The Natural History of Model Organisms: The untapped potential of medaka and its wild relatives
- Article
- Figures and data
- Abstract
- Introduction
- Medaka’s life in the wild
- Natural diversity of medaka
- The diversity of medaka’s wild relatives
- New opportunities from technological advances
- Threatened diversity
- Conclusions
- References
- Decision letter
- Author response
- Article and author information
- Metrics
Abstract
The medaka is a fish that has served as a model organism for over a century, yet there is still much to learn about its life in the wild. Here we summarize the current knowledge, highlight recent progress and outline remaining gaps in our understanding of the natural history of medaka. It has also become clear over time that rather than being a single species, medaka comprises an entire species complex, so disentangling the species boundaries is an important goal for future research. Moreover, medaka and other ricefishes exhibit striking functional diversity, little of which has been investigated to date. As such, there are opportunities to use the resources developed for medaka to study other ricefishes, and to learn more about medaka itself in an evolutionary context.
Introduction
Medaka (Oryzias latipes species complex) is a small, egg-laying freshwater fish from East Asia (Figure 1). It is often found in rice fields, which earned it its common English name of ricefish and the genus name of Oryzias (based on the genus name of rice, Oryza). The medaka has been kept as an ornamental fish in Japan from as early as the 17th century, and its central role in Japanese culture is exemplified by its appearance in pieces ranging from Edo era paintings (Kinoshita et al., 2009; Naruse et al., 2011; Takeda and Shimada, 2010) to modern-day children’s songs (Mamun et al., 2016).
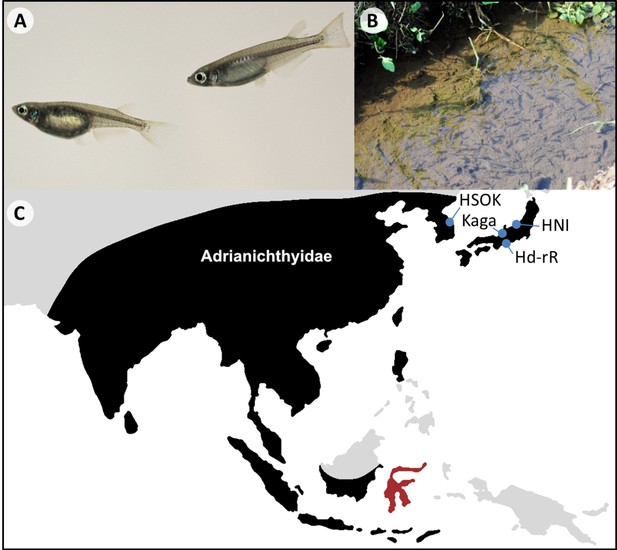
Oryzias latipes.
(A) Male (right) and female (left) Oryzias latipes from Kiyosu (Photo by Felix Loosli). Males can be easily distinguished from females by their elongated anal and dorsal fins. (B) A school of medaka in their natural habitat. (C) Approximate distributional limits of the ricefishes family, the Adrianichthyidae (black), following Parenti (2008) with the locations from which some of the most famous medaka strains are derived (blue). The Indonesian island Sulawesi represents a ricefish biodiversity hotspot (red).
The first medaka species was described as Poecilia latipes by Temminck and Schlegel (1846) in the Fauna Japonica based on collections by the German physician and naturalist Philipp Franz von Siebhold. It was reclassified in the genus Oryzias by Jordan and Snyder (1906) to become Oryzias latipes, and, more recently, it has become clear that the medaka comprises a species complex rather that a single species (Table 1).
Medaka nomenclature.
The model organism medaka comprises several species and deeply divergent lineages from the Oryzias latipes species complex. Hence, in this article we only use the term “medaka” when we collectively refer to lineages within the Oryzias latipes species complex in the context of the model system. Common or scientific species names are used to specifically refer to individual lineages as shown in this table.
Common names | Scientific name |
---|---|
Japanese medaka, Southern (Japanese) population | O. latipes |
Northern medaka, Northern (Japanese) population | O. sakaizumii |
East Korean population | N/A |
China–West Korean population | O. sinensis |
Chinese medaka | O. sinensis |
Taiwanese population, Taiwanese medaka | O. cf. sinensis |
The traditional breeding of naturally occurring color mutants led to experiments in the early 20th century that sparked the medaka’s role as a model organism. It later proved to be an ideal laboratory organism that was easy to maintain due to its small body size, and its simple dietary and habitat requirements. The combination of a sexual dimorphism, short generation times, large and transparent eggs with easily observable development, high tolerance to inbreeding and a comparably small genome size (~700 Mb) also made medaka suitable for a wide range of studies (Braasch et al., 2015; Kasahara et al., 2007; Kirchmaier et al., 2015; Parenti, 2008; Wittbrodt et al., 2002). Today, the medaka is a fully fledged model vertebrate that is deeply rooted within the life sciences (Hori, 2011; Kirchmaier et al., 2015; Shima and Mitani, 2004; Takeda and Shimada, 2010; Wittbrodt et al., 2002).
Early systematic crossing of color mutants of medaka provided evidence for Mendelian inheritance in vertebrates, sex limited inheritance and crossing over between sex chromosomes (Aida, 1921; Ishikawa, 1913; Toyama, 1916). Subsequently, the first hormone induced sex-reversal (Yamamoto, 1958) and the discovery of the first sex determining gene in a non-mammalian vertebrate (Matsuda et al., 2002) paved the way for the medaka as a model for sex determination and sex differentiation (Kondo et al., 2009). Scientific milestones achieved with the medaka further include the detection of the first active DNA-based transposable element (Tol1) in a vertebrate (Koga et al., 1995), which laid the foundation for the development of several tools for genetic modification (Koga, 2011).
Over time, medaka developed into a model for development, toxicology, carcinogenesis and behavior and a growing number of inbred strains in combination with an expanding genetic toolbox provided ever growing resources for genetic studies (reviewed in Kirchmaier et al., 2015). Medaka’s role as genomic model organism was consolidated when its genome became available as one of the first for any vertebrate (Kasahara et al., 2007). More recently, the gene-editing tool CRISPR/Cas9 was also established in medaka and employed in multiple studies (e.g. Gutierrez-Triana et al., 2018; Letelier et al., 2018; Murakami et al., 2017; Watakabe et al., 2018). In many fields, including cancer research, medaka serves as complementary model to the well-established zebrafish (Schartl and Walter, 2016; Takeda and Shimada, 2010). In particular, the natural variation captured in several inbred strains and an isogenic panel offers a unique resource to study allelic variation including disease modifier genes (Schartl and Walter, 2016; Spivakov et al., 2014).
Due to its long and successful history as a model organism, several comprehensive reviews focus on the medaka and its role in different fields of research (Kinoshita et al., 2009; Kirchmaier et al., 2015; Naruse et al., 2011; Shima and Mitani, 2004; Takeda and Shimada, 2010; Wittbrodt et al., 2002; Yamamoto, 1975). In contrast, the natural history of the medaka and its relatives has so far gained comparatively little attention. This is despite the fact that particularly Japanese biologists have been working on ricefishes for decades, which generated a more complete knowledge of O. latipes and its relatives than is available for many other fish species (Iwamatsu, 2006). However, some of this work is not available in English and thus is less accessible to the international research community. Furthermore, studies have highlighted a so far underexplored ricefish diversity in the wild (Katsumura et al., 2019; Parenti, 2008; Takehana et al., 2005; Uwa et al., 1988), which has already inspired new research agendas (e.g., Matsuda and Sakaizumi, 2016; Mokodongan et al., 2018; Spivakov et al., 2014). The combination of available medaka resources and the diversity of its wild relatives represent a treasure trove to target various aspects of biology, which will equally expand our insight into the medaka as a model system. Here, we focus on the natural history of ricefishes and point out recent progress as well as remaining potential for studies focusing on their natural diversity.
