Functional identification of soluble uric acid as an endogenous inhibitor of CD38
Figures
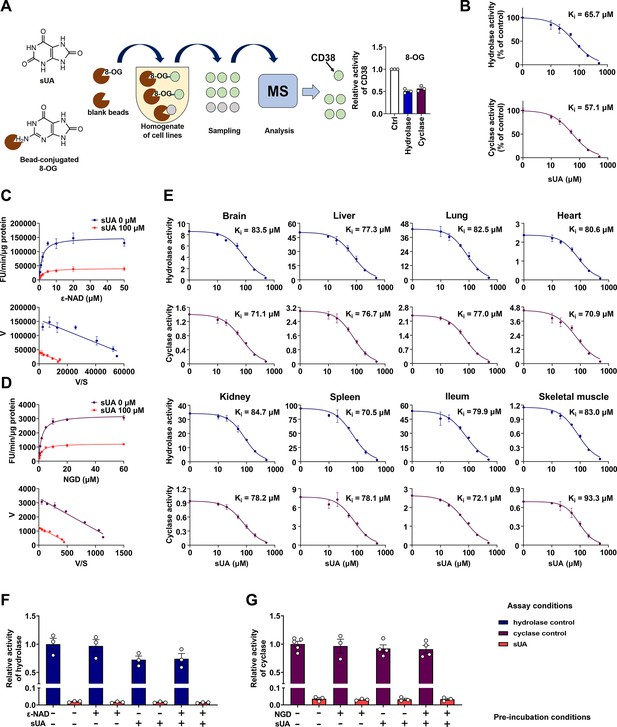
Identification of soluble uric acid (sUA) as an endogenous inhibitor for CD38.
(A) Preliminary screening of 8-oxoguanine (8-OG) binding proteins by mass spectrum (MS)-based proteomics, and effect of 8-OG (50 μM) on CD38 activity (n=3 experiments/technical replicates). (B) Hydrolase and cyclase activities of recombinant human CD38 (hCD38) in the presence of sUA (0–500 μM), using nicotinamide 1, N6-ethenoadenine dinucleotide (ε-NAD+), and nicotinamide guanine dinucleotide (NGD) as substrates, respectively (n=3 experiments/technical replicates). (C and D) Effect of different substrate concentrations on sUA inhibition of recombinant hCD38 hydrolase (C) and cyclase (D) activities (n=3 experiments/technical replicates). (E) Effect of different sUA concentrations (0–500 μM) on hydrolase and cyclase activities (FU/min/μg protein) in tissues from 8- to 12-week-old wild-type (WT) mice (n=3 experiments from 3 mice). (F and G) Reversibility of inhibition of recombinant hCD38 hydrolase (F) and cyclase (G) activities by sUA. After 30 min pre-incubation as indicated, samples were diluted 100-fold in reaction buffer with or without 500 μM sUA for enzyme assay (n=3–5 experiments/technical replicates). Data are mean ± s.d. (B–E) or mean ± s.e.m. (A, F, and G).

Soluble uric acid (sUA) inhibition of CD38 and reversibility in THP-1 and A549 cells.
(A) Hydrolase activity of THP-1 cells in the presence of sUA (0–500 μM) (n=3 experiments/technical replicates). (B) Effect of different ε-NAD+ concentrations on sUA inhibition of hydrolase activity of THP-1 cells (n=3 experiments/technical replicates). (C and D) Reversibility of inhibition of hydrolase (A549 cells) (C) and cyclase (THP-1 cells) (D) by sUA (n=3 experiments/technical replicates). Data are mean ± s.d. (A and B) or mean ± s.e.m. (C and D).

Endogenous soluble uric acid (sUA) concentrations in the final reaction buffer for enzyme assays.
sUA levels in initial homogenate or membrane fractions were measured, then the endogenous sUA concentrations in the final reaction buffer were calculated based on loading dilution (n=3 biologically independent samples). Data are mean ± s.e.m.
