Kidney Fibrosis: Lock out SIRT4
Chronic kidney disease is estimated to affect over 10% of the global population (Kovesdy, 2022). Its most common feature is kidney fibrosis, which is characterized by the accumulation of extracellular matrix proteins in tissues, causing structural damage that can impair the function of the kidneys (Huang et al., 2023).
Previous work revealed that complex mechanisms and protein mediators drive kidney fibrosis, including the protein TGF-β1, which plays a vital role in promoting the expression of pro-fibrotic genes. TGF-β1 acts through a well-established signaling pathway that eventually activates a set of three transcription factors – Smad2, Smad3 and Smad4 – which collectively move from the cytoplasm to the nucleus where they promote the transcription of pro-fibrotic genes (Meng et al., 2016). The transport of molecules from the cytoplasm to the nucleus occurs continuously in cells throughout the body and must be tightly controlled to prevent aberrant traffic that can drive disease.
However, directly inhibiting TGF-β1 signaling to prevent kidney fibrosis is not an ideal therapeutic strategy due to the potential adverse effects of disrupting other biological processes involving TGF-β1. Therefore, a greater understanding of the mechanisms underlying TGF-β1-induced fibrosis is required to identify alternative approaches. Now, in eLife, Lin Kang, Zhen Liang, Shu Yang and colleagues – including Guangyan Yang, Jiaqing Xiang and Xiaoxiao Yang as joint first authors – report how unusual TGF-β1-driven translocation of the protein Sirtuin 4 into the nucleus contributes to the progression of kidney fibrosis (Yang et al., 2024).
Sirtuin 4 (also known as SIRT4 for short) is normally located in the mitochondria. However, Yang et al. (who are based at the First Affiliated Hospital of Southern University of Science and Technology and Hefei University of Technology) found increased amounts of SIRT4 in the nuclei of kidney cells from patients with chronic kidney disease. Similarly, they also discovered elevated levels of nuclear SIRT4 in the kidneys of mice that had been injured during surgery to induce kidney fibrosis experimentally.
Intriguingly, Yang et al. found that deleting the gene for SIRT4 significantly reduced the extent of kidney fibrosis in mice following an injury; this occurred either globally throughout the body or specifically in the epithelial cells lining tubules in the kidney. Consistent with this finding, overexpressing the gene in the same cells markedly enhanced the extent of kidney fibrosis. Taken together, these results suggest that abnormal nuclear accumulation of SIRT4 contributes to kidney fibrosis.
To investigate the mechanism underlying SIRT4-mediated fibrosis, Yang et al. carried out experiments in human kidney tubule epithelial cells to identify which proteins interact with SIRT4 upon TGF-β1 treatment. Interestingly, SIRT4 interacted with several proteins that localized in the nucleus, including U2AF2 (short for U2 small nuclear RNA auxiliary factor 2). This protein plays an important role in assembling the molecular machinery known as a spliceosome, which removes unnecessary parts of pre-mRNA to form the mature mRNA that is translated into a protein (Zorio and Blumenthal, 1999; Agrawal et al., 2016).
Yang et al. found that TGF-β1 treatment induced SIRT4 to remove acetyl groups from U2AF2. This allowed U2AF2 to splice the pre-mRNA of a pro-fibrotic protein called CCN2 (short for cell communication network 2), causing expression of this protein to increase. Together, these findings identify the SIRT4-U2AF2-CCN2 axis as a novel mechanism of TGF-β1-induced kidney fibrosis (Figure 1).
