Current perspectives on the multiple roles of osteoclasts: Mechanisms of osteoclast–osteoblast communication and potential clinical implications
Figures
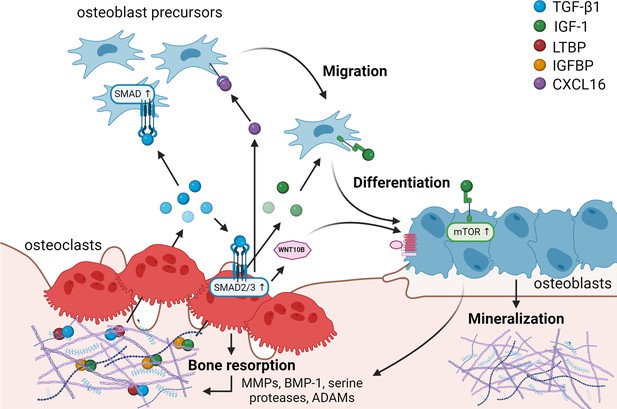
Matrix-derived coupling factors.
To carry out their bone resorptive activity, mature osteoclasts secrete serine proteases, MMPs, ADAMs, and BMPs that cleave latency-associated proteins and liberate coupling factors from the extracellular matrix (ECM). TGF-β1 and IGF-1 are the two major factors that are released from the ECM following osteoclast bone resorption. TGF-β1, released after cleavage of LTBP by osteoclast-secreted proteases, acts on osteoblast precursors by activating SMAD signaling to promote cell migration, and on osteoclasts to stimulate the production of WNT10B and CXCL16. WNT10B stimulates osteoblast differentiation and mineralization, while CXCL16 collaborates with TGF-β to enhance osteoblast precursor migration to the resorptive sites. IGF-1 is activated after cleavage of its regulatory protein IGFBP by proteases secreted by osteoblasts upon bone resorption. Active IGF-1 induces differentiation of osteoblast precursors recruited by TGF-β1 by activating the mammalian target of rapamycin(mTOR) signaling pathway. BMP-1: bone morphogenetic protein 1; CXCL16: C-X-C motif chemokine ligand 16; IGF-1: insulin-like growth factor 1; IGFBP: insulin-like growth factor-binding protein; LTBP: latent TGF-β-binding protein; MMPs: metalloproteinases; TGF-β1: transforming growth factor-β1.
© 2024, BioRender Inc. Figure 1 was created using BioRender, and is published under a CC BY-NC-ND license. Further reproductions must adhere to the terms of this license.
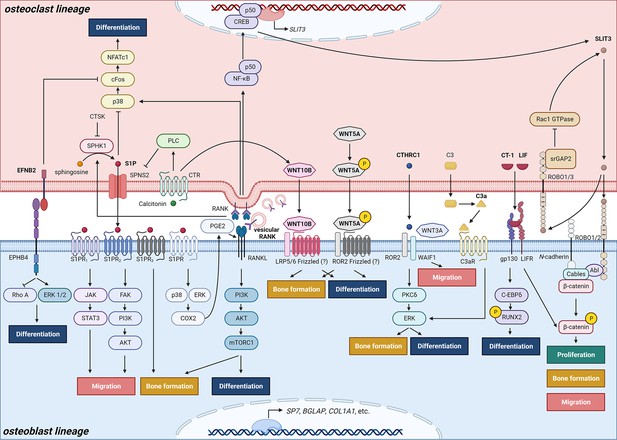
Schematic representation of clastokines produced by osteoclasts and osteoclast precursors and interaction with their receptors on cells from the osteoblast lineage.
The picture shows the pathways that were demonstrated to carry out clastokine anabolic function in vivo and their known interactions. However, many mechanisms and molecular players remain poorly understood.
© 2024, BioRender Inc. Figure 2 was created using BioRender, and is published under a CC BY-NC-ND license. Further reproductions must adhere to the terms of this license.
