The lncRNA Malat1 inhibits miR-15/16 to enhance cytotoxic T cell activation and memory cell formation
Figures
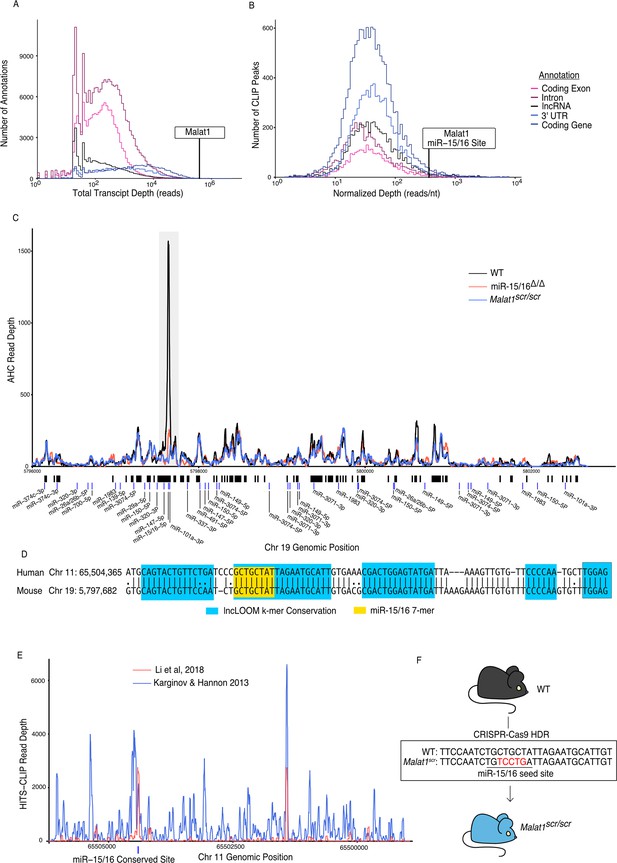
Malat1 is highly bound by miR-15/16.
CD8+ T cells were isolated from spleens, grown in vitro for 5 days, then Ago2 transcriptomic occupancy was assayed via Ago2 HITS-CLIP. (A, B) Transcriptome wide analysis of Ago2 HITS-CLIP libraries prepared from WT cells (combined libraries n = 2). (A) Summed reads across entire annotations. Line indicates total reads across the Malat1 transcript. Malat1 was #8 most highly bound lncRNA annotation, which was in the top 0.0091% of all lncRNA annotations analyzed with >0 HITS-CLIP reads. (B) Ago2 HITS-CLIP peaks were identified and reads were summed within those called peaks that intersected with the given annotation. Peaks were of variable length so summed reads were normalized by peak length. Line indicates HITS-CLIP reads per nucleotide in the called peak containing the miR-15/16-binding site in Malat1. This peak was the #121 most bound HITS-CLIP peak in lncRNA peaks analyzed, which was in the top 2.3% of all evaluated peaks in lncRNAs. (C) Ago2 HITS-CLIP binding to the mouse Malat1 locus reads from combined libraries shown (n = 2 for each genotype). Gray bar indicates the peak containing the miR-15/16-binding site. Black bars indicate regions identified as peaks by piranha. Blue bars indicate predicted binding sites of miRNAs expressed in our dataset from the miRTarget custom sequence prediction algorithm. Gray bar indicates miR-15/16-binding peak. (D) Local alignment of the human and mouse Malat1 sequences near the miR-15/16 conserved binding site. Highlighting indicates the depth of evolutionary conservation of k-mers as predicted by the lncLOOM algorithm (Ross et al., 2021). (E) Ago2 HITS-CLIP binding to the human MALAT1 locus from publicly available datasets (Karginov and Hannon, 2013; Li et al., 2018). Blue vertical bar indicates the conserved miR-15/16-binding site. (F) Schematic representing the creation of the Malat1scr allele. Bases in red indicate the five nucleotides whose sequence was scrambled by CRISPR-Cas9 HDR to prevent miR-15/16 binding.
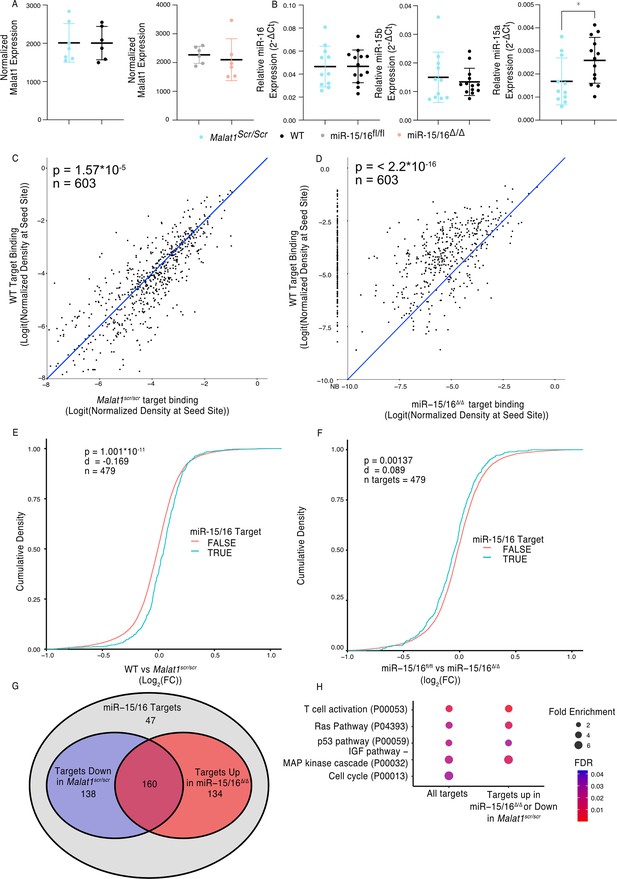
Malat1 Inhibits miR-15/16 binding and suppressive activity.
