A synthetic method to assay polycystin channel biophysics
Figures
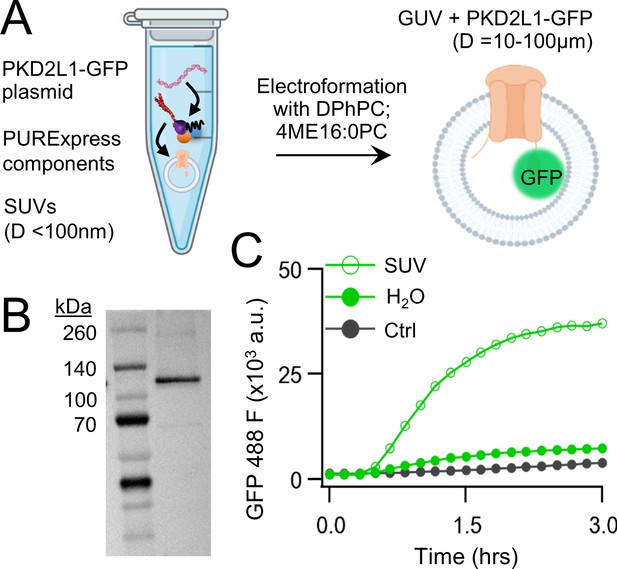
Cell-free expression of synthetic PKD2L1 protein and incorporation into lipid vesicles.
(A) Schematic of cell-free protein expression into synthetic lipid vesicles and subsequent electroformation with Vesicle Prep Pro (Nanion). (B) Full-length PKD2L1-GFP protein detected by western blot after cell-free expression into vesicles. (C) Monitored fluorescence over time of cell-free expressed PKD2L1-GFP and a non-fluorescent control plasmid produced in the presence or absence of lipid vesicles.
-
Figure 1—source data 1
Original file for western blot analysis displayed in Figure 1B.
- https://cdn.elifesciences.org/articles/98534/elife-98534-fig1-data1-v1.zip
-
Figure 1—source data 2
PDF file containing original western blot for Figure 1B, indicating the relevant bands and treatments.
- https://cdn.elifesciences.org/articles/98534/elife-98534-fig1-data2-v1.zip
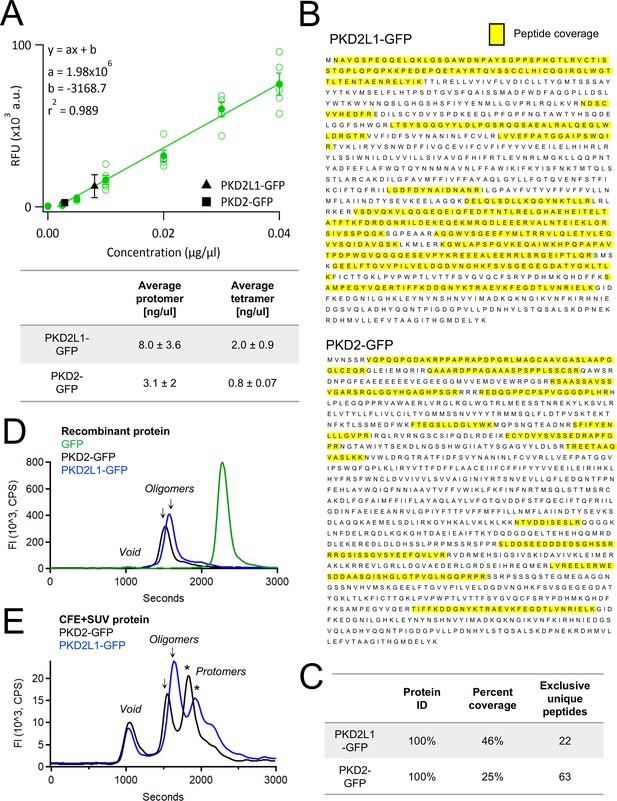
Quantification, identification, and assembly of cell-free synthesized polycystin channels.
(A) Top, fluorescence (488γ) standard curve determined with a recombinant GFP-tagged protein fit with a linear regression. PURExpress synthesized PKD2L1-GFP and PKD2-GFP were measured after 3 hr of expression at 37°C. (GFP standard curve N = 5, PKD2L1 and PKD2 N = 3 replicates). Bottom, average protomer and tetramer protein production from the PURExpress reaction. Error bars represent SEM. (B) Representative sequence coverage of PKD2L1-GFP (top) and PKD2-GFP (bottom) from tandem mass spectrometry spectra. (C) Mass spectrometry outputs identifying polycystin proteins. (D, E) Fluorescence-detection size-exclusion chromatography (FSEC) of polycystin proteins derived from recombinant and cell-free protein expression (CFE) sources. Recombinant human PKD2-GFP and PKD2L1-GFP protein was obtained from lysates of 0.5 × 106 HEK cells stably expressing the channels. Purified Aequorea Victoria GFP His-tag protein was obtained from Thermo Fisher Scientific. SUVs containing CFE-derived polycystins were lysed using dodecyl β-D-maltoside (DDM) prior to FSEC analysis (see methods).