Medaka’s life in the wild
Medaka species are distributed in Japan, China, Taiwan and Korea (Shima and Mitani, 2004; Yamamoto, 1975), where they are mainly found in standing and slow-flowing water bodies such as rice paddies, ponds and agricultural channels (Fukuda et al., 2005, Figure 1B). The preferred habitats of medaka are shallow zones close to the shoreline with abundant plant cover where it feeds on microscopic organisms, including algae and zooplankton (Edeline et al., 2016; Fukuda et al., 2005). Although detailed studies on its position in the food web are lacking, medaka is likely mainly preyed upon by dragonfly larvae, birds and predatory fishes. Throughout its life, medaka is parasitized by both ectoparasites and endoparasites from across the animal kingdom (catalogued in: Nagasawa, 2017), and females appear to be particularly susceptible to ectoparasites during the breeding season (Edeline et al., 2016). In the wild, most medaka have a lifespan of roughly one year during which males and females can reach a length between three and four centimeters (Leaf et al., 2011; Parenti, 2008; Shima and Mitani, 2004; Yamamoto, 1975). In captivity, medaka may live for more than four years (Egami and Etoh, 1969). As an inhabitant of the temperate zone, medaka is confronted with and tolerates a wide range of temperatures, from 4°C to 40°C (Kinoshita et al., 2009; Sampetrean et al., 2009). When the water cools down, fish enter a state of hibernation during which they barely move and stop feeding (Edeline et al., 2016; Kinoshita et al., 2009). Cooling has been used in the lab to slow down the life cycle of medaka and store it for later use (Wittbrodt et al., 2002).
During the breeding season, medaka spawns every morning around sunrise (Leaf et al., 2011; Ueda and Oishi, 1982). Mating involves an intricate courtship dance during which males repeatedly approach females and exhibit diverse displays (Ono and Uematsu, 1957). If accepted, males hold on to the females with their elongated anal and dorsal fins, while females spawn up to 48 eggs (Leaf et al., 2011). Bundles of fertilized eggs stay attached to the mother’s ovarian cavity via attaching filaments for up to a few hours, before they are deposited (Parenti, 2005). Despite its practical use in the laboratory to easily harvest eggs from unambiguously identifiable mothers, the reasons for the evolution of this unusual reproductive strategy remain unexplored. Juveniles finally hatch after 7–9 days and grow to maturity within about two and a half months (Iwamatsu, 2004; Takeda and Shimada, 2010).
Overall, relatively little of our current knowledge on the biology of medaka has been gathered from studies in the wild. Hence, similar to other model organisms, much remains to be discovered about the ecology and life history of wild medaka populations (Box 1), and the driving evolutionary forces (Alfred and Baldwin, 2015; Leaf et al., 2011; Parichy, 2015).
Box 1.
Open questions concerning the natural history of ricefishes:
What is the general ecology of medaka species in the wild: What abiotic factors restrict their distribution; which species do they interact with and in what way?
How much gene flow exists between O. latipes and O. sakaizumii?
Which genes underlie local adaptation in the O. latipes species complex?
How connected are populations of medaka species outside of Japan?
How much ricefish diversity remains undiscovered?
What are the major threats to ricefish populations?
What mechanisms promote evolutionary divergence and functional diversity within medaka and across ricefishes in general?
What are the molecular mechanisms underlying rapid sex chromosome turnover in ricefishes and what is its role for ricefish diversity?
What are the processes underlying the remarkable ricefish diversity on Sulawesi, Indonesia?
Which evolutionary scenarios gave rise to sympatric ricefish species flocks?
How did pelvic brooding evolve: What is its physiological and genetic basis and what are the adaptive benefits?
Natural diversity of medaka
The Oryzias latipes complex
Differences between Japanese medaka populations were recognized early on (Sakaizumi, 1984), but it is only now that the scientific community has begun to fully appreciate the diversity summarized within the “medaka”. For a long time, the so-called northern and southern Japanese populations, from which the two most famous medaka inbred lines (Hd-rR and HNI) are derived, were regarded as a single species: Oryzias latipes (Figure 1C). Yet accumulating evidence suggested that these lines represent different species. Hence, the northern Japanese population was described as a new species: Oryzias sakaizumii by Asai et al., 2011, and medaka is now frequently referred to as “Oryzias latipes species complex” (Iguchi et al., 2018; Kawajiri et al., 2015; Takehana et al., 2016). Depending on the method, Oryzias latipes and Oryzias sakaizumii were estimated to have diverged between 3 and 8 million years ago (Figure 2A, Katsumura et al., 2019; Takehana et al., 2003; but see Setiamarga et al., 2009). They differ in a number of traits including craniofacial anatomy (Kimura et al., 2007), body coloration (Asai et al., 2011), aggressiveness (Kagawa, 2014) and the degree of sexual dimorphism of the dorsal fin (Asai et al., 2011), the last of which is likely mediated by different sex steroid levels during development (Katsumura et al., 2014; Kawajiri et al., 2015). Nonetheless, both species produce viable hybrid offspring in the laboratory (e.g. Murata et al., 2012) and current evidence also suggests limited gene flow in the wild (Iguchi et al., 2018; Katsumura et al., 2019). Oryzias latipes and O. sakaizumii thus offer the opportunity to gain insight into the evolution of physiological and behavioral trait disparity and their genetic bases. Recent insight into the biogeography of the medaka also suggests that O. latipes colonized the main island of Japan from the south (i.e., from Kyushu) and subsequently dispersed along the Pacific coast (Katsumura et al., 2019). In contrast, Oryzias sakaizumii likely originated on the western side of the Japanese Alps, which still largely separate both species in their current distribution (Figure 2A, Katsumura et al., 2019).
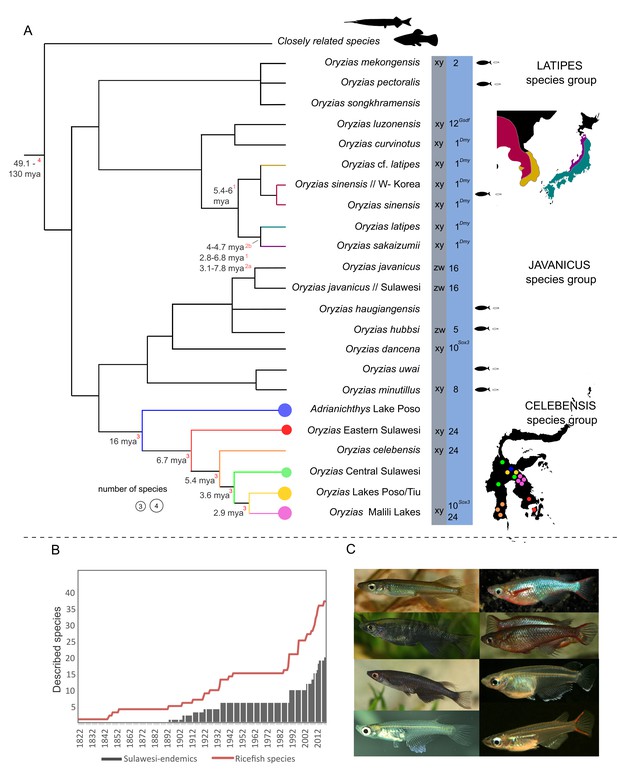
Ricefish diversity.