(A) Malat1 expression measure by RNA-seq from CD8+ T cells isolated from spleens and stimulated with ɑCD3 and ɑCD28 for 24 hr (WT n = 6, Malat1scr/scr n = 7, miR-15/16fl/fl n = 6, miR-15/16Δ/Δ n = 6; 1 experiment, error bars indicate standard deviation). (B) miR-16, miR-15b, and miR-15a expression measured by miRNA qPCR from CD8+ T cells freshly isolated from spleens. Expression was determined relative to 5.8 s ribosomal RNA expression. Unpaired t-test performed to determine significance, and error bars indicate standard deviation. (C, D) TargetScan predicted miR-15/16-binding sites that contained at least one HITS-CLIP read in both WT and Malat1scr/scr CD8+ T cells were compared for depth of Ago2 HITS-CLIP reads. First, reads at the predicted seed site were normalized by total Ago2 HITS-CLIP reads in a given 3′ untranslated region (UTR). To best visualize all sites, logit transforms of these values are plotted. Paired t-test performed to determine significance. Blue line indicates the identity line. Data for each genotype are from combined libraries of n = 2 biological replicates. (C) Comparison of WT and Malat1scr/scr cells. (D) Comparison of WT and miR-15/16Δ/Δ. Values to the left of the y-axis labeled with NB indicate there was no bind detected at that site in the miR-15/16Δ/Δ cells. (E, F) Cumulative density plots to determine changes in expression of miR-15/16 targets. Targets determined by TargetScan predicted miR-15/16 mRNA targets that had at least one 3′ UTR site with reads in both WT and Malat1scr/scr CD8+ T cells. Kolmogorov–Smirnov test used to determine significant differences in the distributions of target and non-target genes. (E) comparison of the log2(FC) between WT and Malat1scr/scr samples stimulated with ɑCD3 and ɑCD28 for 24 hr. (F) comparison of the log2(FC) between miR-15/16fl/fl and miR-15/16Δ/Δ samples stimulated with ɑCD3 and ɑCD28 for 24 hr. (G) Venn diagram of miR-15/16 target expression regulated in concordance with the Malat1-miR-15/16 circuit. The blue circle indicates genes with WT vs Malat1scr/scr log2(FC) >0 and the red circle indicates genes with miR-15/16fl/fl vs miR-15/16Δ/Δ log2(FC) <0. The purple overlap indicates genes that meet both conditions and the gray indicates genes that do not meet either condition. (H) Gene ontology analysis of the bound target set used above as well as genes regulated in accordance with the Malat1-miR-15/16 circuit (WT vs Malat1scr/scr log2(FC) >0 or miR-15/16fl/fl vs miR-15/16Δ/Δ log2(FC) <0). Enrichment determined within the Panther pathway annotations (*p < 0.05).
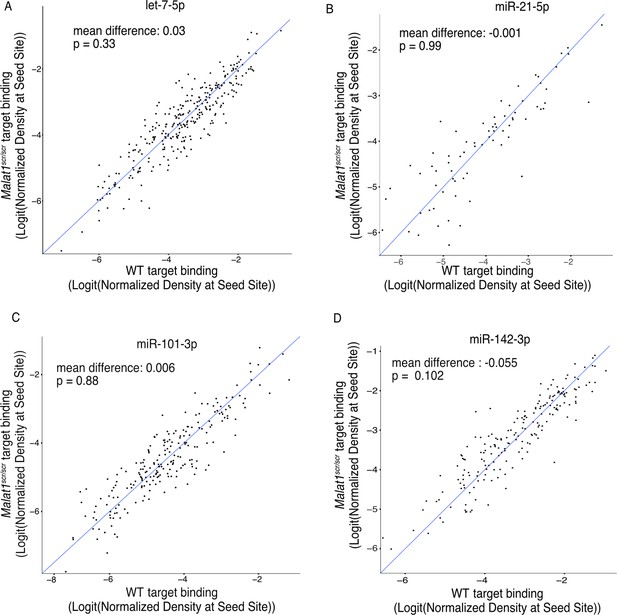
Malat1scr allele does not disrupt other miRNA families.
TargetScan predicted binding sites for highly expressed microRNA families in T cells that contained at least one HITS-CLIP read in both WT and Malat1scr/scr CD8+ T cells were compared for depth of Ago2 HITS-CLIP reads. First, reads at the predicted seed site were normalized by total Ago2 HITS-CLIP reads in a given 3′ untranslated region (UTR). To best visualize all sites, logit transforms of these values are plotted. Paired t-test performed to determine significance. Blue line indicates the identity line. Data for each genotype are from combined libraries of n = 2 biological replicates. (A) Predicted sites for let-7-5p. (B) Predicted sites for miR-21-5p. (C) Predicted sites for miR-101-3p. (D) Predicted sites for miR-142-3p.
