Ecdysone coordinates plastic growth with robust pattern in the developing wing
Figures
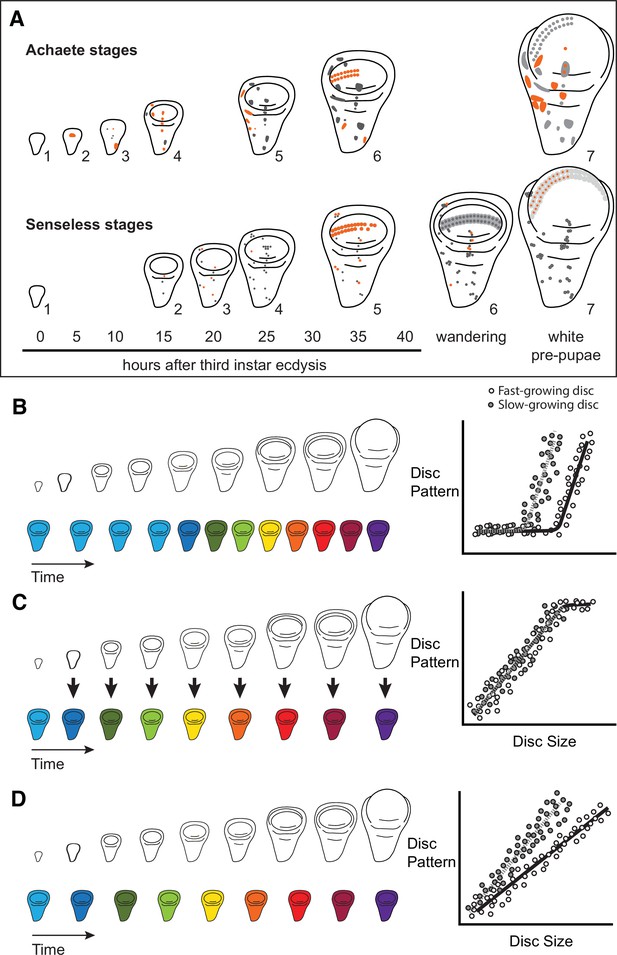
Quantitative assessments of the progression of patterning allow us to test hypotheses about the relationship between the size and patterning stage of the developing wing.
(A) The staging scheme developed by Oliveira et al., 2014 to quantify the progression of Achaete and Senseless pattern. The pattern elements shown in orange are diagnostic for each stage, which is indicated by the number beside the disc. (B–D) The relationship between wing disc size and patterning stage (represented as wing discs progressing through a series of colours) if (B) Hypothesis 1: wing discs grow first and then initiate pattern; (C) Hypothesis 2: wing disc patterning is regulated by wing disc size (arrows); and (D) Hypothesis 3: wing disc pattern and growth are regulated at least partially independently.
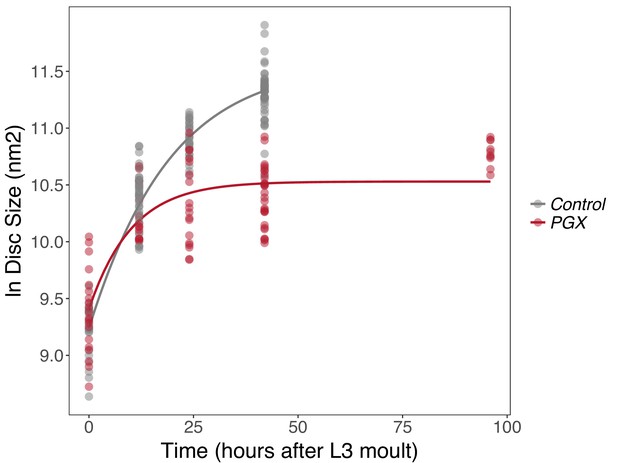
Growth rates of wing discs are reduced in larvae with genetically ablated prothoracic glands (PGX) versus control larvae.