(A) Combined phylogeny based on published studies from: Katsumura et al., 2019, Parenti, 2008; Magtoon, 2010; Mokodongan and Yamahira, 2015; Takehana et al., 2005. Sex determination systems and linkage groups with sex determination (SD) genes are given in the gray and blue columns, respectively (based on Matsuda and Sakaizumi, 2016 and Myosho et al., 2015). Species defined as miniaturized (following Parenti, 2008) are marked with black/white fish. Detailed distribution maps are provided for the O. latipes species complex and for the celebensis species group. Divergence times in the tree are based on: 1Takehana et al., 2003; 2aKatsumura et al., 2019: scenario III; 2bKatsumura et al., 2019: scenario IV; 3Mokodongan and Yamahira, 2015; 4Hughes et al., 2018. (B) Total number of described species from the first description of a ricefish in 1822 (Hamilton, 1822) until today (red line) in relation to described Sulawesi endemics (gray bars). The high number of newly described species in recent years especially on Sulawesi points towards a largely underexplored ricefish diversity. (C) Diversity in coloration and shapes of male Oryzias. From left to right and top to bottom: O. sarasinorum*, O. woworae§, O. eversi*, O. wolasi*, O. nigrimas*, O. dancena§, O. minutillus§, O. mekongensis§ (Photos were taken by A Wagnitz* and J Geck§).
The Korean medaka lineages are genetically divergent from the Japanese species and also highly structured (Figures 1C and 2A). They comprise at least two subgroups that have been referred to as the East Korean (HSOK strain) and China–West Korean populations (Ds et al., 2013; Kang et al., 2005; Katsumura et al., 2019; Parenti, 2008). Although still frequently referred to as O. latipes (see Katsumura et al., 2019; Spivakov et al., 2014; Takeda and Shimada, 2010), the China–West Korean population together with populations from Taiwan, likely represents a different species: Oryzias sinensis (see Ds et al., 2013; Parenti, 2008; Tzeng et al., 2006). While further data are required, mitochondrial phylogenies indicate the existence of a locally restricted ancient Taiwanese medaka lineage and two lineages derived from more recent invasion events (Tzeng et al., 2006). Continental O. sinensis are also genetically diverse and geographically distant populations exhibit signatures of divergence, for example, between Shanghai and Korea (Katsumura et al., 2019). However, comprehensive studies covering the morphological and genetic diversity in continental Asia are still lacking. Such studies will be quintessential to recognize species limits and distribution patterns in the future. Generally, whether or not divergent lineages within the O. latipes species complex represent different species is still treated controversially and inconsistently in the current literature (see Katsumura et al., 2019; Parenti, 2008; Sasaki and Yamahira, 2016; Spivakov et al., 2014; Tzeng et al., 2006). Aside from the underlying taxonomic debate, information about the degree of divergence and abundance of gene flow between lineages is fundamental for the design of experiments, as well as the interpretation and integration of their results.
Latitudinal adaptation in Japanese medaka species
The wide range of Japanese medaka species has inspired research on latitudinal adaptation. For example, a recent study identified differences in courtship between populations of O. sakaizumii, which were inferred to result from stronger sexual selection at lower latitudes (Sasaki and Yamahira, 2016). Additionally, juveniles of O. sakaizumii from higher latitudes compensate for a shorter growing season further north with faster growth rates (Yamahira and Takeshi, 2008). However, faster growth, which is achieved by higher feeding rates, comes with a cost and is accompanied by higher vulnerability for predation by dragonfly larvae (Suzuki et al., 2010). Hence, different growth capacities in O. sakaizumii likely evolve in response to both predation pressure and length of the growing season (Suzuki et al., 2010). One study also found delayed fin development in northern populations of O. sakaizumii compared to the southernmost populations of O. latipes, which was speculated to have evolved in a trade-off for faster body growth (Kawajiri et al., 2009). However, this study illustrates the caveats related to taxonomic uncertainties, because the authors unintentionally compared populations of different species and thus did not take other interspecific differences into consideration. Nonetheless, their interpretation might be accurate and interspecific diversity clearly represents a chance to gain insight into its evolution, if species boundaries are recognized.
The diversity of medaka’s wild relatives
Ricefish phylogeny
Although mainly recognized for medaka, ricefishes comprise a considerable diversity with 36 described species in two genera (Oryzias: 32 species, Adrianichthys: four species, Asai et al., 2011; Herder et al., 2012a; Herder and Chapuis, 2010; Magtoon, 2010; Mandagi et al., 2018; Mokodongan et al., 2014; Parenti, 2008; Parenti et al., 2013; Parenti and Hadiaty, 2010). They form three major clades referred to as the latipes-, javanicus- and celebensis species group, the last of which diversified on Sulawesi, Indonesia (Figure 2A, Mokodongan and Yamahira, 2015; Takehana et al., 2005). With a total of 21 species and 19 local endemics, this island represents a hotspot of ricefish diversity (Figures 1C and 2A–B). Additionally, nine newly described species within the last decade, eight of them endemic to Sulawesi, suggest that both the global ricefish biodiversity as well as the ricefish fauna of Sulawesi are still underdescribed (Figure 2A). Nonetheless, the ricefishes known to science exhibit striking functional diversity, with relatively little of it understood or even investigated so far. In the following paragraphs we highlight research making use of ricefish diversity together with aspects that appear especially promising for future research endeavors.
Sex determination
Sex determination mechanisms are highly variable in bony fishes (Heule et al., 2014) and genetic sex determination (SD) appears to be particularly diverse in ricefishes (Kondo et al., 2009; Matsuda and Sakaizumi, 2016; Myosho et al., 2015; Takehana et al., 2007a; Takehana et al., 2007b). So far, seven sex chromosomes and three different master SD genes have been identified in ricefish species (Figure 2A). For example, O. latipes has a young XY system in which dmrt1bY (dmy) on linkage group 1 (LG1) acts as the male SD gene (Matsuda, 2005; Matsuda et al., 2002). In contrast, gsdf on LG12 is the SD gene in O. luzonensis (Myosho et al., 2012), and sox3 on LG10 serves as SD gene in O. dancena, O. marmoratus and O. profundicola (Figure 2A, Myosho et al., 2015; Takehana et al., 2014). Oryzias hubbsi and O. javanicus even exhibit a ZW, i.e. female heterogametic, system, albeit with different sex chromosomes (Takehana et al., 2007b). This astonishing diversity of young sex determination systems inspired ongoing research on the molecular mechanisms underlying rapid sex chromosome turnover and the rewiring of gene regulatory networks that is required to establish new master SD genes (Herpin et al., 2010; Matsuda and Sakaizumi, 2016).
Salinity tolerance
Ricefishes live in hyper- and hypoosmotic environments and exhibit different levels of salinity tolerance (Hayakawa et al., 2015; Inoue and Takei, 2003; Inoue and Takei, 2002). While most Oryzias are primarily found in freshwater, O. javanicus and O. dancena (also referred to as O. melastigma, likely a synonym; Parenti, 2008; Roberts, 1998) occur in saltwater and brackish water, respectively. These species are being established as models for marine ecotoxicology to complement the medaka’s role in freshwater (Chen et al., 2011; Dong et al., 2014; Kang et al., 2013; Kim et al., 2018; Koyama et al., 2008). Mobility between freshwater and saltwater depends on an organism’s ability to adjust to the osmotic differences. Interestingly, Oryzias latipes can better adjust to saltwater when exposed to elevated levels of salinity earlier in life (Miyanishi et al., 2016). In contrast, species from some remote freshwater systems in Indonesia do not tolerate even small changes in osmolarity (Inoue and Takei, 2002; Myosho et al., 2018). The ability of O. latipes to acclimate to seawater was used to study the physiological and genetic basis of salt-water tolerance. Salinity tolerance in Oryzias is based on both genetic (Myosho et al., 2018, Ogoshi et al., 2015) and epigenetic factors, including an increase in the density of osmoregulating cells called ionocytes (Liu et al., 2016; Miyanishi et al., 2016) and an increase in activity of the same cells (Kang et al., 2008).