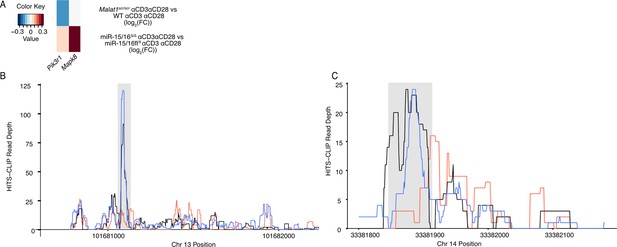
AHC and gene expression analyses nominate direct miR-15/16 targets involved in growth signaling pathways.
(A) Heatmap indicating differential gene expression between WT and Malat1scr/scr cells, and between miR-15/16fl/fl and miR-15/16Δ/Δ cells in RNA sequencing analyses. Histograms of AHC mapping of Ago2 binding in the 3′ untranslated region (UTR) of Pik3r1 (B) and Mapk8 (C) in WT (black), miR-15/16Δ/Δ (red), and Malat1scr/scr (blue) cells. As in Figure 3, AHC libraries were generated from CD8+ T cells isolated from spleens and cultured for 5 days (combined libraries from n = 2 for each genotype). Gray bars indicate the peaks containing TargetScan predicted miR-15/16-binding sites.

The Malat1-miR-15/16 circuit increase CD28 expression and costimulation induced gene expression.
(A) Ago2 HITS-CLIP binding at the Cd28 locus. Sequencing libraries generated from CD8+ T cells isolated from spleens and cultured for 5 days (combined libraries from n = 2 for each genotype). Gray bar indicates the peak containing the TargetScan predicted miR-15/16-binding site. (B) Schematic illustrating the assay scheme to assay acute gene expression downstream of CD28 costimulation. (C) Representative flow cytometry plots of CD28 expression on naive (CD62L+ CD44−) CD8+ T cells from spleens of unchallenged mice. Mean fluorescence intensity for the sample reported in the upper right of the plot. (D) Quantification of CD28 mean fluorescence intensity normalized to the relevant control (Malat1scr/scr compared to WT from three independent experiments; miR-15/16fl/fl compared to miR-15/16Δ/Δ from two independent experiments). (E–H) Cumulative density plots comparing expression of CD28-responsive gene set defined as genes from Martínez-Llordella et al., 2013 with ɑCD3ɑCD28 vs ɑCD3 log2(FC) >1.5 and adjusted p-value <0.001. Kolmogorov–Smirnov test used to determine significant differences in the distributions of target and non-target genes. ɑCD3 used at 1 μg/ml, and ɑCD28 used at 1 μg/ml. (E) Comparison of CD28-responsive genes in WT vs Malat1scr/scr cells stimulated with ɑCD3 alone. (F) Comparison of CD28-responsive genes in WT vs Malat1scr/scr cells stimulated with ɑCD3 and ɑCD28. (G) Comparison of CD28-responsive genes in miR-15/16fl/fl vs miR-15/16Δ/Δ cells stimulated with ɑCD3 alone. (H) Comparison of CD28-responsive genes in miR-15/16fl/fl vs miR-15/16Δ/Δ cells stimulated with ɑCD3 and ɑCD28. (I, J) Heatmaps of CD28-responsive gene set expression by genotype and stimulation condition. Dendrograms represent unbiased hierarchical clustering of the samples. (I) Malat1scr/scr and WT samples compared with ɑCD3 ± ɑCD28. (J) miR-15/16fl/fl vs miR-15/16Δ/Δ samples compared with ɑCD3 ± ɑCD28 ( ***p < 0.001; ****p < 0.0001, error bars indicate standard deviation).
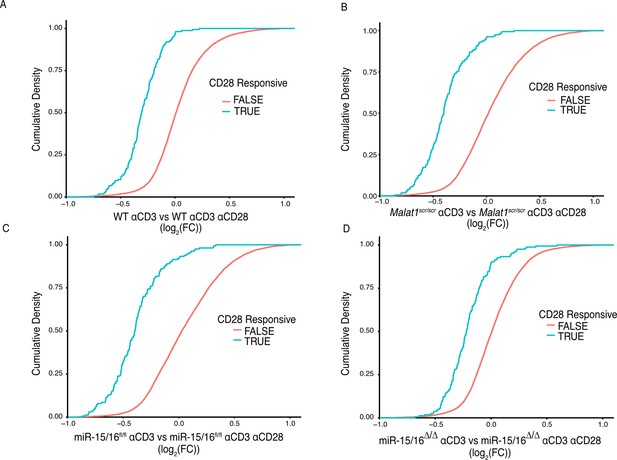
CD28-responsive genes are induced by ɑCD28 stimulation in all genotypes tested.