Curves are Gompertz functions of disc size against time (hours after the third larval instar (L3) moult). Parameters for the curves are significantly different between PGX and control (Supplementary file 1a). Control genotypes are the pooled results from both parental controls (either the phm-GAL4; GAL80ts, or UAS-GRIM parental strain crossed to w1118). Each point represents the size of an individual wing disc. NPGX = 95, NControl = 125 across all time points.
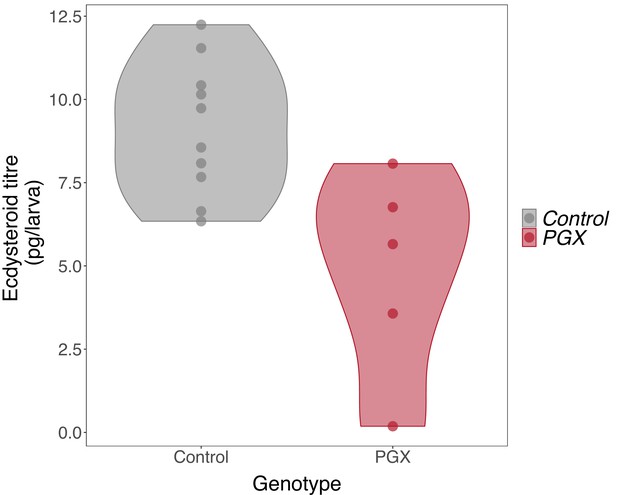
Ecdysteroid titres in genetically ablated prothoracic glands (PGX) and control larvae.
Newly ecdysed larvae were placed on sucrose/yeast diets. Ecdysteroid titres in control (phm> + and + >grim) are significantly higher ecdysteroid titres than PGX larvae, as determined by linear models and pairwise comparisons of the means (F-value1, 13 = 10.75, p-value=0.006, Ncontrol = 10, NPGX = 5). Data is plotted with violin plots, and the individual replicates (five per genotype) are included as points overlaying the violin plots.
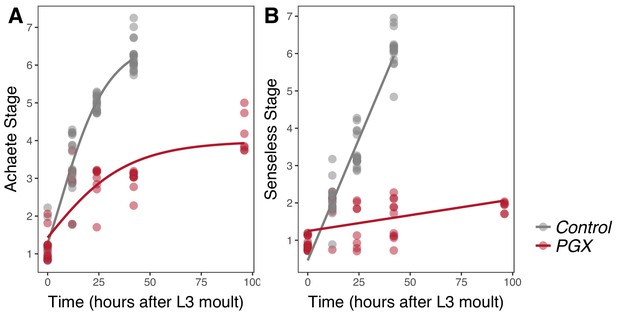
Achaete and Senseless patterning of wing discs is delayed in larvae with genetically ablated prothoracic glands (PGX) versus control larvae.
(A) Curves are Gompertz functions of Achaete stage against time (hours after the third instar (L3) moult). Parameters for the curves are significantly different between PGX and control (Supplementary file 1b). (B) Lines are linear regression of Senseless stage against time (hours after the L3 moult). Parameters for the lines are significantly different between PGX and control (Supplementary file 1c). Control genotypes are the pooled results from both parental controls (either the phm-GAL4; GAL80ts, or UAS-GRIM parental strain crossed to w1118). For Achaete: NPGX = 50, NControl = 61, for Senseless: NPGX = 52, NControl = 54 across all time points.