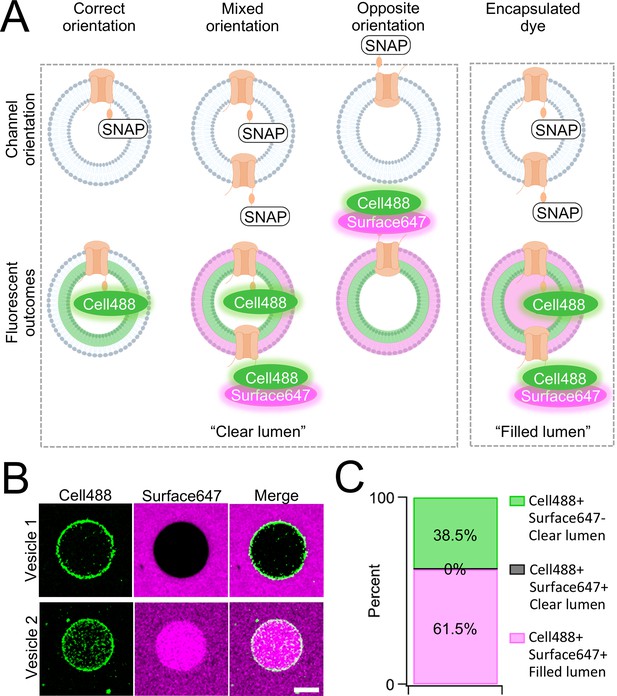
Orientation of synthetic PKD2L1 channels in vesicles.
(A) Schematic of possible ion channel orientation outcomes from PKD2L1 cell-free expression (top) and hypothesized fluorescence results when Cell488 and Surface647 added (bottom). (B) Fluorescent confocal images from the SNAP-tagged vesicles. The scale bar represents 10 μm for all images. (C) Vesicle percentage depicts the percent of vesicles with each fluorescent output (N = 65 vesicles).
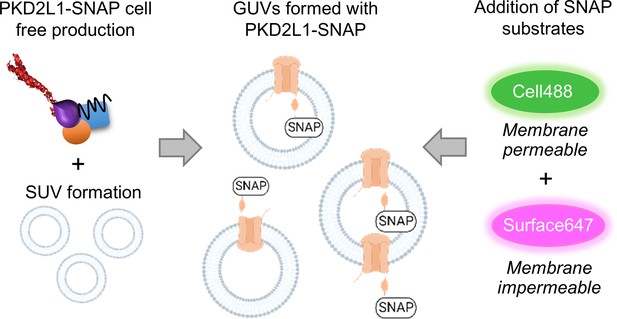
The SNAP-fluorescence approach to assess polycystin protein orientation in giant unilamellar vesicle (GUV).
Schematic of PKD2L1-SNAP incorporated into GUVs, followed by SNAP staining with cell permeable (Cell488), and cell impermeable (Surface647) SNAP-Tag marker.
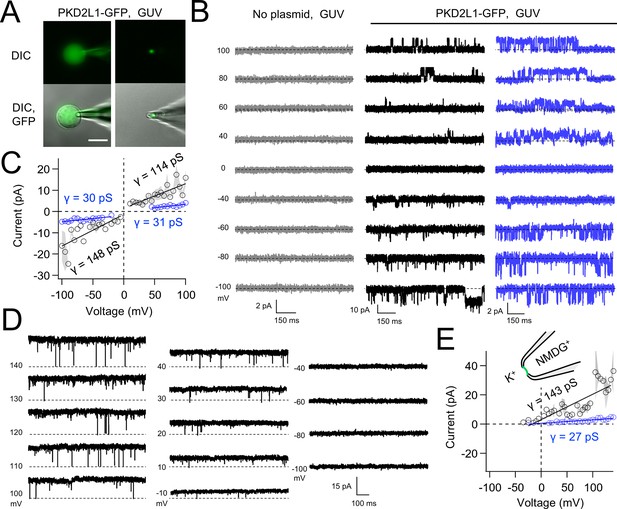
Synthetic PKD2L1 channels are functional in artificial membranes.