Cumulative density plots comparing expression of CD28-responsive gene set defined as genes from Martínez-Llordella et al., 2013 with ɑCD3ɑCD28 vs ɑCD3 log2(FC) >1.5 and adjusted p-value <0.001. Kolmogorov–Smirnov test used to determine significant differences in the distributions of target and non-target genes. ɑCD3 used at 1 μg/ml, and ɑCD28 used at 1 μg/ml. Data are from a single experiment with n = 6 for each genotype and stimulation condition combination. (A) Comparison of CD28-responsive genes in WT cells. (B) Comparison of CD28-responsive genes in Malat1scr/scr cells. (C) Comparison of CD28-responsive genes in miR-15/16fl/fl cells. (D) Comparison of CD28-responsive genes in miR-15/16Δ/Δ cells.
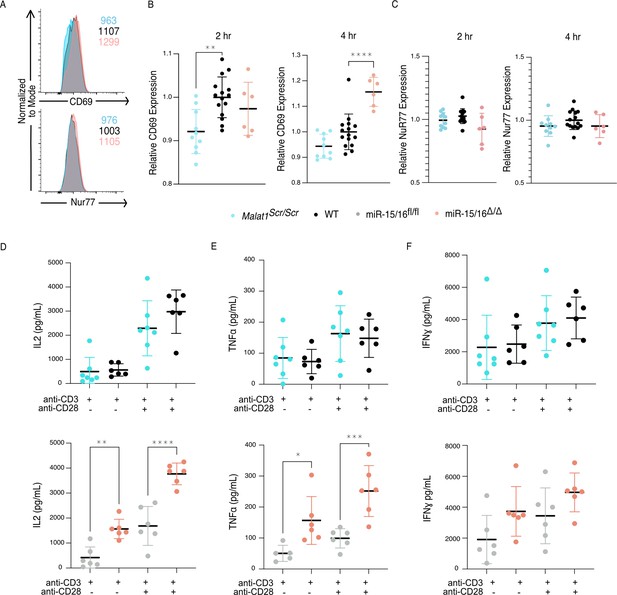
The Malat1-MiR-15/16 circuit increases functional outcomes of CD28 costimulation.
(A–C) CD8+ T cells were isolated from spleens and stimulated with ɑCD3 and ɑCD28 antibodies, results displayed are gated on activated cells (CD69+ Nur77+). (A) Representative histograms of CD69 and Nur77 expression 4 hr after stimulation. (B) Quantification of CD69 mean fluorescence intensity 2 and 4 hr after stimulation. Both time points reflect statistically significant changes (p < 0.01) by ordinary one-way analysis of variance (ANOVA); statistics displayed on graph represent results of post hoc multiple comparisons of Malat1scr/scr to WT and miR-15/16Δ/Δ to WT. Data from two independent experiments, each normalized to WT average value. (C) Quantification of Nur77 mean fluorescence intensity 2 and 4 hr after stimulation. No significant changes determined by ordinary one-way ANOVA. Data from two independent experiments, each normalized to WT average value. (D–F) Quantification of cytokine secretion into the supernatant by CD8+ T cells isolated from spleens, stimulated ɑCD3 ± ɑCD28, and cultured 16 hr. Cell-free supernatant protein concentration measured by ELISA. Data from a single experiment. (D) Quantification of IL-2 secretion. By two-way ANOVA, in both experiments there was a significant (p < 0.0001) increase in IL-2 with the addition of ɑCD28 stimulation. But the only significant (p = 0.0001) genotypic effect was increased IL-2 secretion in miR-15/16Δ/Δ vs mir-15/16fl/fl. Comparisons shown on plot are the results of post hoc multiple comparison tests. (E) Quantification of TNFɑ secretion. By two-way ANOVA, in both experiments there was a significant (p < 0.0001) increase in IL-2 with the addition of ɑCD28 stimulation. But the only significant (p = 0.003) genotypic effect was increased IL-2 secretion in miR-15/16Δ/Δ vs mir-15/16fl/fl. Comparisons shown on plot are the results of post hoc multiple comparison tests. (F) Quantification of IFNγ secretion. By two-way ANOVA, in both experiments there was a significant (p < 0.0001) increase in IL-2 with the addition of ɑCD28 stimulation. But no genotypic effect was observed (*p < 0.05; **p < 0.01; ***p <0 .001; ****p < 0.0001, error bars indicate standard deviation).
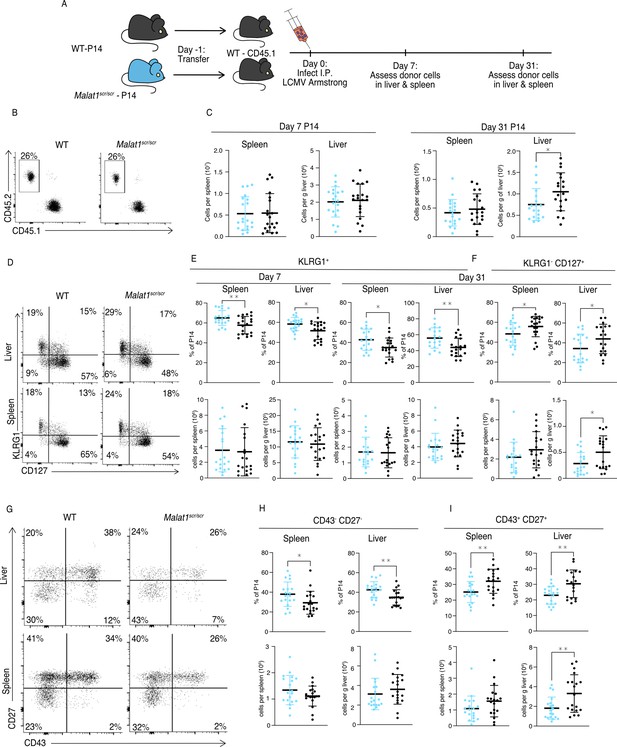
Malat1 enhances memory T cell persistence following LCMV infection.