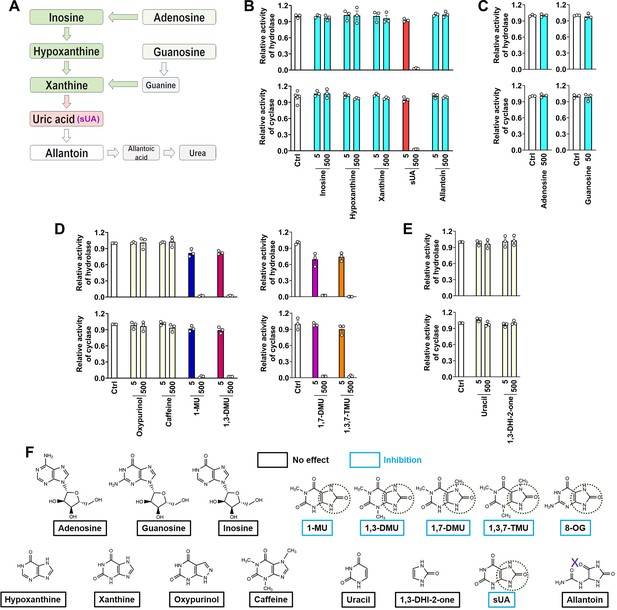
CD38 inhibition is restricted to soluble uric acid (sUA) in purine metabolism.
(A) Major pathways of purine metabolism. (B and C) Effect of sUA and its precursors and metabolite on hydrolase and cyclase activities of recombinant hCD38 (n=3 experiments/technical replicates for each ligand). (D) Effect of different analogs on hydrolase and cyclase activities (n=3 experiments/technical replicates). THP-1 cells were used to detect the effects of oxypurinol, caffeine, 1-methyluric acid (1-MU), and 1,3-dimethyluric acid (1,3-DMU) on hydrolase activity, recombinant hCD38 was used in the remaining detections. (E) Effect of uracil and 1,3-dihydroimidazol-2-one (1,3-DHI-2-one) on hydrolase and cyclase activities of recombinant hCD38 (n=3 experiments/technical replicates). (F) A structural comparison reveals the functional group for CD38 inhibition. The concentrations of all ligands are from 5 to 500 μM. Data are mean ± s.e.m.

Effect of soluble uric acid (sUA), sUA precursors, and metabolite, and other derivates on CD38 activity in cells and tissues.
(A–C) Effect of sUA and its precursors and metabolite on hydrolase (A and B) and cyclase (C) activities (n=3 experiments/technical replicates). (D and E) Effect of uracil and 1,3-dihydroimidazol-2-one (1,3-DHI-2-one) on hydrolase and cyclase activities of wild-type (WT) lung tissues (n=3 experiments/technical replicates). Data are mean ± s.e.m.
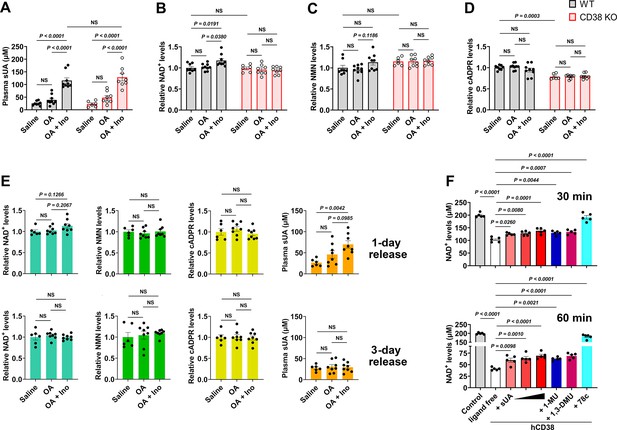
Soluble uric acid (sUA) physiologically limits NAD+ degradation via CD38 inhibition.