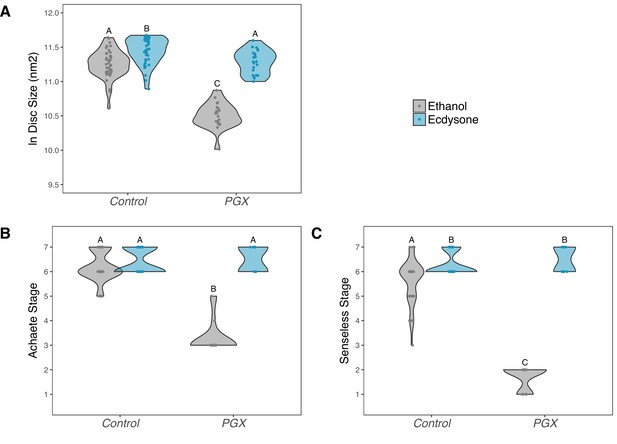
Supplementing genetically ablated prothoracic gland (PGX) larvae with 20-hydroxyecdysone (20E) rescues wing disc growth (A), and Achaete (B) and Senseless (C) patterning.
Both the control and PGX larvae were exposed to 20E-treated food (0.15 mg/mg of food) or ethanol-treated food (which contains the same volume of ethanol) at 0 hr after the third instar moult. Wing discs were removed at 42 hr after the third instar moult. Control genotypes are the pooled results from both parental controls (either the phm-GAL4; GAL80ts, or UAS-GRIM parental strain crossed to w1118). Treatments marked with different letters are significantly different (Tukey’s HSD, p<0.05, for ANOVA see Supplementary file 1d). Data were plotted using violin plots with individual wing discs displayed over the plots. NPGX + ethanol = 21, NPGX + 20E = 23, NControl + ethanol = 43, NControl + 20E = 42.
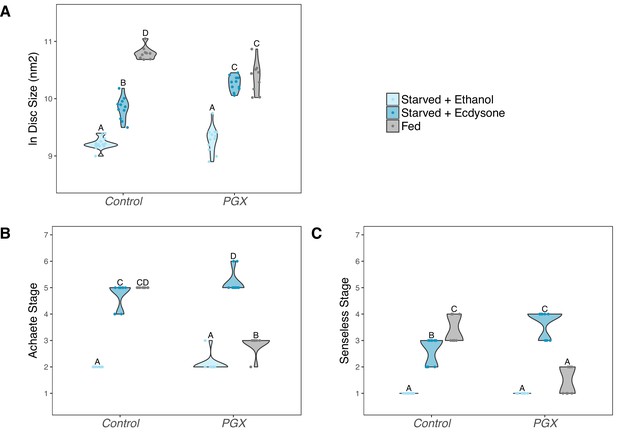
Supplementing genetically ablated prothoracic gland (PGX) larvae with 20-hydroxyecdysone (20E) is able to partially rescue the effect of yeast starvation on (A) wing discs growth, and fully rescue (B) Achaete and (C) Senseless patterning.
Both the control and PGX larvae were exposed from 0 hr after the third instar moult to one of three food types: (1) starved + 20 E – starvation medium containing 1% sucrose and 1% agar laced with 20E (0.15 mg/mg of food), (2) starved + ethanol – starvation medium treated with the same volume of ethanol, or (3) fed – normal fly food. Wing discs were removed at 24 hr after the third instar moult. Control genotypes are the pooled results from both parental controls (the UAS-GRIM parental strain crossed to w1118). Treatments marked with different letters are significantly different (Tukey’s HSD, p<0.05, for ANOVA see Supplementary file 1e). Data were plotted using violin plots with individual wing discs displayed over the plots. NPGX + starved - ethanol = 23, NPGX + starved - 20E = 22, NPGX + fed = 26, NControl + starved-ethanol = 28, NControl + starved-20E = 22, NControl + fed = 27.
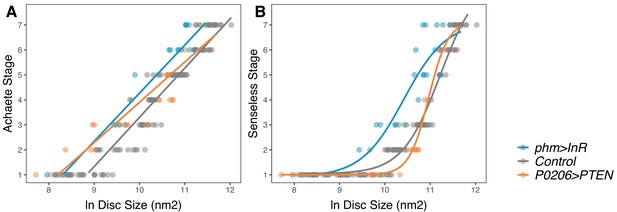
Changing rate of ecdysteroid synthesis changes the relationship between disc pattern and disc size throughout the third-instar larval stage.