Sulawesi: A natural laboratory of ricefish diversity
In addition to their species richness, Sulawesi ricefishes stand out due to their diversity in several traits (Kottelat, 1990; Myosho et al., 2018; Myosho et al., 2015; Parenti, 2008). While most ricefishes are largely pale in color, Sulawesi ricefishes exhibit several different fin and nuptial colorations and males in the O. woworae complex evolved an outstanding iridescent blue ornamentation (Figure 2C, Parenti et al., 2013). This diversity allows us to expand on the tremendous knowledge of medaka color mutants and to investigate, for example, its genetic basis and the evolutionary role of coloration disparity in ricefishes. Ricefishes further have a wide range of body shapes and sizes (Parenti, 2008). Within the O. woworae species complex there is variation in body depth (Parenti et al., 2013), which likely evolved in response to different flow regimes (Mokodongan et al., 2018) and raises the question of interspecific diversity and plasticity of this trait. The largest ricefish A. poptae from the ancient Lake Poso reaches more than ten times the size of miniature species such as O. mekongensis (see Parenti, 2008). Investigating the evolutionary mechanisms that gave rise to the sympatric ricefish species flocks from Lake Poso (namely Oryzias orthognathus, O. nigrimas, O. nebulosus and Adrianichthys oophorus, A. kruyti, A. roseni, A. poptae) appears particularly intriguing (Mokodongan and Yamahira, 2015). Virtually nothing is known about the ecology and evolution of these species, but striking differences in their craniofacial anatomy point at specialization in their feeding habits as a potential driver of lineage diversification (Parenti, 2008; Parenti and Soeroto, 2004). Finally, a new reproductive strategy referred to as “pelvic brooding” has evolved in at least two lineages from Sulawesi (Herder et al., 2012a; Kottelat, 1990). Pelvic brooders do not deposit fertilized eggs, but instead carry their clutch until the fry hatches (Box 2, Iwamatsu, 2004; Iwamatsu et al., 2008; Parenti, 2008). While this innovation likely relies on a complex set of behavioral, physiological and anatomical adaptations, the adaptive benefit of this female investment remains unexplored. The potentially repeated and likely relatively recent evolution of pelvic brooding in combination with the available resources for medaka make it a promising system to gain insight into the molecular basis of evolutionary innovations, which is a central goal of evolutionary biology (Erwin, 2015; Shubin et al., 2009; Wagner and Lynch, 2010).
Box 2.
Pelvic brooding – a complex innovation.
Some ricefishes from Sulawesi exhibit a unique reproductive strategy referred to as “pelvic brooding” (Kottelat, 1990). While most ricefishes deposit fertilized eggs shortly after spawning, pelvic brooders instead carry an egg-clutch in a ventral concavity until the fry hatches (Box 2—figure 1). Pelvic brooding likely relies on a complex set of adaptations (Iwamatsu et al., 2007; Iwamatsu et al., 2008; Kottelat, 1990; Parenti, 2008). Elongated pelvic fins cover the eggs, while a plug structure that is connected to the eggs via attaching filaments anchors them inside the female’s ovarian cavity. Ovulation is delayed in pelvic brooders for as long as eggs are attached, and the plug was even assumed to allow the transfer of nutrients, akin to a placenta (Iwamatsu et al., 2008). Despite this complexity, pelvic brooding occurs in at least three species from two lineages, namely Adrianichthys oophorus, Oryzias eversi and Oryzias sarasinorum. This means it was either lost multiple times or evolved twice independently (Mandagi et al., 2018; Mokodongan and Yamahira, 2015). Pelvic brooding was suggested to have evolved in adaptation to the absence of suitable spawning substrates in pelagic habitats (Herder et al., 2012a). The recent discovery of the oviparous O. dopingdopingensis by Mandagi et al. (2018), a sister species of the pelvic brooding O. sarasinorum and O. eversi, in a river in central Sulawesi might be considered as support for this hypothesis. However, the pelvic brooding O. eversi was described from a spring where potential spawning substrates are abundant (Herder et al., 2012a). Consequently, the eco-evolutionary dynamics promoting pelvic brooding as well as the underlying molecular mechanisms remain virtually unknown.
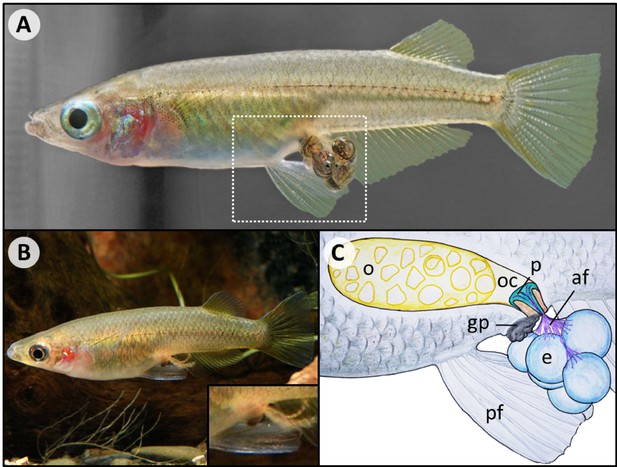
Overview of pelvic brooding.
(A) The pelvic brooding ricefish O. eversi carries its eggs until the fry hatches (dashed box indicates section depicted in (C)). (B) Once the fry has hatched, only the filaments remain attached to the female (Photo by Hans-Georg Evers). (C) A schematic overview of anatomic structures that likely play a role in pelvic brooding. The eggs (e) stay attached to the female via attaching filaments (af). A plug (p) anchors the attaching filaments (af) inside the female’s ovarian cavity (oc) next to the ovary (o). Compared to their non-pelvic brooding relatives, pelvic brooding species further appear to have elongated pelvic fins (pf) that cover the eggs and a heavily pigmented, enlarged genital papilla (gp), whose role in pelvic brooding is unclear.
New opportunities from technological advances
Technological progress continues to open up new opportunities to study the molecular basis of the natural diversity of ricefishes. Genome evolution can now be studied using long sequencing reads (Eid et al., 2009; Jain et al., 2018; Vaser et al., 2017) to shed light on the contribution of structural variants (see Sedlazeck et al., 2018) and methylation patterns (Simpson et al., 2017) to adaptation and speciation. The development of single cell transcriptome sequencing also enables insight into cell-specific gene expression patterns (Andrews and Hemberg, 2018; Fiers et al., 2018; Tang et al., 2010). Single cell transcriptome sequencing can, for example, be used to study the evolution of gene regulatory networks underlying sex determination. All of these technologies can help to identify candidate loci that may contribute to evolutionary processes of interest, while the phenotypic effects of natural genetic variation at these loci can now be assessed using gene-editing tools (Gutierrez-Triana et al., 2018; Letelier et al., 2018; Murakami et al., 2017; Watakabe et al., 2018). Together these technological advances have set the stage for scientists to begin converting the described natural diversity of ricefishes into a better understanding of the molecular basis of evolutionary processes.