Wild-type (WT) and CD38 knockout (KO) mice (10- to 12-week-old) received oral administration of saline, oxonic acid (OA), or OA plus inosine (Ino) twice (1-day moderate sUA supplementation). (A–D) Effect of 1-day sUA supplementation on plasma sUA (A) and whole blood NAD+ (B), nicotinamide mononucleotide (NMN) (C), and cyclic ADP-ribose (cADPR) (D) levels in WT and CD38 KO mice (WT-Saline: n=8 mice, WT-OA: n=9 mice, WT-OA+Ino: n=9 mice, KO-Saline: n=6 mice, KO-OA: n=8 mice, KO-OA+Ino: n=8 mice). (E) Effect of 1-day or 3-day release on whole blood NAD+, NMN, cADPR, and plasma sUA levels in WT mice that received 1-day supplementation (WT-Saline: n=6 mice, WT-OA: n=8 mice, WT-OA+Ino: n=8 mice). (F) Effect of sUA (100, 200, or 500 μM) and other ligands (analogs at 500 μM, 78c, a CD38 inhibitor, at 0.5 μM) on NAD+ degradation by recombinant hCD38 (n=5 independent samples). Data are mean ± s.e.m. Significance was tested using two-way ANOVA (A–D), Kruskal-Wallis test, or one-way ANOVA with Tukey’s multiple comparisons test (E and F). NS, not significant. Statistical difference (A–D) between OA and OA+Ino groups in WT or KO mice (saline alone group excluded) was also analyzed by two-tailed unpaired t-test or Mann-Whitney test; WT mice: p<0.0001 (A), p=0.0056 (B), or 0.0351 (C); KO mice: p=0.0003 (A).

Effect of soluble uric acid (sUA) on intracellular NAD+ levels in A549 and THP-1 cells.
(A) Effect of sUA on intracellular NAD+ levels of A549 cells (n=5 biologically independent samples). (B) Effect of sUA pre-incubation on intracellular NAD+ levels of THP-1 cells. Naïve THP-1 cells were incubated with sUA (0–10 mg/dL) for 2 hr, then the cells were washed twice with sterile PBS and stimulated with monosodium urate (MSU) crystals (200 μg/mL), crude lipopolysaccharide (cLPS) (20 μg/mL), zymosan (50 μg/mL), or ATP (2 mM) for 6 hr (n=6 biologically independent samples). Data are mean ± s.e.m. Significance was tested using one-way ANOVA with Dunnett’s multiple comparisons test (A and B) or Kruskal-Wallis test (cLPS groups in B).

Effect of soluble uric acid (sUA) and its precursors and metabolite on nicotinamide phosphoribosyltransferase (NAMPT) and poly(ADP-ribose) polymerase (PARP) activities.
(A and B) NAMPT activity (n=4 technical replicates). (C and D) PARP activity. 3-Aminobenzamide is a PARP inhibitor (n=4 technical replicates). Data are mean ± s.d. (A and C) or mean ± s.e.m. (B and D).

Effect of oxonic acid (OA) on CD38 activity and plasma soluble uric acid (sUA) levels.
(A and B) Hydrolase and cyclase activities of lung tissues from wild-type (WT) mice in the presence of OA (0–5 mM) (n=3 experiments/technical replicates). (C) Effect of OA administration on plasma sUA levels in WT mice (10- to 12-week-old) that received oral administration of inosine. In saline group, the mice received oral administration and intraperitoneal injection of saline. In OA p.o. group, the mice received oral administration of inosine (1.5 g/kg) and OA (1.5 g/kg) (the same treatment in our models), and intraperitoneal injection of saline. In OA i.p. group, the mice received oral administration of inosine (1.5 g/kg), and intraperitoneal injection of OA (0.25 g/kg). Four hours after treatment, plasma sUA was measured (n=5–8 mice per group). Data are mean ± s.d. (A and B) or mean ± s.e.m. (C). Significance was tested using Brown-Forsythe and Welch ANOVA tests (C).

Effect of soluble uric acid (sUA) at physiological levels on NAD+ degradation under inflammatory conditions.
(A–D) Effect of 1-day sUA supplementation on plasma sUA (A) and whole blood NAD+ (B), nicotinamide mononucleotide (NMN) (C), and cyclic ADP-ribose (cADPR) (D) levels in wild-type (WT) and CD38 knockout (KO) mice (10- to 12-week-old) under inflammatory conditions. The mice received 1-day sUA supplementation. Two hours after the last treatment, the mice were intraperitoneally stimulated with sterile PBS or crude lipopolysaccharide (cLPS) (2 mg/kg) for 6 hr (WT-oxonic acid [OA]: n=6 mice, WT-OA+cLPS: n=11 mice, WT-OA+Ino+cLPS: n=12 mice, KO-OA: n=6 mice, KO-OA+cLPS: n=8 mice, KO-OA+Ino+cLPS: n=8 mice). (E–H) Effect of 1-day treatment of OA or inosine (Ino) on plasma sUA (E) and whole blood NAD+ (F), NMN (G), and cADPR (H) levels in WT mice (10- to 12-week-old) under inflammatory conditions. The mice received 1-day treatment of OA or Ino (from the evening of day 0 to the morning of day 1). Two hours after the last treatment, the mice were intraperitoneally stimulated with sterile PBS or cLPS (2 mg/kg) for 6 hr (n=6 mice in Saline+cLPS group, n=5 mice in other groups). Data are mean ± s.e.m. Significance was tested using two-way ANOVA with Tukey’s multiple comparisons test (A–D), or one-way ANOVA with Tukey’s multiple comparisons test (E–H). Statistical difference (A–D) between OA+cLPS and OA+Ino+cLPS groups in WT or KO mice (OA alone group excluded) was also analyzed by two-tailed unpaired t-test (with Welch’s correction when applicable) or Mann-Whitney test; WT mice: p<0.0001 (A), or p=0.0092 (B), 0.0221 (D); KO mice: p=0.0004 (A).