(A) Images of voltage-clamped giant unilamellar vesicles (GUVs) with incorporated PKD2L1-GFP channels. Left, establishing high-resistance seals in the on-cell patch configuration. Right, transitioning to the inside-our patch configuration. Scale bar = 20 μm. (B) Example unitary single-channel current records from GUVs reconstituted with or without PKD2L1 protein. Vesicles were patched using the symmetrical 150 mM K+ conditions (see methods) and PKD2L1 single-channel current producing full and sub-conductances are colored black and blue, respectively. (C) Average single-channel current amplitudes. Conductance (γ) estimated by fitting the average single-channel currents to a linear equation. Error (gray) indicates SEM from N = 3–8 GUVs. Several replicates lacked single-channel openings at all test potentials. (D) PKD2L1 single-channel current recorded using asymmetric cationic solutions, with 150 mM K+ in the bath and 150 mM NMDG+ in the pipette. (E) Resulting average single-channel current amplitudes where no inward single-channel current was detected (N = 3–4 GUVs).
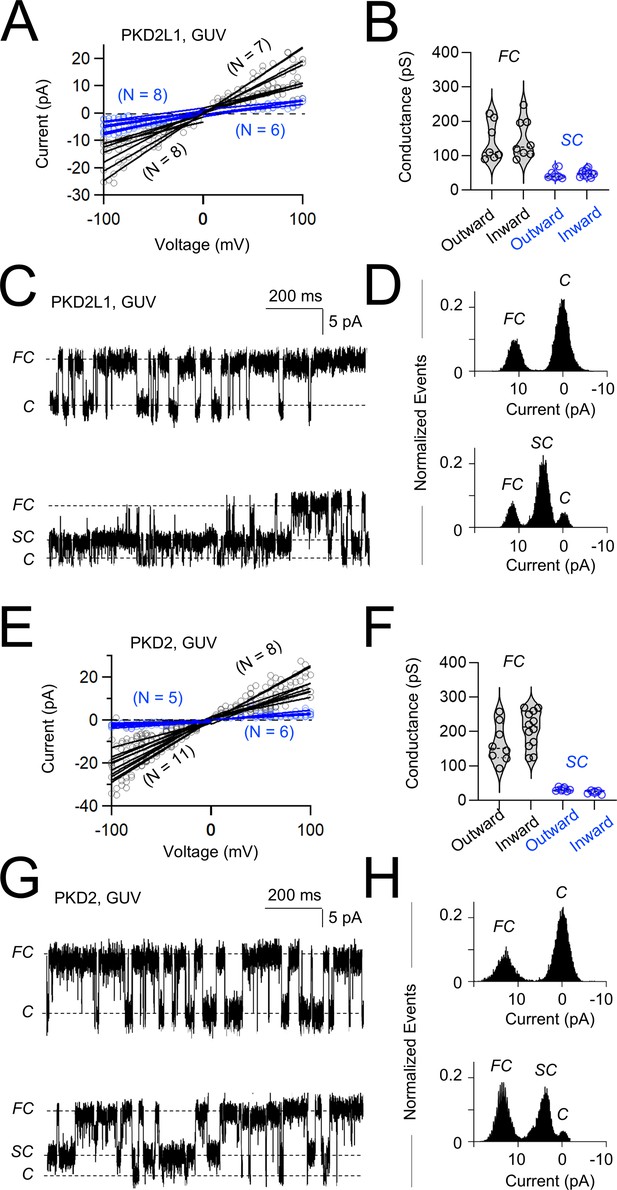
Synthetic polycystin channels exhibit full and sub-conductive states in giant unilamellar vesicles (GUVs).
(A, E) Single-channel current amplitudes measured from individual GUVs (open circles). GUVs with open channel events measure at four or more potentials in inward and outward direction were fit to a linear equation to estimate their conductance. (B, F) Resulting violin plots of the sub- (SC, blue) and full (FC, black) polycystin conductance as estimated from individual GUV recordings (N = 5–7 GUVs). (C, G) Unitary single-channel currents measured from GUVs expressing polycystins held at 100 mV. Examples on the top demonstrated channels transitioning from closed (C) to the full conductance current levels, whereas examples below are measured from channels shifting between sub- and fully conductive current levels. (D, H) Resulting histogram analysis of the corresponding single-channel currents.