Threatened diversity
The world’s freshwater biota is facing a biodiversity crisis and ricefishes are no exception. Only seven out of 21 assessed ricefish species are classified as least concerned according to the IUCN Red List. However, much of the currently available data needs to be treated with caution because the majority of species assessments based on sufficient data were carried out more than two decades ago (IUCN, 2019). What is known is that the high endemism among Sulawesi ricefishes, which are threatened by invasive species, habitat destruction, intensive fishing and pollution, makes them particularly vulnerable for extinction (Herder et al., 2012b; Hilgers et al., 2018; Mokodongan et al., 2014; Parenti, 2008). Accordingly, the Lake Poso endemics A. roseni and A. kuyti, which have not been caught since 1978 and 1983, respectively, are either on the brink of extinction or have already gone extinct (Kottelat, 1990). However, severe threats are not restricted to medaka’s relatives from Sulawesi. Populations of both Japanese ricefish species have reportedly been declining, particularly in densely populated regions and where exotic species have been introduced (Asai et al., 2011; Mamun et al., 2016; Parenti, 2008; Parenti and Soeroto, 2004). Ricefishes were even thought to have gone extinct in Taiwan, before they were rediscovered in 1993 (Parenti, 2008; Tzeng et al., 2006). In conclusion, current threats and their potential effect often remain speculative. Thus, new species assessments and conservation actions are urgently needed, and ultimately their success will also rely on a better understanding of medaka’s life in the wild.
Conclusions
Despite recent progress, there is much more we can learn about the natural history of medaka. The taxonomic debate around medaka that is fueled by recent insights clearly calls for further investigations, particularly targeting continental medaka species. Combining the tools and resources available for medaka with the diversity of its wild relatives harbors tremendous potential for evolutionary biology. In turn, putting our knowledge on medaka into an evolutionary context may inspire and inform further research in the fields that have traditionally used medaka as a model organism. Sulawesi appears to be an obvious focal point for studying the evolution of ricefish diversity. Conservation efforts targeting some of the ricefish species that are most interesting to science and which appear to be on the brink of extinction are urgently needed. Finally, exciting new research opportunities on medaka arise from the recent establishment of new gene-editing tools and the first isogenic population genetic panel for a vertebrate, which will allow interactions between genotype and environment to be dissected (Spivakov et al., 2014).
References
-
On the inheritance of color in a fresh-water fish, Aplocheilus latipes Temmick and Schlegel, with special reference to sex-linked inheritanceGenetics 6:554–573.
-
Identifying cell populations with scRNASeqMolecular Aspects of Medicine 59:114–122.https://doi.org/10.1016/j.mam.2017.07.002
-
Oryzias sakaizumii, a new ricefish from northern japan (Teleostei: Adrianichthyidae)Ichthyol Explor Freshwaters 22:289–299.
-
A new model army: emerging fish models to study the genomics of vertebrate Evo-DevoJournal of Experimental Zoology Part B: Molecular and Developmental Evolution 324:316–341.https://doi.org/10.1002/jez.b.22589
-
Molecular staging of marine medaka: a model organism for marine ecotoxicity studyMarine Pollution Bulletin 63:309–317.https://doi.org/10.1016/j.marpolbul.2011.03.042
-
Cytochrome oxidase subunit I (COI) DNA sequence divergence between tow cryptic species of Oryzias in South KoreaJournal of Ecology and Environment 36:159–166.https://doi.org/10.5141/ecoenv.2013.159
-
Empirical evidence for competition-driven semelparity in wild medakaPopulation Ecology 58:371–383.https://doi.org/10.1007/s10144-016-0551-4
-
Life span data for the small fish, Oryzias latipesExperimental Gerontology 4:127–129.https://doi.org/10.1016/0531-5565(69)90035-7
-
Novelty and innovation in the history of lifeCurrent Biology 25:R930–R940.https://doi.org/10.1016/j.cub.2015.08.019
-
Mapping gene regulatory networks from single-cell omics dataBriefings in Functional Genomics 17:246–254.https://doi.org/10.1093/bfgp/elx046
-
An adaptive modeling technique for instream fish habitat preference of japanese medaka (Oryzias latipes)Faculty of Agriculture Kyushu University 50:363–373.
-
BookAn Account of the Fishes Found in the River Ganges and Its BranchesEdinburgh: A. Constable and company.
-
Pelvic-fin brooding in a new species of riverine ricefish (Atherinomorpha: Beloniformes: Adrianichthyidae) from Tana Toraja, Central Sulawesi, IndonesiaThe Raffles Bulletin of Zoology 60:467–476.
-
Oryzias hadiatyae, a new species of ricefish (Atherinomorpha: beloniformes: adrianichthyidae) endemic to Lake Masapi, Central Sulawesi, IndonesiaThe Raffles Bulletin of Zoology 58:269–280.
-
Alien attack: trophic interactions of flowerhorn cichlids with endemics of ancient Lake Matano (Sulawesi, Indonesia)Evolutionary Ecology Research 19:575–590.
-
BookA Glance at the Past of Medaka Fish BiologyIn: Naruse K, Tanaka M, Takeda H, editors. Medaka - a Model for Organogenesis, Human Disease, and Evolution. Tokyo: Japan: Springer. pp. 1–18.https://doi.org/10.1007/978-4-431-92691-7_1
-
Diverse adaptability in oryzias species to high environmental salinityZoological Science 19:727–734.https://doi.org/10.2108/zsj.19.727
-
Asian medaka fishes offer new models for studying mechanisms of seawater adaptationComparative Biochemistry and Physiology Part B: Biochemistry and Molecular Biology 136:635–645.https://doi.org/10.1016/S1096-4959(03)00204-5
-
Stages of normal development in the medaka Oryzias latipesMechanisms of Development 121:605–618.https://doi.org/10.1016/j.mod.2004.03.012
-
BookThe Integrated Book for the Biology of the Medaka (2nd)Tokyo, Japan: Daigaku Kyoiku.
-
Oviposition cycle in the oviparous fish Xenopoecilus sarasinorumZoological Science 24:1122–1127.https://doi.org/10.2108/zsj.24.1122
-
Nanopore sequencing and assembly of a human genome with ultra-long readsNature Biotechnology 36:338–345.https://doi.org/10.1038/nbt.4060
-
A review of the poeciliidae or killifishes of japanProceedings of the United States National Museum 31:287–290.https://doi.org/10.5479/si.00963801.31-1486.287
-
Molecular phylogeny and geography of korean medaka fish (Oryzias latipes)Molecules and Cells 20:151–156.