Effect of 1-, 3-, and 7-day soluble uric acid (sUA) supplementation on NAD+, nicotinamide mononucleotide (NMN), and sUA levels in whole blood and tissues.
Wild-type (WT) and CD38 knockout (KO) mice (10- to 12-week-old) received oral administration of saline, oxonic acid (OA), or OA plus inosine (Ino) twice daily for 1, 3, or 7 days. Four hours after the last treatment, the mice were sacrificed. In 1-day model, the mice were treated from the evening of day 0 to the morning of day 1. (A) Effect of 1-day sUA supplementation on tissue NAD+, NMN, and sUA levels (n=5 male mice per group). (B) Effect of 3-day sUA supplementation on tissue NAD+, NMN, and sUA levels (n=5 male mice per group). (C–F) Effect of 3-day sUA supplementation on plasma sUA (C), whole blood NAD+ (D), NMN (E), and cyclic ADP-ribose (cADPR) (F) levels (WT-Saline: n=6 mice, WT-OA: n=8 mice, WT-OA+Ino: n=8 mice, KO-Saline: n=6 mice, KO-OA: n=8 mice, KO-OA+Ino: n=8 mice). (G–I) Effect of 7-day sUA supplementation on tissue NAD+ and sUA levels (n=5 male mice per group). Data are mean ± s.e.m. Significance was tested using two-way ANOVA with Tukey’s multiple comparisons test (A–H), Kruskal-Wallis test or one-way ANOVA with Dunnett’s multiple comparisons test (I). Statistical difference (C–H) between OA and OA+Ino groups in WT or KO mice (saline alone group excluded) was also analyzed by two-tailed unpaired t-test; WT mice: p<0.0001 (C), or p=0.0058 (D), 0.0453 (E), 0.0083 (G, left panel), 0.0036 (H, left panel); KO mice: p=0.0004 (C).

No effect of 1- to 7-day soluble uric acid (sUA) supplementation on serum IL-1β production.
Wild-type (WT) and CD38 knockout (KO) mice (10- to 12-week-old) received oral administration of saline, oxonic acid (OA), or OA plus inosine (Ino) twice daily for 1, 3, or 7 days. Four hours after the last treatment, serum was collected for IL-1β measurement (n=5 male mice per group).
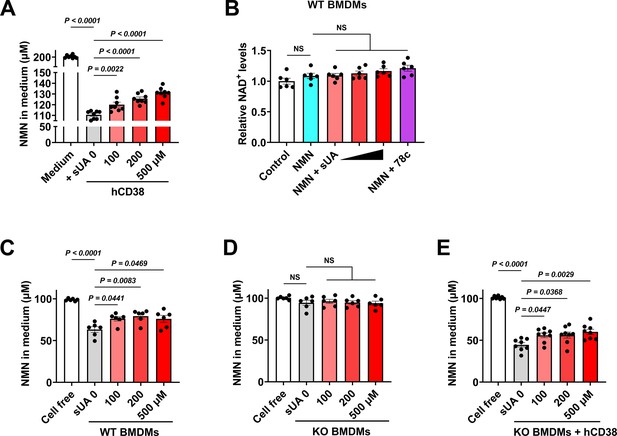
Soluble uric acid (sUA) limits nicotinamide mononucleotide (NMN) degradation via CD38 inhibition.