Malat1scr/scr and WT cells containing the GP33-specific TCR transgene (P14) on the CD45.2 background were transferred separately into congenic CD45.1 WT hosts. One day later the recipient mice were infected with 5 × 105 p.f.u. I.p. LCMV Armstrong. LCMV-specific responses were assayed by monitoring the transferred cells by flow cytometry in the blood, spleen, and liver over time (data from two independent experiments per time point) (A) Schematic of experimental design. (B) Representative flow plots to identify and quantify transferred cells. (C) Quantification of transferred P14 cell numbers at days 7 and 31. (D) Representative flow plots of KLRG1 and CD127 expression on P14 cells at day 31 post infection. (E) Quantification of P14 KLRG1+ cells by percent of P14 and total numbers in spleen and liver at days 7 and 31 post infection. (F) Quantification of P14 KLRG1− CD127+ by percent of P14 and total numbers in spleen and liver at day 31 post infection. (G) Representative flow plots of CD43 and CD27 expression on P14 cells at day 31 post infection. (H) Quantification of P14 CD43− CD27− t-Tem cells by percent of P14 and total numbers in spleen and liver at day 31 post infection. (I) Quantification of P14 CD43+ CD27+ memory cells by percent of P14 and total numbers in spleen and liver at day 31 post infection. Statistics displayed determined by unpaired t-test between Malat1scr/scr and WT transferred cells (*p < 0.05; **p < 0.01, error bars indicate standard deviation).
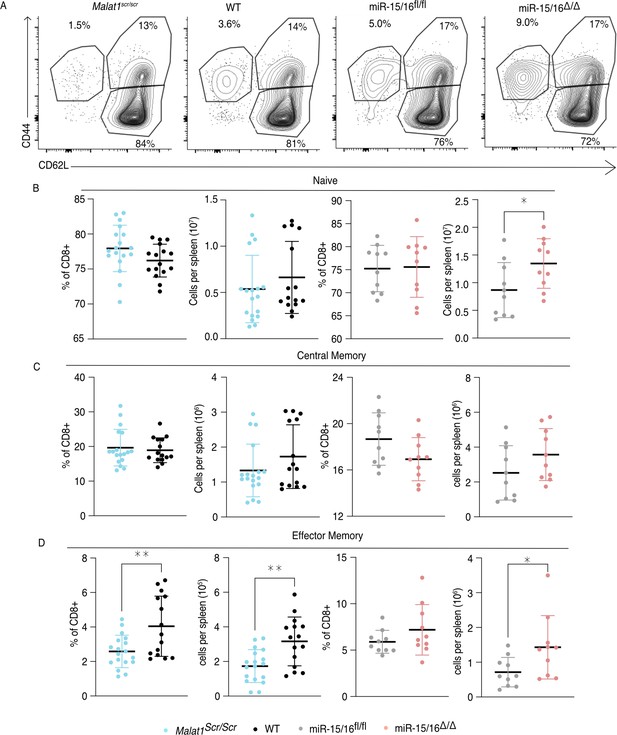
Malat1 regulates memory formation in unchallenged poly-clonal animals.
Cells were isolated from the spleens of young, age-matched, naive mice and analyzed by flow cytometry for CD44 and CD62L to delineate naive, effector memory, and central memory cells. Results shown are gated on CD8+ CD5+ lymphocytes. Data for Malat1scr/scr and WT cells are from three independent experiments. Data for miR-15/16fl/fl and miR-15/16Δ/Δ cells are from two independent experiments. Statistics displayed determined by unpaired t-test between Malat1scr/scr and WT cells or between miR-15/16fl/fl and miR-15/16Δ/Δ cells (*p < 0.05; **p < 0.01 error bars indicate standard deviation). (A) Representative flow cytometry plots of CD44 and CD62L for each genotype assayed. Percentages shown are of CD8+ population. (B) Quantification of naive cells (CD62L+ CD44−). (C) Quantification of central memory cells (CD62L+ CD44+). (D) Quantification of effector memory cells (CD62L− CD44+).