(A) The relationship between Achaete stage and disc size was fitted with a linear regression, the parameters of which are significantly different between genotypes (Supplementary file 1f). (B) The relationship between Senseless stage and disc size was fitted with a four-parameter logistic regression, the parameters of which are significantly different between genotypes (Supplementary file 1g). We staged third instar larvae from the onset of the moult to the formation of white prepupae. The length of this developmental interval varied across genotypes. For control genotypes, we sampled larvae at 0, 10, 20, 30, 48, and 51 (InR control)/53 (PTEN control) hr after third instar ecdysis (AL3E). For phm>InR, we sampled larvae at 0, 10, 20, 29, 30, and 36 hr AL3E. For P0206>PTEN, we sampled larvae at 0, 10, 20, 30, 40, 60, 73, and 80 hr AL3E. The number of discs sampled for each genotype and patterning gene was: for Achaete: Nphm>InR = 29, NControl = 99, NP0206>PTEN = 40, for Senseless: Nphm>InR = 29, NControl = 99, NP0206>PTEN = 41.
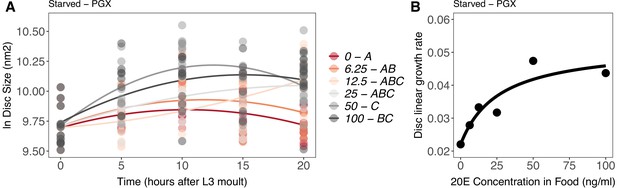
Effect of supplemental 20-hydroxyecdysone (20E) Supplementary file 1Eon growth of the wing imaginal disc in starved genetically ablated prothoracic gland (PGX) larvae.
Growth was modelled as S = E + T + T2 + E * T + E * T2, where S = disc size, E = 20E concentration, and T = disc age. (A) There was a significant effect of E on the linear growth rate of the wing imaginal discs (Supplementary file 1j). Each point corresponds to a wing disc, NPGX – starved = 409 (63–72 discs were sampled per treatment across all time points). 20E treatments that do not share a letter (see legend) are significantly different in patterning rates as determined by post-hoc test on the slopes (Supplementary file 1j). (B) The linear growth rate of the wing disc was extracted from the growth model for each concentration of 20E and modelled using a three-parameter Michaelis–Menten equation: y = c + (d-c)/(1 + (b/x)), where c is y at x = 0, d = y[max], and b is x where y is halfway between c and d. Linear growth rate increases steadily with ecdysone concentration in the food up until 25 ng of 20E/ml of food, after which growth rate increases more slowly with increasing 20E concentration.
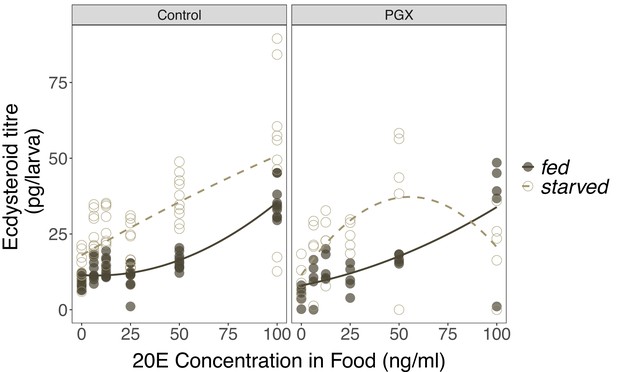
The effects of 20-hydroxyecdysone (20E) concentration in the food on ecdysteroid titres in control and genetically ablated prothoracic gland (PGX) larvae.