(A) Effect of sUA on recombinant hCD38-mediated NMN (200 μM) degradation in medium (n=8 independent samples). (B) Effect of sUA (100, 200, and 500 μM) or 78c (0.5 μM) on intracellular NAD+ levels of wild-type (WT) bone marrow-derived macrophages (BMDMs) treated with NMN. WT BMDMs were primed with 100 ng/mL ultrapure lipopolysaccharide (LPS) for 8 hr (n=6 biologically independent samples). (C–E) Effect of sUA on extracellular NMN degradation in WT (C) or CD38 KO BMDMs in the absence (D) or presence (E) of recombinant hCD38 (10 ng/mL). BMDMs were primed with 100 ng/mL ultrapure LPS for 8 hr before metabolic assays (n=6 biologically independent samples in C and D), n=8 biologically independent samples in (E). Data are mean ± s.e.m. Significance was tested using one-way ANOVA with Tukey’s multiple comparisons test.
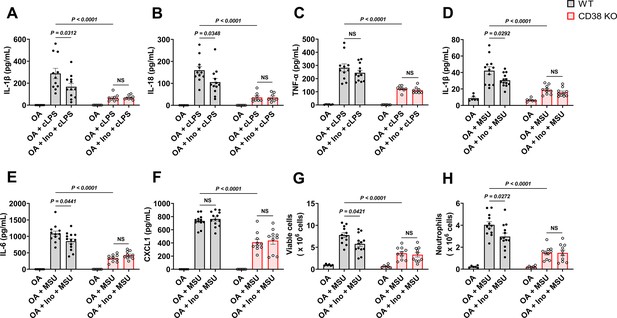
Soluble uric acid (sUA) physiologically prevents excessive inflammation by interacting with CD38.
Wild-type (WT) and CD38 knockout (KO) mice (10- to 12-week-old) received 1-day moderate sUA supplementation, plasma sUA was increased to the minimum physiological levels of humans in oxonic acid (OA) plus inosine (Ino) groups. Then, the mice were stimulated with crude lipopolysaccharide (cLPS) (2 mg/kg) or monosodium urate (MSU) crystals (2 mg/mouse) for 6 hr. (A–C) Effect of sUA at physiological levels on serum levels of IL-1β (A), IL-18 (B), and TNF-α (C) in mice with cLPS-induced systemic inflammation (WT-OA: n=6 mice, WT-OA+cLPS: n=11 mice, WT-OA+Ino+cLPS: n=12 mice, KO-OA: n=6 mice, KO-OA+cLPS: n=8 mice, KO-OA+Ino+cLPS: n=8 mice). (D–H) Effect of sUA at physiological levels on IL-1β (D), IL-6 (E), and CXCL1 (F) levels and recruitment of viable cells (red blood cells excluded) (G) and neutrophils (H) in peritoneal lavage fluid from the mice with MSU crystal-induced peritonitis (WT-OA: n=6 mice, WT-OA+MSU: n=12 mice, WT-OA+Ino+MSU: n=13 mice, KO-OA: n=6 mice, KO-OA+MSU: n=10 mice, KO-OA+Ino+MSU: n=10 mice). Data are mean ± s.e.m. Significance was tested using two-way ANOVA with Tukey’s multiple comparisons test. NS, not significant. Statistical difference between OA+cLPS (or MSU) and OA+Ino+cLPS (or MSU) groups in WT or KO mice (OA alone group excluded) was also analyzed by two-tailed unpaired t-test (with Welch’s correction when applicable) or Mann-Whitney test; WT mice: p=0.0398 (A), 0.0383 (B), 0.0407 (D), 0.0417 (E), 0.0222 (G), or 0.0205 (H).
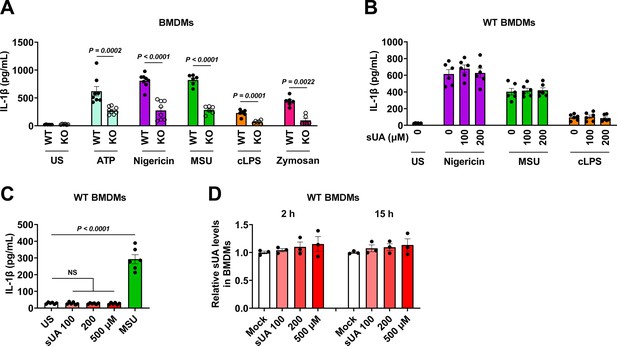
Effect of CD38 knockout (KO) or soluble uric acid (sUA) pre-incubation on IL-1β release in primed bone marrow-derived macrophages (BMDMs).