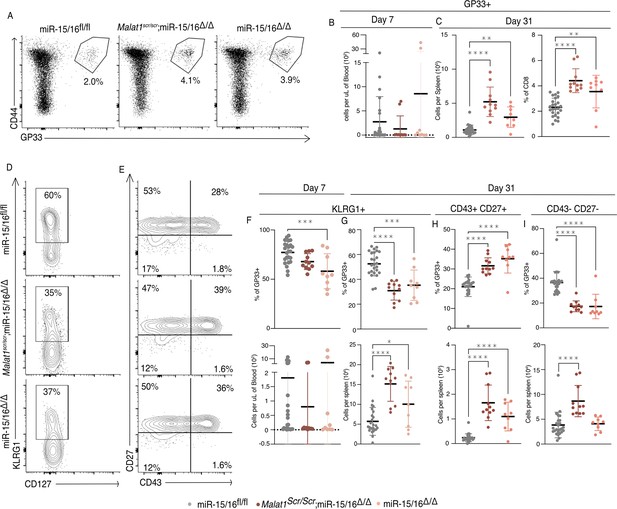
Malat1 is epistatic to miR-15/16 in the regulation memory cell expansion following LCMV infection.
Polyclonal mice of the miR-15/16fl/fl, miR-15/16Δ/Δ, and Malat1scr/scr;miR-15/16Δ/Δ genotypes were directly infected i.p. with 5 × 105 p.f.u. I.p. LCMV Armstrong. Antigen-specific responses were tracked in the blood and spleen using the GP33 tetramer at days 7 and 31 post infection. (A) Representative flow cytometry plots illustrating the gating on antigen-specific cells using CD44 and GP33. (B) Quantification of GP33+ cells in the blood at day 7. (C) Quantification of GP33+ cells in the spleen at day 31 by absolute numbers and percent of the CD8+ T cell population. (D) Representative flow cytometry plots of KLRG1 and CD127 expression at day 31 in the antigen-specific cell population. (E) Representative flow cytometry plots of CD27 and CD43 expression at day 31 in the antigen-specific cell population. (F) Quantification of KLRG1+ antigen-specific cells in the blood at day 7 by relative percentage and absolute numbers. (G) Quantification of KLRG1+ antigen-specific cells in the spleen at day 31 by relative percentage and absolute numbers. (H) Quantification of CD43+ CD27+ memory cells in the spleen at day 31 by relative percentage and absolute numbers. (I) Quantification of CD43− CD27− t-Tem cells in the spleen at day 31 by relative percentage and absolute numbers (*p < 0.05; **p < 0.01; ***p <0 .001; ****p < 0.0001, error bars indicate standard deviation).
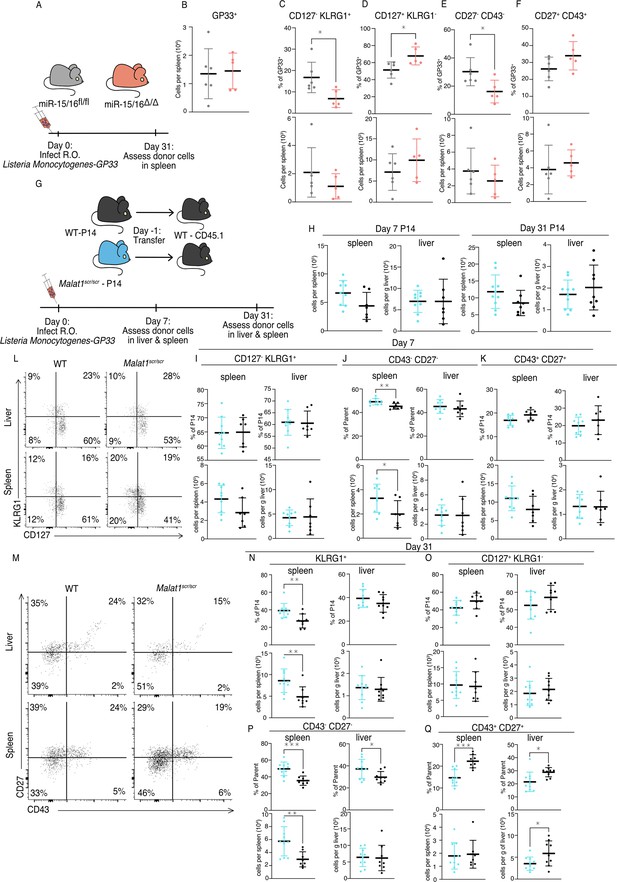
Malat1 and miR-15/16 alter memory T cell differentiation following Listeria monocytogenes infection.
miR-15/16fl/fl and miR-15/16Δ/Δ with a polyclonal TCR repertoire were directly infected with 2 × 104 colony forming units (c.f.u.) r.o. Listeria monocytogenes-gp33 (LM-GP33). LM-GP33-specific responses were then assayed in the spleen 31 days post infection (miR-15/16Δ/Δ n = 5 and miR-15/16fl/fl n = 6 from a single experiment). (A) Schematic of experimental design. (B) Quantification of tetramer-specific CD8 T cells in the spleen. Quantification of tetramer-specific subpopulations by percent of GP33+ and numbers for (C) CD127− KLRG1−, (D) CD127+ KLRG1−, (E) CD27− CD43−, and (F) CD27+ CD43+ populations. Malat1scr/scr and WT cells containing the GP33-specific TCR transgene (P14) on the CD45.2 background were transferred separately into congenic CD45.1 WT hosts. One day later the recipient mice were infected with 2 × 104 c.f.u. r.o. LM-GP33. LM-GP33-specific responses were assayed by monitoring the transferred cells by flow cytometry in the spleen and liver at discrete time points (data from a single experiment per time point). (G) Schematic of experimental design. (H) Quantification of transferred P14 cell numbers at days 7 and 31. Quantification of P14 (I) CD127− KLRG1+, (J) CD43− CD27−, and (K) CD43+ CD27+ cells by percent of P14 and total numbers in spleen and liver at day 7. (L) Representative flow plots of KLRG1 and CD127 expression on P14 cells at day 31 post infection. (M) Representative flow plots of CD43 and CD27 expression on P14 cells at day 31 post infection. Quantification of P14 (N) KLRG1+, (O) CD127+ KLRG1−, (P) CD43− CD27−, and (Q) CD43+ CD27+ cells by percent of P14 and total numbers in spleen and liver at day 31 post infection. Statistics displayed determined by unpaired t-test between Malat1scr/scr and WT transferred cells (*p < 0.05; **p < 0.01; ***p < 0.001, error bars indicate standard deviation).

