | Sullivan et al., 2018* |
Lard (vs. fish oil) | Increases ROS production, insulin resistance, mitochondrial dysfunction, oxidative stress, and mitochondrial fission (increased lifespan or reproduction), (S) | Mouse, rat | Chen et al., 2012; Lionetti et al., 2014; Yu et al., 2014 |
PA C16:0, POA C16:1 (vs. OA C18:1, LA C18:2) | Reduces growth of dividing cells (slow development), (S) | Mouse, cell lines | Lien et al., 2021 |
DEFENCE, GROWTH, and DEVELOPMENT | |||
Long-chained SFA (vs. SFA) | Reduces growth hormone production (S) | Mouse | Levi et al., 2015* |
Fish oil (vs. lard) | Reduces enteric damage during infections (M) | Mouse | DeCoffe et al., 2016 |
Sitosterol, stigmasterol, campesterol (vs. cholesterol) | Reduces body size and weight (S) | Fly | Lavrynenko et al., 2015* |
Brassicasterol, cholestanol, zymosterol, desmosterol (vs. sitosterol, stigmasterol, campesterol, cholesterol) | Prevents larval or pupal development because they were no precursors for ecdysteroid hormones (S) | Fly | Lavrynenko et al., 2015 |
POA C16:1 (vs. OA C18:1, PA C16:0; AA C20:4; EPA C20:5; DHA C22:6) | Modulates IGF1 signalling that controls growth and proliferation of white adipose tissue (S) | Mouse | Meln et al., 2019 |
Plant PUFA (vs. yeast PUFA) | Increases developmental rates at 12°C, reduces rates at high temperatures (S, M) | Fly | Brankatschk et al., 2018 |
PHYSIOLOGY, BEHAVIOUR, and HEALTH | |||
MUFA (vs. SFA) n-3 PUFA (vs. n-6 PUFA) | Reduces obesity (S, M) | Human | Moussavi et al., 2008 |
EPA 20:5, DPA 22:5, DHA C22:6 (vs. ALA C18:3) | Increases levels of long-chain n-3 PUFA (C20-22) in the blood thereby delaying mortality (S) | Human | Harris et al., 2021 |
SFAs (vs. MUFAs) | Modulates dopaminergic signalling thereby increasing locomotory activity (S) | Rat | Hryhorczuk et al., 2016 |
AA C20:4 and DGLA C20:3 (vs. EPA C20:5) | Promotes resistance to starvation and extends lifespan by increased autophagy (S) | Worm | O’Rourke et al., 2013 |
Enriched ALA C18:3 (vs. enriched LA C18:2) | Prevents hibernation (?) | Marmot Marmota flaviventris | Hill and Florant, 2000 |
PUFA (vs. SFA) | Individuals select colder areas that reduce body temperature (?) | Several species of lizard | Simandle et al., 2001 |
REPRODUCTION, FERTILITY, and FITNESS | |||
Stigmasterol (vs. cholesterol, campesterol, or sitosterol) | Reduces male fertility (S) | Ladybird beetle Coccinella septempunctata | Ugine et al., 2022b |
Fish oil (vs. corn oil) | Increases fertilising ability of sperm (M) | Chicken Gallus domesticus | Blesbois et al., 1997 |
Plant-based lipids (vs. yeast-based lipids) | Delays sperm production, decreases sperm viability, reduces sperm ROS production rate, no effect on sperm osmotic stress resistance (S, M) | Fly | Guo and Reinhardt, 2020 |
DGLA C20:3 (vs. OA 18:1) | Causes sterility via germ-cell ferroptosis (S) | Worm, human | Perez et al., 2013 |
EPOA C20:5 (vs. ARA C20:4) | No difference in clutch sizes (S) | Daphnia magna and Daphnia pulex | Ilić et al., 2019 |
ALA C18:3 (vs. PA C16:0) | Reduces reproductive rate, offspring size, and survival (S) | Hydra, Hydra oligactis | Kaliszewicz et al., 2018 |
OA C18:1 (vs. LA C18:2, VCA C18:1, DGLA C20:3, EPA C20:5) | Rescues mating-induced reduction in female lifespan (?) | Worm | Choi et al., 2021 |
-
AA – arachidonic acid, ALA – α-Linolenic acid, DGLA – bihomo-γ-linolenic acid, DHA – docosahexaenoic acid, DPA – docosapentaenoic acid, EPA – eicosapentaenoic acid, LA – linoleic acid, OA – oleic acid, PA – palmitic acid, PAHSA – palmitic acid esters of hydroxystearic acid, POA – palmitoleic acid, VCA – cis-vaccenic acid.