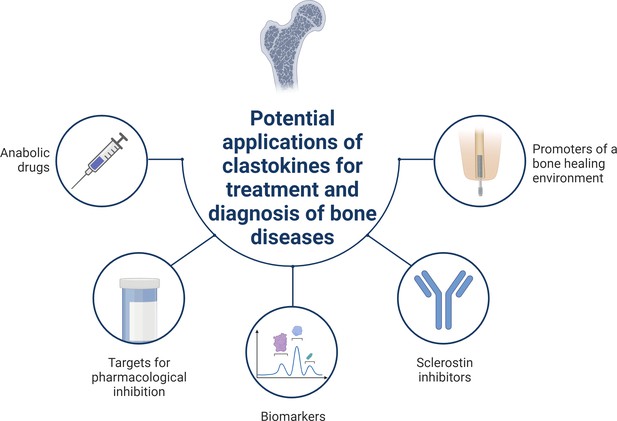
Schematic summary of potential applications of clastokines for treatment and diagnosis of bone diseases based on research evidence.
Clastokines may be used as anabolic drugs able to promote bone formation without negatively affecting bone resorption. RANKL represents a target for pharmacological inhibition to activate reverse signaling and promote bone formation while simultaneously suppressing bone resorption. Circulating levels of clastokines correlate with bone quality in human, implying the possibility of using them as predictive biomarkers. Clastokines such as cardiotrophin-1 (CT-1) and leukemia inhibitory factor (LIF) have been shown to inhibit sclerostin production by osteocytes and have the potential to be used as sclerostin inhibitors with limited side effects. Titanium osteoimplants have been demonstrated to modulate osteoclast secretory phenotype, increasing clastokine production and promoting a bone healing environment.
© 2024, BioRender Inc. Figure 3 was created using BioRender, and is published under a CC BY-NC-ND license. Further reproductions must adhere to the terms of this license.
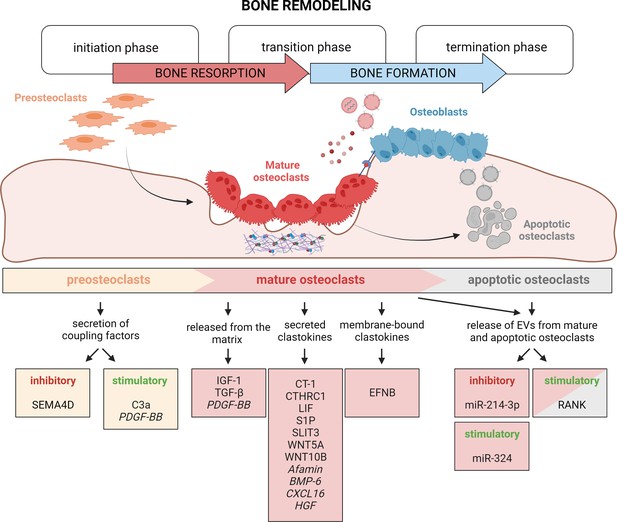
Timely activation of clastokines during bone remodeling.
Bone remodeling is generally classified into three phases: initiation, transition, and termination. During the initiation phase, preosteoclasts are recruited to the bone remodeling site. These cells have been demonstrated to secrete C3a and PDGF-BB clastokines, but also soluble SEMA4D, which has an inhibitory effect on osteoblast differentiation. In the transition phase, mature, bone resorptive osteoclasts promote switching toward bone formation in three ways: by releasing coupling factors from the matrix, by directly secreting them, and by engaging in direct cell–cell contact with cells of the osteoblast lineage. They are also able to release extracellular vesicles (EVs) containing stimulatory and inhibitory miRNAs or vesicular RANK, activating RANKL reverse signaling. Apoptotic osteoclasts have been shown to also release vesicular RANK, which could further stimulate osteoblast differentiation during the termination phase. Putative clastokines are listed in italic.
© 2024, BioRender Inc. Figure 4 was created using BioRender, and is published under a CC BY-NC-ND license. Further reproductions must adhere to the terms of this license.
Tables
Summary of coupling factors linking bone resorption to bone anabolism.