Malat1 enhances pro-survival cues downstream of T cell activation.
Malat1scr/scr and WT cells containing the GP33-specific TCR transgene (P14) on the CD45.2 background were transferred separately into congenic CD45.1 WT hosts. One day later the recipient mice were infected with 2 × 105 p.f.u. I.p. LCMV Armstrong or 2 × 104 c.f.u. r.o. LM-GP33. Antigen-specific responses were assayed by monitoring the transferred cells by flow cytometry in the spleen. (A–C) Bcl2 expression in transferred P14 cells in the spleen 7 and 31 days post infection for both LCMV and LM-GP33. Data from two independent experiments per LCMV time point and a single experiment per LM-GP33 time point. (A) Representative flow cytometry plots of P14 KLRG1+ CD127− cell Bcl2 expression 7 days post infection. Numbers shown are mean fluorescence intensity. (B) Representative flow cytometry plots of P14 CD43− CD27− cell Bcl2 expression 31 days post infection. Numbers shown are mean fluorescence intensity. (C) Quantification of Bcl2 expression producing cells by mean fluorescence intensity within the indicated P14 subpopulation defined by KLRG1 or CD27 and CD43. (D, E) Analysis of dead cells within splenic P14 CD43 and CD27 subpopulations at days 7 and 31 post LM-GP33 infection, data from a single experiment per time point. (D) Representative flow cytometry plots of P14 subsets defined by CD43 and CD27. Numbers shown represent percent of dead cells per the parent subpopulation. (E) Quantification of dead cells as a percentage of parent P14 subpopulation. (F, G) Analysis of IL-2 producing P14 subsets in the spleen via IL-2 capture assay at days 7 and 31 post infection for both LCMV and LM-GP33, data from a single experiment per infection per time point. (F) Representative flow cytometry plots of all P14 cells stained for KLRG1 and captured IL-2 from both infections at day 7. Numbers represent percent of cells in that quadrant of all P14 transferred cells. (G) Quantification of IL-2 producing cells by percent of parent within the indicated P14 subpopulation defined by KLRG1 or CD27 and CD43. Statistics displayed determined by unpaired t-test between Malat1scr/scr and WT transferred cells, where multiple tests were performed the Holm–Šidák method was used to correct for multiple comparisons (*p < 0.05; **p < 0.01, error bars indicate standard deviation).
Tables
lncRNAs with the most AHC reads.
Non-code gene ID | Total reads | Chromosome | Alias | Note |
---|---|---|---|---|
NONMMUG018330.3 | 4,766,634 | 17 | ENSMUST00000198477.1 | Contains rRNA repeat |
NONMMUG044354.2 | 2,291,785 | M | mt-Rnr2 | Overlaps mt-ND1 |
NONMMUG044353.2 | 1,208,170 | M | mt-Rnr1 | |
NONMMUG076321.1 | 1,012,732 | 6 | Overlaps and best aligns to NONMMUG034479.2 | |
NONMMUG034478.3 | 1,012,015 | 6 | Overlaps and best aligns to NONMMUG034479.2 | |
NONMMUG034479.2 | 1,011,610 | 6 | Contains rRNA repeat | |
NONMMUG007938.3 | 785,970 | 11 | Intronic of Gm36876, contains rRNA repeat (Weirick et al., 2016) | |
NONMMUG020671.2 | 383,447 | 19 | Malat1 | |
NONMMUG015781.2 | 336,664 | 16 | Intronic of Zc3h7a, contains rRNA repeat | |
NONMMUG044321.2 | 317,822 | 9 | Contained in Lars2 3′ UTR, contains rRNA repeat |
lncRNAs with the most AHC reads that do not align to rRNA repeat elements.