(A) Effect of CD38 KO on IL-1β release in primed BMDMs. Wild-type (WT) and KO BMDMs were primed with 100 ng/mL ultrapure lipopolysaccharide (LPS) for 4 hr, then primed BMDMs were challenged by ATP (5 mM, 30 min), nigericin (3 μM, 2 hr), monosodium urate (MSU) crystals (200 μg/mL, 6 hr), crude LPS (cLPS) (20 μg/mL, 6 hr), and zymosan (50 μg/mL, 4 hr). US means unstimulated (n=8 biologically independent samples in ATP and nigericin groups, n=6 biologically independent samples in other groups). (B–D) Effect of sUA pre-incubation on IL-1β release and intracellular sUA levels in primed BMDMs. WT BMDMs were primed with 100 ng/mL ultrapure LPS for 4 hr. (B) The cells were pre-incubated with or without sUA (100 or 200 μM) for 2 hr. Then, the cells were washed twice with sterile PBS and were stimulated with nigericin (3 μM, 2 hr), MSU crystals (200 μg/mL, 4 hr), or cLPS (1 μg/mL, 4 hr). (C and D) Primed BMDMs were directly incubated with sUA (100, 200, and 500 μM) or MSU crystals (100 μg/mL) for 6 hr in C, 2 or 15 hr in D. US means unstimulated (n=6 biologically independent samples in B and C, n=3 biologically independent samples in D). Data are mean ± s.e.m. Significance was tested using two-tailed unpaired t-test and Mann-Whitney test (ATP and zymosan) (A), or one-way ANOVA with Dunnett’s multiple comparisons test (B–D).

Effect of soluble uric acid (sUA) pre-incubation and CD38 blockade on IL-1β release in primed THP-1 cells.
THP-1 cells were primed with 0.5 μM phorbol 12-myristate 13-acetate (PMA) for 3 hr the day before stimulation. (A) Primed THP-1 cells were pre-incubated with sUA (0–10 mg/dL) for 2 hr, then the cells were washed twice with sterile PBS and challenged by monosodium urate (MSU) crystals (200 μg/mL), crude lipopolysaccharide (cLPS) (20 μg/mL), zymosan (50 μg/mL), and ATP (2 mM) for 4 hr (n=6 biologically independent samples). (B and C) Primed THP-1 cells were treated without or with 2 μM 78c (CD38 blockade by the highly specific and potent inhibitor of CD38) for 2 hr. Then, the cells were pre-incubated with blank medium or sUA for 2 hr. The cells were washed twice with sterile PBS. The washed cells were challenged by MSU crystals (200 μg/mL, 4 hr) for IL-1β measurement (B) or were directly used for sUA uptake measurement without MSU crystal stimulation (C). 78c was used in all steps for CD38 blockade groups, including sUA pre-incubation and MSU crystal stimulation (n=6 biologically independent samples). Data are mean ± s.e.m. Significance was tested using Kruskal-Wallis test (ATP groups in A), one-way ANOVA with Dunnett’s multiple comparisons test (A), or two-way ANOVA with Tukey’s multiple comparisons test (B).

Crystal precipitation in high-concentration soluble uric acid (sUA) stock solutions after long-term storage.
sUA stock solutions in NaOH were prepared without pH adjustment. Crystals were immediately precipitated after dissolution in 50 mg/mL tube. Visible crystals were observed in 5 mg/mL tube after 2-month storage at 4°C (before taking the picture, the tube was slightly shaken to resuspend the crystals on the bottom).

One-day soluble uric acid (sUA) supplementation fails to prevent high-dose crude lipopolysaccharide (cLPS)-induced systemic inflammation.
Wild-type (WT) mice (10- to 12-week-old) received 1-day treatment of oxonic acid (OA) or OA plus inosine (Ino), 2 hr after the last treatment, the mice were intraperitoneally stimulated with sterile PBS or cLPS (20 mg/kg) for 4 hr (n=5 mice per group). (A–C) Serum IL-1β (A), IL-18 (B), and TNF-α (C) were measured. (D–G) Plasma sUA (D), whole blood NAD+ (E), nicotinamide mononucleotide (NMN) (F), and cyclic ADP-ribose (cADPR) (G) levels were measured. Data are mean ± s.e.m. Significance was tested using one-way ANOVA with Tukey’s multiple comparisons test (A and C–G) or Kruskal-Wallis test (B).