Known coupling factor | Osteoblast receptor | Reported coupling and anabolic effects | Mechanisms | References | |
---|---|---|---|---|---|
Membrane bound | |||||
EFNB2 | Ephrin B2 | EPHB4 | Promotes osteoblast differentiation in vitro and in vivo. Prevents osteoblast apoptosis in vitro and in vivo. | Ephrin B2 binds to EPHB4 receptor and inhibits RhoA activity. | Zhao et al., 2006; Tonna et al., 2014 |
Matrix derived | |||||
TGF-β1 | Transforming growth factor-β1 | TGFβR1 | Promotes MSCs migration to the remodeling sites in vitro and in vivo. | SMAD signaling mediates the creation of a TGF-β1 gradient that directs cell migration. | Tang et al., 2009; Ota et al., 2013b; Ota et al., 2013a |
Stimulates the secretions of other clastokines such as WNT10B, LIF, and CXCL16. | TGF-β activates SMAD2/3 signaling to stimulate CXCL16 expression and SMAD2/3 and MEK signaling to stimulate LIF expression. | ||||
IGF-1 | Insulin-like growth factor 1 | IGF1R | Promotes osteoblast differentiation. | IGF-1 activates mTOR1 signaling pathway. | Xian et al., 2012 |
Secreted (clastokines) | |||||
S1P | Sphingosine-1-phosphate | S1Ps receptors | Promotes osteoblast differentiation in vitro. Promotes bone formation in vivo. | S1P is phosphorylated by SPHK1, an event inhibited in presence of cathepsin K. S1P is secreted from the osteoclasts thanks to SPNS2 export protein. In the extracellular space, S1P binds its S1PR3 receptor located on osteoblast surface. In presence of calcitonin, binding its receptor (CTR) on osteoclast membrane, PLC signaling results in the inhibition of SPNS2 expression. | Ryu et al., 2006; Quint et al., 2013; Pederson et al., 2008; Keller et al., 2014; Lotinun et al., 2013 |
Enhances osteoblast migration and survival in vitro. | MSCs chemokinesis is stimulated by JAK/STAT3 and FAK/PI3K/AKT signaling pathways via S1PR1 and S1PR2, respectively. | ||||
Increases RANKL expression in vitro. | S1P binding to its receptor leads to the activation of ERK and p38 to upregulate COX2 level, leading to increased PGE2 production in the osteoblast. | ||||
Suppresses osteoclast differentiation in vitro. | Following RANKL-binding RANK on osteoclast precursors, the downstream activation of p38, c-Fos, and NFATc1 stimulates osteoclast differentiation. RANKL also stimulates S1P production by SPHK1. Intracellular S1P constitutes a negative feedback loop by suppressing p38 and inhibiting osteoclast differentiation. | ||||
WNT10B | Wingless-type MMTV integration site family, member 10B | N/A | Promotes osteoblast differentiation in vitro. Improves bone mineralization, bone quality and quantity in vivo. | WNT10B expression and secretion is stimulated by calcitonin and by TGF-β1. | Ota et al., 2013a; Pederson et al., 2008; Zheng et al., 2019; Hsiao et al., 2020 |
C3a | Complement component 3a | C3aR | Promotes osteoblast differentiation in vitro. Stimulates bone formation and maintains bone mass and structure. | C3a binds to its osteoblast receptor C3aR, and then likely induces ERK pathway, but the mechanism is still poorly understood. | Matsuoka et al., 2014 |
CTHRC1 | Collagen Triple Helix Repeat Containing 1 | WAIF1, ROR2 | Promotes osteoblast differentiation and mineralization in vitro. Suppresses adipocytic differentiation in vitro. Maintains bone mass and trabecular structure through regulation of bone formation in vivo. | WAIF1 and ROR2 receptors, located on the surface of stromal cells, bind CTHRC1 and activate the PKCδ/ERK pathway, stimulating differentiation into osteoblasts. | Kimura et al., 2008; Takeshita et al., 2013; Matsuoka et al., 2018 |
Stimulates chemotaxis of stromal cells. | The stimulation occurs in presence of WNT3A, supporting a crosstalk between CTHRC1 and WNT pathway. | ||||
WNT5A | Wingless-type MMTV integration site family, member 5A | N/A | Maintains bone mass through regulation of bone formation in vivo. | WNT5A derived from osteoclasts is phosphorylated at Serine residues to acquire its function. | Roberts et al., 2020 |