Non-code gene ID | Total reads | Chromosome | Alias | Note |
---|---|---|---|---|
NONMMUG018330.3 | 688,875 | 17 | ENSMUST00000198477.1 | Partially anti-sense to XR_877120.2, binding extends beyond rRNA repeat |
NONMMUG020671.2 | 383,447 | 19 | Malat1 | |
NONMMUG094408.1 | 296,723 | 6 | Contains B4A/B3 SINE, binding restricted to SINE | |
NONMMUG005751.2 | 291,393 | 11 | Intronic of Dock2, contains multiple RLTR44-int repeat elements, binding restricted to RLTRs | |
NONMMUG094727.1 | 289,326 | 6 | Contains B4A/B3 SINE, binding is restricted to repeats | |
NONMMUG014644.3 | 241,005 | 15 | Pvt1 | Many repeats, but binding is not restricted to any definite subset |
NONMMUG096664.1 | 229,834 | 15 | Pvt1 | Splice variant of NONMMUG014644.3 |
NONMMUG024327.3 | 222,686 | 2 | Oip5os1, Cyrano | Well described in Han et al., 2020 |
NONMMUG026716.2 | 207,631 | 3 | Overlapping Mbnl1 | |
NONMMUG094659.1 | 196,269 | 6 | Overlapping Foxp1 |
Reagent type (species) or resource | Designation | Source or reference | Identifiers | Additional information |
---|---|---|---|---|
Commercial assay or kit | IL-2 ELISA | Invitrogen | 5018280 | |
Commercial assay or kit | TNFɑ ELISA | Invitrogen | BMS607-3 | |
Commercial assay or kit | IFNγ ELISA | R&D Systems | MIF00 | |
Commercial assay or kit | IL-2 Secretion Assay APC | Miltenyi Biotec | 130-090-987 | |
Sequence-based reagent | Malat1 CRISPR Guide 1 | Dharmacon | RNA oligomer | Sequence – GCATTCTAATAGCAGCAGAT |
Sequence-based reagent | Malat1 HDRT Ultramer | IDT | DNA oligomer | Sequence – ACAGACCACACAGAATGCAGGTGTCTTGACTTCAGGTCATGTCTGTTCTTTGGCAAGTAATATGTGCAGTACTGTTCCAATCTGTCCTGATTAGAATGCATTGTGACGCGACTGGAGTATGATTAAAGAAAGTTGTGTTTCCCCAAGTGTTTGGAGTAGTGGTTGTTGGAGGAAAAGCCATGAGTAACAGGCTGAGTGTT |
Antibody | anti-CD127 PE (Clone-A7R34, rat monoclonal) | Invitrogen | 12-1271-83 | (1:200) |
Antibody | anti-CD127 FITC (Clone-A7R34, rat monoclonal) | Invitrogen | 11-1271-82 | (1:200) |
Antibody | anti-CD45.2 BV785 (Clone-104, mouse monoclonal) | BioLegend | 109839 | (1:200) |
Antibody | anti- CD45.1 Alexa Fluor 700 (Clone-A20, mouse monoclonal) | BioLegend | 110724 | (1:200) |
Antibody | anti-CD90.2 BV605 (Clone-30-H12, rat monoclonal) | BD Biosciences | 740334 | (1:200) |
Antibody | anti-CD90.2 eFluor 450 (Clone-53-2.1, rat monoclonal) | eBiosciences | 48-0902-80 | (1:200) |
Antibody | anti-CD44 PE-Cy7 (Clone-IM7, rat monoclonal) | eBiosciences | 25-0441-82 | (1:200) |
Antibody | anti-CD27 APC (Clone-LG.7F9, Armenian hamster monoclonal) | eBiosciences | 17-0271-82 | (1:200) |
Antibody | anti-CD27 FITC (Clone-LG.7F9, Armenian hamster monoclonal) | eBiosciences | 11-0271-82 | (1:200) |
Antibody | anti-Bim PE (Clone-C34C5, rabbit monoclonal) | Cell Signaling | 12186S | (1:100) |
Antibody | anti-CD43 Percp-Cy5.5 (Clone-1B11, rat monoclonal) | BioLegend | 121224 | (1:200) |
Antibody | anti-CD45.2 PE-Cy7 (Clone-104, Mouse monoclonal) | BD Biosciences | 560696 | (1:200) |
Antibody | anti-CD45.1 BV785 (Clone-A20, mouse monoclonal) | BioLegend | 110743 | (1:200) |
Antibody | anti-Bcl2 Alexa Fluor 647 (Clone-BCL/10C4) | BioLegend | 633510 | (1:100) |
Antibody | anti-Bim Alexa Fluor 700 (Clone-C34C5, rabbit monoclonal) | Cell Signaling | 28997S | (1:100) |
Other | GP33 Tetramer PE | NIH Tetramer Core | 57624 | Peptide: KAVYNFATM (1:100) |
Antibody | anti-KLRG1 BV711 (Clone-2F1, hamster monoclonal) | BD Bioscience | 564014 | (1:200) |
Other | Fixable Viability Dye eFluor 780 | eBioscience | 65-0865-14 | (1:2000) |
Antibody | anti-CD8ɑ BV805 (Clone-53-6.7, rat monoclonal) | BD Bioscience | 612898 | (1:200) |
Antibody | anti-CD28 FITC (Clone-E18, mouse monoclonal) | BioLegend | 122008 | (1:200) |
Antibody | anti-CD69 APC (Clone-H1.2F3, Armenian hamster monoclonal) | Invitrogen | 17-0691-82 | (1:200) |
Antibody | anti-Nur77 PE (Clone-12.14, mouse monoclonal) | Invitrogen | 12-5965-80 | (1:100) |
Antibody | anti-CD62L BV605 (Clone-MEL-14, rat monoclonal) | BD Biosciences | 563252 | (1:200) |