-
Differential expression of branchial na+/K+-ATPase of two medaka species, Oryzias latipes and Oryzias dancena, with different salinity tolerances acclimated to fresh water, brackish water and seawaterComparative Biochemistry and Physiology Part A: Molecular & Integrative Physiology 151:566–575.https://doi.org/10.1016/j.cbpa.2008.07.020
-
The acute and regulatory phases of time-course changes in gill mitochondrion-rich cells of seawater-acclimated medaka (Oryzias dancena) when exposed to hypoosmotic environmentsComparative Biochemistry and Physiology Part A: Molecular & Integrative Physiology 164:181–191.https://doi.org/10.1016/j.cbpa.2012.08.010
-
Natural allelic variations of xenobiotic-metabolizing enzymes affect sexual dimorphism in Oryzias latipesProceedings of the Royal Society B: Biological Sciences 281:20142259.https://doi.org/10.1098/rspb.2014.2259
-
Medaka population genome structure and demographic history described via genotyping-by-sequencingG3: Genes|Genomes|Genetics 9:217–228.https://doi.org/10.1534/g3.118.200779
-
Heterochronic differences in fin development between latitudinal populations of the medaka Oryzias latipes (Actinopterygii: Adrianichthyidae)Biological Journal of the Linnean Society 97:571–580.https://doi.org/10.1111/j.1095-8312.2009.01233.x
-
The genome of the marine medaka Oryzias melastigmaMolecular Ecology Resources 18:656–665.https://doi.org/10.1111/1755-0998.12769
-
Insertion of a novel transposable element in the tyrosinase gene is responsible for an albino mutation in the medaka fish, Oryzias latipesMGG Molecular & General Genetics 249:400–405.https://doi.org/10.1007/BF00287101
-
Medaka: A Model for Organogenesis, Human Disease, and EvolutionTransposable Elements Tol1 and Tol2, Medaka: A Model for Organogenesis, Human Disease, and Evolution, Tokyo: Japan, Springer.
-
Sex determination and sex chromosome evolution: insights from medakaSexual Development 3:88–98.https://doi.org/10.1159/000223074
-
Synopsis of the endangered buntingi (Osteichthyes: adranichtyidae and oryziidae) of Lake Poso, Central Sulawesi, Indonesia, with a new reproductive guild and descriptions of three new speciesIchthyol Explor Freshwaters 1:46–67.
-
Java medaka: a proposed new marine test fish for ecotoxicologyEnvironmental Toxicology 23:487–491.https://doi.org/10.1002/tox.20367
-
Oryzias songkhramensis, A new species of ricefish (Beloniformes; Adrianichthyidae) from northeast Thailand and central laosTropical Natural History 10:107–129.
-
Medaka in japanese agricultural water channels: genetic diversity and conservationReviews in Agricultural Science 4:8–20.https://doi.org/10.7831/ras.4.8
-
Sex determination in the teleost medaka, Oryzias latipesAnnual Review of Genetics 39:293–307.https://doi.org/10.1146/annurev.genet.39.110304.095800
-
Evolution of the sex-determining gene in the teleostean genus OryziasGeneral and Comparative Endocrinology 239:80–88.https://doi.org/10.1016/j.ygcen.2015.10.004
-
Phylogenomics reveals habitat-associated body shape divergence in Oryzias woworae species group (Teleostei: Adrianichthyidae)Molecular Phylogenetics and Evolution 118:194–203.https://doi.org/10.1016/j.ympev.2017.10.005
-
Origin and intra-island diversification of Sulawesi endemic AdrianichthyidaeMolecular Phylogenetics and Evolution 93:150–160.https://doi.org/10.1016/j.ympev.2015.07.024
-
Turnover of sex chromosomes in Celebensis group medaka fishesG3: Genes|Genomes|Genetics 5:2685–2691.https://doi.org/10.1534/g3.115.021543
-
A synopsis of the parasites of medaka (Oryzias latipes) of Japan (1929-2017)Biosph Sci 56:71–85.
-
Mating ethogram in Oryzias latipes (With 1 Text-figure)J Fac Sci Hokkaido Univ Ser VI Zool 13:197–202.
-
BookThe phylogeny of atherinomorphs: Evolution of a novel fish reproductive systemIn: Uribe M. C, Grier H. J, editors. Viviparous Fishes. New Life Publications, FL. pp. 13–30.
-
A phylogenetic analysis and taxonomic revision of ricefishes, Oryzias and relatives (Beloniformes, Adrianichthyidae)Zoological Journal of the Linnean Society 154:494–610.https://doi.org/10.1111/j.1096-3642.2008.00417.x
-
Rigid isolation between the northern population and the southern population of the medaka, Oryzias latipes (Genetics)Zoological Science 1:795–800.
-
Variation in male courtship activeness between latitudinal populations of northern medakaIchthyological Research 63:302–306.https://doi.org/10.1007/s10228-015-0484-5
-
BookXiphophorus and medaka cancer modelsIn: Langenau D. M, editors. Cancer and Zebrafish, Advances in Experimental Medicine and Biology. Cham: Springer International Publishing. pp. 531–552.https://doi.org/10.1007/978-3-319-30654-4_23
-
Medaka as a research organism: past, present and futureMechanisms of Development 121:599–604.https://doi.org/10.1016/j.mod.2004.03.011
-
Detecting DNA cytosine methylation using nanopore sequencingNature Methods 14:407–410.https://doi.org/10.1038/nmeth.4184
-
The art of medaka genetics and genomics: what makes them so unique?Annual Review of Genetics 44:217–241.https://doi.org/10.1146/annurev-genet-051710-151001
-
Molecular phylogeny of the medaka fishes genus Oryzias (Beloniformes: Adrianichthyidae) based on nuclear and mitochondrial DNA sequencesMolecular Phylogenetics and Evolution 36:417–428.https://doi.org/10.1016/j.ympev.2005.01.016
-
Origin of boundary populations in medaka (Oryzias latipes species complex)Zoological Science 33:125–131.https://doi.org/10.2108/zs150144
-
The phylogeography and population demographics of selected freshwater fishes in TaiwanZoological Studies 45:285–297.
-
Circadian oviposition rhythm and locomotor activity in the medaka, Oryzias latipesJournal of Interdisciplinary Cycle Research 13:97–104.https://doi.org/10.1080/09291018209359769
-
Karyotypes and geographical distribution of ricefishes from Yunnan, southwestern ChinaJapanese J Ichthyol 35:332–340.
-
Fast and accurate de novo genome assembly from long uncorrected readsGenome Research 27:737–746.https://doi.org/10.1101/gr.214270.116
-
Medaka--a model organism from the far eastNature Reviews Genetics 3:53–64.https://doi.org/10.1038/nrg704
-
Artificial induction of functional sex-reversal in genotypic females of the medaka (Oryzias latipes)Journal of Experimental Zoology 137:227–263.https://doi.org/10.1002/jez.1401370203
Decision letter
-
Stuart RF KingReviewing Editor; eLife, United Kingdom
-
Peter RodgersSenior Editor; eLife, United Kingdom
In the interests of transparency, eLife includes the editorial decision letter and accompanying author responses. A lightly edited version of the letter sent to the authors after peer review is shown, indicating the most substantive concerns; minor comments are not usually included.
Thank you for submitting your article "The Natural History of Model Organisms: The untapped potential of medaka's wild relatives" for consideration by eLife. Your article has been reviewed by three peer reviewers, and the evaluation has been overseen by two Features Editors at eLife (Stuart King and Peter Rodgers). The following individuals involved in review of your submission have also agreed to reveal their identity: Kiyoshi Naruse (Reviewer #2); Lynne Parenti (Reviewer #3).
After a consultation with the reviewers, the editor has drafted this decision to help you prepare a revised submission.
Summary:
This essay is being considered as part of a series of articles on "The Natural History of Model Organisms" (https://elifesciences.org/collections/8de90445/the-natural-history-of-model-organisms). Each article should explain how our knowledge of the natural history of a model organism has informed recent advances in biology, and how understanding its natural history can influence/advance future studies.
This article on the "untapped potential of medaka's wild relatives" sheds interesting light on under-explored resources around the long-established genetic model system: the Japanese ricefish or medaka. The authors swiftly review the history and advantages of medaka as a model system before highlighting the possible application of medaka and its relatives for the context of evolutionary biology. The editors and reviewers share the authors' conviction that this article will interest a wide audience that includes scientists working with medaka in the lab, and evolutionary biologists with a focus on other natural model systems. However, a number of details should be attended to prior to publication.