Oxonic acid (OA) or inosine (Ino) alone does not limit crude lipopolysaccharide (cLPS)-induced systemic inflammation and monosodium urate (MSU) crystal-induced peritonitis.
Wild-type (WT) mice (10- to 12-week-old) received 1-day oral administration of saline, OA, or Ino, 2 hr after the last treatment, the mice were intraperitoneally stimulated with sterile PBS, cLPS (2 mg/kg), or monosodium urate (MSU) crystals (2 mg/mouse) for 6 hr. (A–C) Serum IL-1β (A), IL-18 (B), and TNF-α (C) levels in mice with cLPS-induced systemic inflammation were measured (n=6 mice in Saline+cLPS group, n=5 mice in other groups). (D–H) IL-1β (D), IL-6 (E), CXCL1 (F), and the number of viable cells (red blood cells excluded) (G) and neutrophils (H) in peritoneal lavage fluid from the mice with MSU crystal-induced peritonitis were measured (n=5 mice per group). Data are mean ± s.e.m. Significance was tested using one-way ANOVA with Tukey’s (A, C–H) multiple comparisons test, or Kruskal-Wallis test (B).

Potential mechanism of the paradox in gout therapy (urate-lowering therapy).
The soluble uric acid (sUA)-monosodium urate (MSU) crystal curve in this figure was also described in our previous review article. The curve was minorly adapted and was permitted to be published in this eLife paper.
© 2024, Elsevier. Reprinted from Figure 4D in Wen et al., 2024 with permission from Elsevier; further reproduction of this figure would need permission from the copyright holder.
Tables
Reagent type (species) or resource | Designation | Source or reference | Identifiers | Additional information |
---|---|---|---|---|
Chemical compound, drug | Uric acid | Sigma-Aldrich | Cat#U0881 | |
Chemical compound, drug | β-Nicotinamide Adenine Dinucleotide (NAD+) | Nacalai | Cat#24338-86 | |
Chemical compound, drug | β-Nicotinamide mononucleotide (NMN) | BLD Pharmatech | Cat#BD116593 | |
Chemical compound, drug | cADP-Ribose (cADPR) | Santa Cruz | Cat#sc-201512 | |
Chemical compound, drug | FYU-981 | Fuji Yakuhin Co., Ltd. | N/A | |
Chemical compound, drug | 78c | Sigma-Aldrich | Cat#5.38763 | |
Chemical compound, drug | N-Cyclohexyl benzamide | Sigma-Aldrich | Cat#R531332 | |
Chemical compound, drug | Inosine | Nacalai | Cat#07139-42 | |
Chemical compound, drug | Hypoxanthine | Sigma-Aldrich | Cat#H-9636 | |
Chemical compound, drug | Xanthine | Sigma-Aldrich | Cat#X0626 | |
Chemical compound, drug | Allantoin | Sigma-Aldrich | Cat#A-7878 | |
Chemical compound, drug | Adenosine | Wako | Cat#010-10491 | |
Chemical compound, drug | Guanosine | Wako | Cat#079-01111 | |
Chemical compound, drug | Uracil | FUJIFILM Wako | Cat#212-00062 | |
Chemical compound, drug | 1,3-Dihydroimidazol-2-one | BLD Pharmatech | Cat#BD00733674 | |
Chemical compound, drug | Oxypurinol | Sigma-Aldrich | Cat#O-6881 | |
Chemical compound, drug | Caffeine | FUJIFILM Wako | Cat#031-06792 | |
Chemical compound, drug | 1-Methyluric acid | Sigma-Aldrich | Cat#M6885 | |
Chemical compound, drug | 1,3-Dimethyluric acid | Santa Cruz | Cat#sc-206240 | |
Chemical compound, drug | 1,7-Dimethyluric acid | Cayman Chemical | Cat#19584 | |
Chemical compound, drug | 1,3,7-Trimethyluric acid | Cayman Chemical | Cat#16949 | |
Chemical compound, drug | 8-Oxoguanine | Cayman Chemical | Cat#89290 | |