Newly ecdysed larvae were placed on sucrose/yeast (fed) or 20% sucrose/1% agar (starved) diets supplemented with a range of 20E concentrations for 20 hr. They were then transferred to the same diet without 20E and dyed with blue food colouring for 2 hr to eliminate residual 20E in the gut. Ecdysteroid titres in fed and starved control (phm> + and + >grim) and PGX larvae fed a range of 20E concentrations. There is a significant positive relationship between 20E concentration in the food and the concentration of ecdysteroids in the larvae, as indicated by a significant 20E term (Supplementary file 1h). Furthermore, starved larvae had higher ecdysone titres than fed larvae. Open and closed points represent the biological replicates, and solid and dashed lines are linear regressions. Ncontrol - starved = 60, Ncontrol - fed = 60, NPGX - starved = 30, NPGX - fed = 30 across all 20E concentrations.
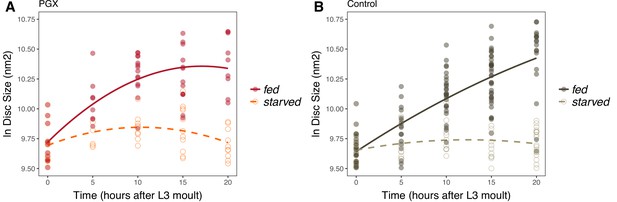
Wing imaginal disc growth is suppressed in fed genetically ablated prothoracic gland (PGX) larvae relative to controls and in starved larvae of both genotypes.
PGX (A) and control (B) larvae reared on sucrose/yeast (fed) or 20% sucrose/1% agar (starved) diets without 20E supplementation. Wing discs were sampled at 5 h intervals between 0-20 h after the moult to third instar. Wing disc growth was modelled as a quadratic, and there was a significant interaction between genotype (PGX vs. Control) and nutrition (fed vs. starved) on growth (Supplementary file 1i). Solid line/closed point = fed larvae, broken line/open point = starved larvae. Each point corresponds to a wing disc, NPGX - starved = 67, NPGX - fed = 74, Ncontrol - starved = 118, Ncontrol - fed = 151 across all time points.
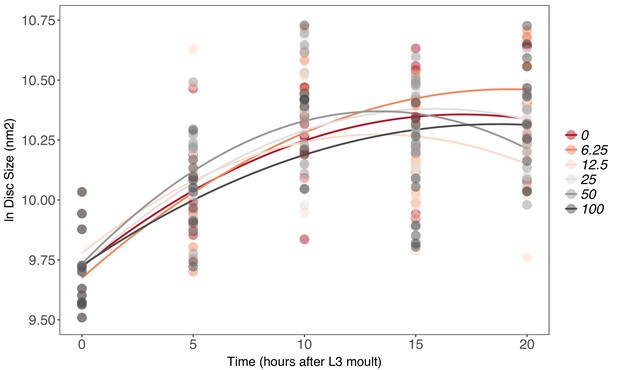
There is no effect of supplemental 20-hydroxyecdysone (20E) Supplementary file 1Eon growth of the wing imaginal disc in fed genetically ablated prothoracic gland (PGX) larvae.
Growth was modelled as S = E + T + T2 + E * T + E * T2, where S = disc size, E = 20E concentration, and T = disc age. There was no significant effect of E on the linear or quadratic growth rate of the wing imaginal discs (Supplementary file 1j). Each point corresponds to a wing disc, NPGX – fed = 459 (73–86 discs were sampled per treatment across all time points).
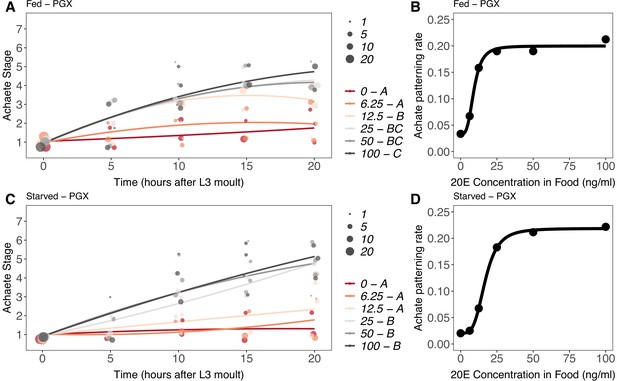
Effect of supplemental 20-hydroxyecdysone (20E) Supplementary file 1Eon Achaete patterning of the wing imaginal disc in (A, B) fed and (C, D) starved genetically ablated prothoracic gland (PGX) larvae.