SLIT3 | Slit Guidance Ligand 3 | ROBO1/2 | Stimulates osteoblast migration and proliferation in vitro and in vivo. Promotes bone formation in vivo. | During osteoclast differentiation, RANKL induces the activation of NF-κB p50 and CREB, increasing SLIT3 expression. SLIT3 signaling is mediated by ROBO1 and ROBO2 receptors in osteoblasts. SLIT3/ROBO complex associates with Abl kinase, N-cadherin, and β-catenin. Following Abl phosphorylation, N-cadherin-associated β-catenin is released, promoting osteoblast migration and proliferation. | Kim et al., 2018; Shin et al., 2020 |
Suppresses bone resorption in an autocrine manner in vivo. | SLIT3 signaling is mediated by ROBO1 and ROBO3 receptors in osteoclasts. Osteoclast SrGAP2 intracellularly binds the SLIT3/ROBO complex and inhibits Rac1 GTPase, resulting in the inhibition of TNF-α-mediated osteoclastogenesis. The pathway has negative feedback: Rac1 promotes the production of SLIT3, which recruits SrGAP2 to inhibit Rac1 expression. | ||||
CT-1 | Cardiotrophin-1 | LIFR, gp130 | Increases osteoblast activity in vitro. Suppresses adipocytic differentiation in vitro. Promotes bone formation in vivo. Inhibits sclerostin production. | CT-1 induces the expression of C/EBPδ, which acts in synergy with RUNX2 to promote osteocalcin expression through a C/EBP enhancer element. CT-1 induces high level of STAT3 and ERK phosphorylation in vitro. CT-1 signals through LIFR and gp130 receptors on osteoblast membrane. A third, CT-1-specific receptor subunit that has still not yet been identified might also be involved. | Sims and Walsh, 2010; Walker et al., 2008; Walker et al., 2010 |
LIF | Leukemia inhibitory factor | LIFR, gp130 | Promotes bone formation in vivo. | LIF expression and secretion are stimulated by TGF-β1 through SMAD2/3 signaling. LIF binds LIFR and gp130 receptors on osteoblast surface. LIF signaling downregulates sclerostin expression and upregulates WNT/β-catenin pathway in osteoblasts. | Ota et al., 2013b; Sims and Walsh, 2010; Walker et al., 2010 |
Putative clastokines (limited data) | |||||
BMP6 | Bone morphogenetic protein 6 | N/A | Promotes MSCs differentiation, mineralization, and migration in vitro. | N/A | Pederson et al., 2008 |
Afamin | Afamin | N/A | Induces osteoblast precursors migration in vitro and in vivo. | Afamin activates the AKT pathway in osteoblasts. | Kim et al., 2012 |
PDGF-BB | Platelet-derived growth factor BB | PDGFR-β | Induces a migratory response in MSCs in vitro. Inhibits osteoblast differentiation in vitro. Promotes bone formation in vivo*. | N/A | Kreja et al., 2010; Sanchez-Fernandez et al., 2008; Kubota et al., 2002; Nash et al., 1994; Mitlak et al., 1996 |
HGF | Hepatocyte growth factor | cMET | Promotes osteoblast proliferation in vitro. Stimulates osteoclast migration in vitro. | HGF binding to cMET activates the PI3K, Akt, and c-Src cascade, which promotes the binding of c-Jun to the AP-1 site. This results in the stimulation of OPN expression in osteoblasts. | Grano et al., 1996; Chen et al., 2012 |
CXCL16 | Chemokine (C-X-C motif) Ligand 16 | N/A | Stimulates osteoblast migration in vitro. | CLXC16 expression and secretion are stimulated by TGF-β1 through SMAD2/3 signaling. | Ota et al., 2013b |
EV-contained | |||||
Vesicular RANK | Receptor activator of nuclear factor κB | RANKL | Promotes osteoblast differentiation and bone formation in vitro and in vivo. | The binding activates RANKL reverse signaling, triggering, the PI3K–Akt–mTORC1 pathway with subsequent production of RUNX2 by osteoblasts. | Ikebuchi et al., 2018 |
miR-214-3p | EPHA2 | Suppresses osteoblast differentiation and bone formation in vitro and in vivo. | miR-214-3p inhibits ATF4 and Osterix expression. | Li et al., 2016; Wang et al., 2013; Shi et al., 2013 | |
miR-324 | N/A | Enhances osteoblast differentiation and mineralization in vitro and in vivo. | miR-324 downregulates the expression of ARHGAP1, a member of the RhoGAP family containing GTPase-activating proteins, stimulating RhoA/ROCK signaling. | Liang et al., 2021 |
-
MSCs: mesenchymal stem cells; N/A: unclear or missing evidence. *Still controversial.