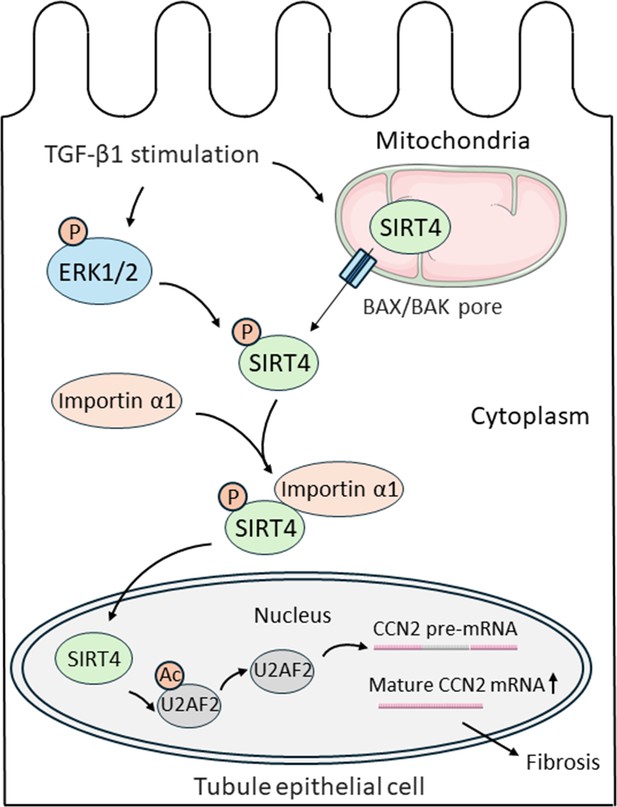
Nuclear import of SIRT4 regulates the progression of kidney fibrosis.
TGF-β1 increases the expression of genes that promote kidney fibrosis in epithelial cells lining kidney tubules (black outline). First, TGF-β1 triggers the protein SIRT4 (green) to translocate from the mitochondria (top right) to the cytoplasm through BAX/BAK pores (blue rectangles). Simultaneously, TGF-β1 also activates ERK1/2 (blue oval), which phosphorylate SIRT4 once it is in the cytosol (represented by the addition of an orange circle containing the letter P). Phosphorylated SIRT4 then binds to importin α1 (orange oval) and is transported into the nucleus (bottom) where it removes acetyl groups (orange circle containing the letters Ac) from the protein U2AF2 (grey oval). This allows U2AF2 to splice the pre-mRNA of the pro-fibrotic protein CCN2, resulting in more mature mRNA that can be translated into CCN2 and promote the progression of kidney fibrosis. BAX: B-cell lymphoma 2-associated X protein; BAK: B-cell lymphoma 2 antagonist killer 1 pore; ERK1/2: extracellular signal-regulated kinase 1 and 2; U2AF2: U2 small nuclear RNA auxiliary factor 2; CCN2: cell communication network 2.
Yang et al. next set out to explore how SIRT4 translocates from the mitochondria to the nucleus upon TGF-β1 treatment. The experiments showed that TGF-β1-induced release of SIRT4 from mitochondria occurs through pores formed by protein polymers known as BAX and BAK, which are located in the outer membrane of mitochondria (Cosentino and García-Sáez, 2017). Screening using a variety of protein inhibitors demonstrated that in order to translocate, SIRT4 must be phosphorylated by enzymes known as ERK1 and ERK2. This modification allows SIRT4 to bind to a nuclear-transport receptor called importin α1, which moves the protein into the nucleus. Finally, Yang et al. treated mice with anti-SIRT4 antibodies that only inhibit expression of SIRT4 in the nucleus, and found this targeted disruption could sufficiently alleviate surgery-induced kidney fibrosis.
These findings open an exciting avenue for the development of a novel therapeutic strategy against kidney fibrosis: to lock the ‘bad guy’ – SIRT4 – out of the nuclei of kidney cells. It is conceivable that in the future, small molecules that hinder SIRT4 from interacting with ERK1/2 or importin α1 could be screened or synthesized to treat kidney fibrosis. However, nuclear SIRT4 may play beneficial roles in other cells and tissues (Zeng et al., 2018), thus further clinical studies are needed to evaluate the safety of such a strategy.