Essential revisions:
1) The reviewers believe that the revised article could do more to acknowledge prior work involving medaka. In particular, Japanese biologists have been comparing their native ricefish species to those found throughout Asia for decades and, as a result, more is known about Oryzias latipes and its close relatives than about many other species of fishes. For example, Takashi Iwamatsu's multiple editions of The Integrated Book for the Biology of the Medaka (most recent edition 2018) are remarkably detailed and comparative texts available in Japanese. The revision should avoid any phrases that could be misinterpreted as a dismissal of this knowledge (e.g. "surprisingly little is known about the natural history of medaka").
2) To further highlight the potential of this model system, reviewer #1 has suggested adding an additional paragraph that discusses how new technical advances could help to convert the described diversity in the natural resources into a molecular understanding of evolutionary processes, such as adaptation and speciation.
3) The article uses the word "medaka" in several ways: once to mean Oryzias latipes (Introduction, second paragraph); a second time to mean O. latipes and O. sakaizumii (publications on the Japanese Medaka before 2011 referred to general characteristics of both of these species unless the particular population was specified); and a third to mean the O. latipes complex (Introduction, first paragraph and subsection “Medaka’s life in the wild”). Further, the article also uses "medaka" as a collective and a singular noun. Though this is a common problem when the meaning of a word is changed, it may confuse readers. All of these species have unique common names, so please revise the article with this in mind. Reviewer #3 suggested adding a table of scientific names along with those common names to help readers sort through this information.
4) Reviewer #2 felt that, while they are described as Oryzias latipes from the Aichi Prefecture (Aichi-ken), the fish depicted in Figure 1A-B look more like an orange-red variety rather than the wild type brown. Please consider changing the figure legend to acknowledge this, or finding new photos that more clearly show the brown coloration of wild type medaka.
5) Reviewer #2 also thought that the distribution of the ricefish family (Adrianichthyidae) in Figure 1D was potentially misleading. To our knowledge, there is no information about distribution of these fish inside the Asian continent, especially in the Himalayan area. We are also unaware of any information about the Oryzias latipes species complex around Hong Kong (subsection “Medaka’s life in the wild”, first paragraph). The revised article should provide additional references to support these claims, or revise the figures and the text to match the distributions described in previous studies.
6) Box 2's discussion of the pelvic brooding could benefit from the inclusion of the recently described species, Oryzias dopingdopingensis [Mandagi et al., 2018]. This species is a sister species of O. eversi and O. sarasinorum, both of which live in lake habitats and have pelvic fin brooding system. O. dopingdopingensis, on the other hand, lives in a river system and deposits its fertilized eggs on substrates around its habitat. The reviewers feel that these details are important in the context of the evolutionary scenario of the pelvic fin brooding system in Oryzias in Sulawesi.
https://doi.org/10.7554/eLife.46994.008Author response
Essential revisions:
1) The reviewers believe that the revised article could do more to acknowledge prior work involving medaka. In particular, Japanese biologists have been comparing their native ricefish species to those found throughout Asia for decades and, as a result, more is known about Oryzias latipes and its close relatives than about many other species of fishes. For example, Takashi Iwamatsu's multiple editions of The Integrated Book for the Biology of the Medaka (most recent edition 2018) are remarkably detailed and comparative texts available in Japanese. The revision should avoid any phrases that could be misinterpreted as a dismissal of this knowledge (e.g. "surprisingly little is known about the natural history of medaka").
We thank the reviewers for raising our awareness for this issue. To avoid statements that could be misinterpreted as dismissal of knowledge based on prior work, we followed the reviewers’ advice and now reference Iwamatsu’s “The integrated book for the biology of the medaka” and added all further citations mentioned by the reviewers. As suggested by reviewer #3 we further changed: "surprisingly little is known about the natural history of medaka" to “there is much more we can learn about the natural history of medaka”.
Additionally: “Overall, very little of our current knowledge on the biology of medaka has been gathered from studies in the wild.” has been modified to “Overall, relatively little of our current knowledge on the biology of medaka has been gathered from studies in the wild.”
Finally, to further acknowledge previous achievements, yet still underline the contrast between knowledge on the model system and the natural history of ricefishes, we modified the following section, which now reads:
“This is despite the fact that particularly Japanese biologists have been working on ricefishes for decades, which generated a more complete knowledge of O. latipes and its relatives than is available for many other fish species (Iwamatsu, 2006). However, some of this work is not available in English and thus remains hardly accessible for the international research community. Furthermore, studies have highlighted a so far underexplored ricefish diversity in the wild (Katsumura et al., 2018; Parenti, 2008; Takehana et al., 2005; Uwa et al., 1988), which has already inspired novel research agendas (e.g., Matsuda and Sakaizumi, 2016; Mokodongan et al., 2018; Spivakov et al., 2014).”
2) To further highlight the potential of this model system, reviewer #1 has suggested adding an additional paragraph that discusses how new technical advances could help to convert the described diversity in the natural resources into a molecular understanding of evolutionary processes, such as adaptation and speciation.
We are grateful for the suggestion of reviewer #1 to add an additional paragraph that discusses how recent technical advances can be used to generate a better understanding of the molecular processes that gave rise to the previously discussed natural diversity of ricefishes. We agree that this may be an interesting addition and thus included a short paragraph that discusses recent advances together with some of the benefits that may be employed to shed light onto the evolution of ricefish diversity.
“New opportunities: Technological advances
Technological progress continues to open up new opportunities to study the molecular basis of the natural ricefish diversity. Genome evolution can now be studied using long sequencing reads (Eid et al., 2009; Jain et al., 2018; Vaser et al., 2017) to shed light on the contribution of structural variants (see Sedlazeck et al., 2018) and methylation patterns (Simpson et al., 2017) to adaptation and speciation in ricefishes. Additionally, the development of single cell transcriptome sequencing enables insight into cell-specific gene expression patterns (Andrews and Hemberg, 2018; Fiers et al., 2018; Tang et al., 2010). Single cell transcriptome sequencing can for example be employed to study the evolution of gene regulatory networks underlying sex determination. All of these technologies can help to identify candidate loci that potentially contribute to evolutionary processes of interest. Intriguingly, phenotypic effects of natural genetic variation at these loci can now be assessed using the gene editing tool CRISPR/Cas9 (Gutierrez-Triana et al., 2018; Letelier et al., 2018; Murakami et al., 2017; Watakabe et al., 2018). Consequently, recent technological advances will facilitate converting the described natural ricefish diversity into a better understanding of the molecular basis of evolutionary processes.
3) The article uses the word "medaka" in several ways: once to mean Oryzias latipes (Introduction, second paragraph); a second time to mean O. latipes and O. sakaizumii (publications on the Japanese Medaka before 2011 referred to general characteristics of both of these species unless the particular population was specified); and a third to mean the O. latipes complex (Introduction, first paragraph and subsection “Medaka’s life in the wild”). Further, the article also uses "medaka" as a collective and a singular noun. Though this is a common problem when the meaning of a word is changed, it may confuse readers. All of these species have unique common names, so please revise the article with this in mind. Reviewer #3 suggested adding a table of scientific names along with those common names to help readers sort through this information.