Chemical compound, drug | Oxonic acid potassium salt | Sigma-Aldrich | Cat#156124 | |
Chemical compound, drug | LPS from Escherichia coli 0111:B4 (crude LPS) | Sigma-Aldrich | Cat#L4130 | |
Chemical compound, drug | Ultrapure LPS from Escherichia coli 0111:B4 | InvivoGen | Cat#tlrl-3pelps | |
Chemical compound, drug | Phorbol 12-Myristate 13-Acetate | FUJIFILM Wako | Cat#162-23591 | |
Chemical compound, drug | Nigericin | Sigma-Aldrich | Cat#N-7143 | |
Chemical compound, drug | ATP | Oriental Yeast | Cat#45142000 | |
Chemical compound, drug | Zymosan A from Saccharomyces cerevisiae | Sigma-Aldrich | Cat#Z4250 | |
Chemical compound, drug | Nicotinamide 1, N6-ethenoadenine dinucleotide (ε-NAD+) | Sigma-Aldrich | Cat#N2630 | |
Chemical compound, drug | Nicotinamide guanine dinucleotide (NGD) | Sigma-Aldrich | Cat#N5131 | |
Peptide, recombinant protein | Recombinant human CD38 protein | Sino Biological | Cat#10818-H08H | |
Peptide, recombinant protein | Recombinant human M-CSF protein | Proteintech Group | Cat#HZ-1192 | |
Commercial assay or kit | Human IL-1β ELISA Kit | R&D Systems | Cat#DY201 | |
Commercial assay or kit | Mouse IL-1β ELISA Kit | R&D Systems | Cat#SMLB00C | |
Commercial assay or kit | Mouse IL-6 ELISA Kit | R&D Systems | Cat#DY406-05 | |
Commercial assay or kit | Mouse IL-18 ELISA Kit | R&D Systems | Cat#DY7625-05 | |
Commercial assay or kit | Mouse TNF-α ELISA Kit | R&D Systems | Cat#DY410-05 | |
Commercial assay or kit | Mouse CXCL1 ELISA Kit | R&D Systems | Cat#DY453-05 | |
Commercial assay or kit | Wright-Giemsa Stain Kit | ScyTek Laboratories | Cat#WGK-1 | |
Commercial assay or kit | CycLex NAMPT Colorimetric Assay Kit Ver.2 | MBL | Cat#CY-1251V2 | |
Commercial assay or kit | HT Universal Colorimetric PARP Assay Kit | R&D Systems | Cat#4677-096-K | |
Cell line (human) | A549 | ATCC | CCL-185 | |
Cell line (human) | THP-1 | ATCC | TIB-202 | |
Cell line (mouse) | ICR bone marrow-derived macrophages | This paper | N/A | See more details in Preparation of BMDMs section |
Strain, strain background (mouse) | ICR mice | Japan SLC, Inc | N/A | |
Strain, strain background (mouse) | CD38 KO ICR mice | Dr. Haruhiro Higashida, Kanazawa University | N/A | |
Software, algorithm | Prism 9 | GraphPad Software | https://www.graphpad.com | |
Software, algorithm | Labsolutions software | Shimadzu | https://www.shimadzu.com | |
Other | RF-6000 Spectrofluorophotometer | Shimadzu | https://www.shimadzu.com | For the measurement of CD38 activity |
Other | LC-30A system | Shimadzu | https://www.shimadzu.com | For LC-MS/MS analysis |
Other | LCMS-8050 | Shimadzu | https://www.shimadzu.com | For LC-MS/MS analysis |
Additional files
-
Supplementary file 1
Comparison between Ki values and mean levels of soluble uric acid (sUA) in different tissues.
- https://cdn.elifesciences.org/articles/96962/elife-96962-supp1-v1.docx
-
Supplementary file 2
The data not shown in enzyme assays.
- https://cdn.elifesciences.org/articles/96962/elife-96962-supp2-v1.xlsx
-
Supplementary file 3
The raw images of chemical structures.
- https://cdn.elifesciences.org/articles/96962/elife-96962-supp3-v1.pptx
-
MDAR checklist
- https://cdn.elifesciences.org/articles/96962/elife-96962-mdarchecklist1-v1.docx