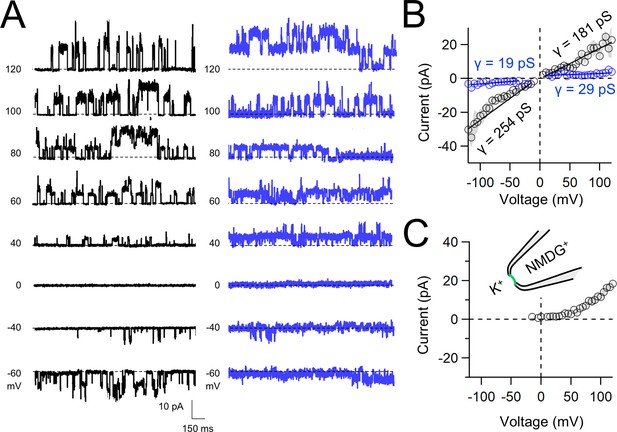
Functional synthetic PKD2 channels in artificial membranes.
(A) Example unitary single-channel current records from giant unilamellar vesicles (GUVs) reconstituted with PKD2 protein in symmetrical 150 mM K+ conditions producing full (black traces) and sub-conductances (blue traces), respectively. (B, C) Average single-channel current amplitudes recorded using K+ or NMDG+ in the recording electrode solution. Conductance (γ) estimated by fitting the average single-channel currents to a linear equation. Error (gray) indicate SEM from K+ (N = 3–12 GUVs) and NMDG+ (N = 2–5 GUVs) conditions.
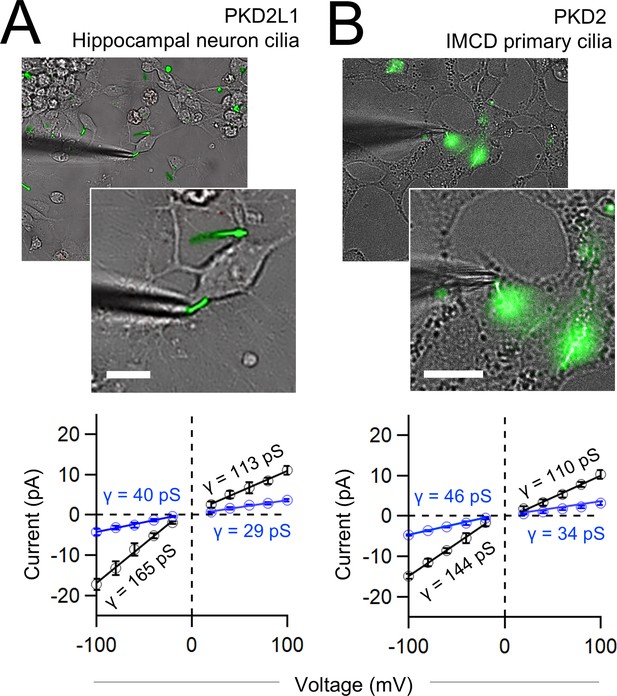
Native polycystin channels measured from primary cilia membranes exhibit full and sub-conductance states.
(A, B) Top, images of voltage-clamped primary cilia from mouse hippocampal neurons and inner medullary kidney collecting duct cells (IMCD) harvested from transgenic mice expressing a fluorescent cilia reporter (ARL13B-EGFPtg) (Delling et al., 2013). Scale bar = 20 μm. Previous work has genetically identified PKD2L1 and PKD2 as essential ion channel subunit in the primary cilia of the renal collecting duct cells and hippocampal neurons (Vien et al., 2023; Kleene and Kleene, 2017). Bottom, average single-channel current amplitudes recorded from primary cilia using K+ in the recording electrode solution. Conductance (γ) estimated by fitting the average single-channel currents to a linear equation. Error indicates SEM (N = 6 cilia).
Tables
Conductance properties of polycystins measured from giant unilamellar vesicle (GUV) and cilia membranes.
Polycystin channel and membrane context | Major K+ g (pS) ± SD | Minor K+ g (pS) ± SD | ||
---|---|---|---|---|
Inward | Outward | Inward | Outward | |
PKD2 (GUV membrane, cell-free expression) | 282 ± 38 | 153 ± 32 | 23 ± 4 | 21 ± 3 |
PKD2 (primary cilia membrane, endogenous murine inner medullary collecting duct [IMCD] cell line) | 144 ± 9 | 110 ± 6 | 46 ± 4 | 34 ± 4 |
PKD2L1 (GUV membrane, cell-free expression) | 148 ± 29 | 114 ± 28 | 30 ± 6 | 31 ± 7 |
PKD2L1 (primary cilia membrane, endogenous hippocampal neurons) | 165 ± 10 | 113 ± 6 | 40 ± 4 | 29 ± 3 |