We thank the reviewers for pointing out ambiguity and inconsistency in the nomenclature linked to medaka. We agree that this may cause confusion and have revised the manuscript accordingly. Hence, medaka is only used as singular noun in the revised manuscript. Further, we only use the term “medaka” to collectively refer to lineages within the Oryzias latipes species complex in the context of the model system. We stick to common or scientific species names when we specifically refer to individual lineages. Furthermore, to help readers sort through this information, we added Table 1 – Medaka nomenclature, which explains our use of medaka within this manuscript. Additionally, to assist the reader, we follow the recommendation of reviewer #3 and added Table 1 with common and scientific names of species in the Oryzias latipes species complex.
The explanatory text in Table 1 now reads:
“The model organism medaka comprises several species and deeply divergent lineages from the Oryzias latipes species complex. Hence, in this article we only use the term “medaka” when we collectively refer to lineages within the Oryzias latipes species complex in the context of the model system. Common or scientific species names are used to specifically refer to individual lineages as shown in Table 1.”
4) Reviewer #2 felt that, while they are described as Oryzias latipes from the Aichi Prefecture (Aichi-ken), the fish depicted in Figure 1A-B look more like an orange-red variety rather than the wild type brown. Please consider changing the figure legend to acknowledge this, or finding new photos that more clearly show the brown coloration of wild type medaka.
We followed reviewer #2’s suggestion and chose a different photograph for which no doubt exists with respect to type or locality. The figure and figure legend have been modified accordingly.
5) Reviewer #2 also thought that the distribution of the ricefish family (Adrianichthyidae) in Figure 1D was potentially misleading. To our knowledge, there is no information about distribution of these fish inside the Asian continent, especially in the Himalayan area. We are also unaware of any information about the Oryzias latipes species complex around Hong Kong (subsection “Medaka’s life in the wild”, first paragraph). The revised article should provide additional references to support these claims, or revise the figures and the text to match the distributions described in previous studies.
Although we agree with reviewer #2 in that little is known about the continental distribution of Adrianichthyidae, we prepared the figure in accordance with a figure published in the most comprehensive work, i.e. the revision of Adrianichthyidae by Parenti, 2008. Within this study it is also stated that medaka is “widely distributed throughout eastern China, Hong Kong and Hainan Is.”. However, since we lack definite evidence for the distribution of medaka in Hong Kong and this is not an essential point within this manuscript, we decided to remove “Hong Kong”. To point out the reference we followed in preparing Figure 1C, we now explicitly reference Parenti, 2008, in the figure legend. Additionally, we recognize reviewer #2’s concern and now stress that this is an approximation of distributional limits and not a map of known distribution. This modification was also suggested by reviewer #3. The respective section of the figure legend now reads:
”(C) Approximate distributional limits of Adrianichthyidae (black) following Parenti, 2008, with the locations from which some of the most famous medaka strains are derived (blue).”
6) Box 2's discussion of the pelvic brooding could benefit from the inclusion of the recently described species, Oryzias dopingdopingensis [Mandagi et al., 2018]. This species is a sister species of O. eversi and O. sarasinorum, both of which live in lake habitats and have pelvic fin brooding system. O. dopingdopingensis, on the other hand, lives in a river system and deposits its fertilized eggs on substrates around its habitat. The reviewers feel that these details are important in the context of the evolutionary scenario of the pelvic fin brooding system in Oryzias in Sulawesi.
We agree with the reviewers that the discovery of O. dopingdopingensis is very interesting and may add more detail to the discussion of pelvic brooding. However, O. eversi does not occur in a lake habitat (see Herder et al. 2012a). To further discuss the potential implication of the recent description of O. dopingdopingensis, but also point out the somewhat contradictory evidence for pelvic brooding as an open water adaptation, we modified the following section. “Pelvic brooding was suggested to have evolved in adaptation to the absence of suitable spawning substrates in pelagic habitats (Herder et al., 2012a). The recent discovery of the oviparous O. dopingdopingensis Mandagi et al., (2018), a sister species of the pelvic brooding O. sarasinorum and O. eversi, in doping-doping river might be considered as support for this hypothesis. However, the pelvic brooding O. eversi was also described from a river (Herder et al., 2012a). Consequently, the eco-evolutionary dynamics promoting pelvic brooding as well as the underlying molecular mechanisms remain virtually unknown.”
https://doi.org/10.7554/eLife.46994.009Article and author information
Author details
Funding
Leibniz Association (P91/2016)
- Julia Schwarzer
- Leon Hilgers
No external funding was received for this work.
Acknowledgements
We thank Fabian Herder, Manfred Schartl, Bernhard Misof, Arne Nolte and the entire AG RICEFISH for helpful discussions and comments to a previous version of this manuscript. We would further like to express our gratitude to Kiyoshi Naruse, Lynne Parenti and one anonymous reviewer as well as the editors Stuart King and Peter Rodgers, who helped improve this manuscript. Finally, we thank Felix Loosli, Jakob Geck, Hans-Georg Evers and Andreas Wagnitz for generously providing ricefish photos. This work was supported by the Leibniz association, grant P91/2016 to JS.
Publication history
- Received:
- Accepted:
- Version of Record published:
Copyright
© 2019, Hilgers and Schwarzer
This article is distributed under the terms of the Creative Commons Attribution License, which permits unrestricted use and redistribution provided that the original author and source are credited.
Metrics
-
- 5,817
- views
-
- 500
- downloads
-
- 42
- citations
Views, downloads and citations are aggregated across all versions of this paper published by eLife.
Download links
Downloads (link to download the article as PDF)
Open citations (links to open the citations from this article in various online reference manager services)
Cite this article (links to download the citations from this article in formats compatible with various reference manager tools)
Further reading
-
- Evolutionary Biology
The majority of highly polymorphic genes are related to immune functions and with over 100 alleles within a population, genes of the major histocompatibility complex (MHC) are the most polymorphic loci in vertebrates. How such extraordinary polymorphism arose and is maintained is controversial. One possibility is heterozygote advantage (HA), which can in principle maintain any number of alleles, but biologically explicit models based on this mechanism have so far failed to reliably predict the coexistence of significantly more than 10 alleles. We here present an eco-evolutionary model showing that evolution can result in the emergence and maintenance of more than 100 alleles under HA if the following two assumptions are fulfilled: first, pathogens are lethal in the absence of an appropriate immune defence; second, the effect of pathogens depends on host condition, with hosts in poorer condition being affected more strongly. Thus, our results show that HA can be a more potent force in explaining the extraordinary polymorphism found at MHC loci than currently recognised.
-
- Computational and Systems Biology
- Evolutionary Biology
As pathogens spread in a population of hosts, immunity is built up, and the pool of susceptible individuals are depleted. This generates selective pressure, to which many human RNA viruses, such as influenza virus or SARS-CoV-2, respond with rapid antigenic evolution and frequent emergence of immune evasive variants. However, the host’s immune systems adapt, and older immune responses wane, such that escape variants only enjoy a growth advantage for a limited time. If variant growth dynamics and reshaping of host-immunity operate on comparable time scales, viral adaptation is determined by eco-evolutionary interactions that are not captured by models of rapid evolution in a fixed environment. Here, we use a Susceptible/Infected model to describe the interaction between an evolving viral population in a dynamic but immunologically diverse host population. We show that depending on strain cross-immunity, heterogeneity of the host population, and durability of immune responses, escape variants initially grow exponentially, but lose their growth advantage before reaching high frequencies. Their subsequent dynamics follows an anomalous random walk determined by future escape variants and results in variant trajectories that are unpredictable. This model can explain the apparent contradiction between the clearly adaptive nature of antigenic evolution and the quasi-neutral dynamics of high-frequency variants observed for influenza viruses.