References
-
An extended U2AF(65)-RNA-binding domain recognizes the 3’ splice site signalNature Communications 7:10950.https://doi.org/10.1038/ncomms10950
-
Bax and Bak pores: Are we closing the circle?Trends in Cell Biology 27:266–275.https://doi.org/10.1016/j.tcb.2016.11.004
-
Kidney fibrosis: from mechanisms to therapeutic medicinesSignal Transduction and Targeted Therapy 8:129.https://doi.org/10.1038/s41392-023-01379-7
-
Epidemiology of chronic kidney disease: an update 2022Kidney International Supplements 12:7–11.https://doi.org/10.1016/j.kisu.2021.11.003
-
TGF-β: the master regulator of fibrosisNature Reviews Nephrology 12:325–338.https://doi.org/10.1038/nrneph.2016.48
Article and author information
Author details
Publication history
Copyright
© 2024, Zhao and Zhou
This article is distributed under the terms of the Creative Commons Attribution License, which permits unrestricted use and redistribution provided that the original author and source are credited.
Metrics
-
- 235
- views
-
- 35
- downloads
-
- 0
- citations
Views, downloads and citations are aggregated across all versions of this paper published by eLife.
Download links
Downloads (link to download the article as PDF)
Open citations (links to open the citations from this article in various online reference manager services)
Cite this article (links to download the citations from this article in formats compatible with various reference manager tools)
Further reading
-
- Cell Biology
Membrane proteins are sorted to the plasma membrane via Golgi-dependent trafficking. However, our recent studies challenged the essentiality of Golgi in the biogenesis of specific transporters. Here, we investigate the trafficking mechanisms of membrane proteins by following the localization of the polarized R-SNARE SynA versus the non-polarized transporter UapA, synchronously co-expressed in wild-type or isogenic genetic backgrounds repressible for conventional cargo secretion. In wild-type, the two cargoes dynamically label distinct secretory compartments, highlighted by the finding that, unlike SynA, UapA does not colocalize with the late-Golgi. In line with early partitioning into distinct secretory carriers, the two cargoes collapse in distinct ER-Exit Sites (ERES) in a sec31ts background. Trafficking via distinct cargo-specific carriers is further supported by showing that repression of proteins essential for conventional cargo secretion does not affect UapA trafficking, while blocking SynA secretion. Overall, this work establishes the existence of distinct, cargo-dependent, trafficking mechanisms, initiating at ERES and being differentially dependent on Golgi and SNARE interactions.
-
- Cell Biology
- Microbiology and Infectious Disease
Zika virus (ZIKV) infection causes significant human disease that, with no approved treatment or vaccine, constitutes a major public health concern. Its life cycle entirely relies on the cytoplasmic fate of the viral RNA genome (vRNA) through a fine-tuned equilibrium between vRNA translation, replication, and packaging into new virions, all within virus-induced replication organelles (vROs). In this study, with an RNA interference (RNAi) mini-screening and subsequent functional characterization, we have identified insulin-like growth factor 2 mRNA-binding protein 2 (IGF2BP2) as a new host dependency factor that regulates vRNA synthesis. In infected cells, IGF2BP2 associates with viral NS5 polymerase and redistributes to the perinuclear viral replication compartment. Combined fluorescence in situ hybridization-based confocal imaging, in vitro binding assays, and immunoprecipitation coupled to RT-qPCR showed that IGF2BP2 directly interacts with ZIKV vRNA 3’ nontranslated region. Using ZIKV sub-genomic replicons and a replication-independent vRO induction system, we demonstrated that IGF2BP2 knockdown impairs de novo vRO biogenesis and, consistently, vRNA synthesis. Finally, the analysis of immunopurified IGF2BP2 complex using quantitative mass spectrometry and RT-qPCR revealed that ZIKV infection alters the protein and RNA interactomes of IGF2BP2. Altogether, our data support that ZIKV hijacks and remodels the IGF2BP2 ribonucleoprotein complex to regulate vRO biogenesis and vRNA neosynthesis.