In (A) and (C), patterning stage was modelled as A = E + T + T2 + E * T + E * T2, where A = Achaete stage, E = 20E concentration, and T = disc age. The size of each point corresponds to the number of wing disc in each stage. 20E treatments that do not share a letter (see legend) are significantly different in patterning rates as determined by post-hoc test on the slopes (for ANOVA, see Supplementary file 1l). In (B) and (D), we extracted the linear patterning rate from fed (B) or starved (D) PGX larvae. We then modelled the relationship between patterning rate and 20E concentration using a four-parameter log-logistic equation: y = c + (d)(–c)/(1 + e(b(log(x))-log(a))), where c is the lower asymptote, d is the upper asymptote, b is the rate of increase, and a is the inflection point. NPGX – fed = 459, NPGX – starved = 409, 63–86 discs were sampled per treatment across all time points.
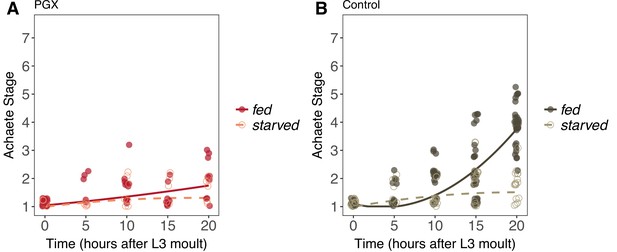
Achaete patterning in wing discs from fed and starved genetically ablated prothoracic gland (PGX) and control larvae.
(A) Patterning does not progress in either fed or starved PGX larvae. (B) Patterning does not progress in starved control larvae but does in fed control larvae. There is a significant interaction between the effects of disc age and food on Achaete patterning in control larvae (orthogonal polynomial regression: = 67.98, p<0.001), but not in PGX larvae (orthogonal polynomial regression: = 1.81, p=0.163). Each point corresponds to a wing disc, NPGX - starved = 67, NPGX - fed = 74, Ncontrol - starved = 118, Ncontrol - fed = 151 across all time points.
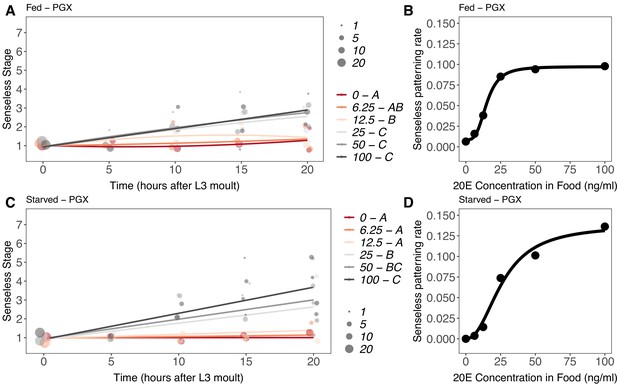
Effect of supplemental 20-hydroxyecdysone (20E) Supplementary file 1Eon Senseless patterning of the wing imaginal disc in (A, B) fed and (C, D) starved genetically ablated prothoracic gland (PGX) larvae.
In (A) and (C), patterning stage was modelled as S = E + T + T2 + E * T + E * T2, where S = Senseless stage, E = 20E concentration, and T = disc age. The size of each point corresponds to the number of wing discs in each stage. 20E treatments that do not share a letter (see legend) are significantly different in patterning rates as determined by post-hoc test on the slopes (for ANOVA, see Supplementary file 1m). In (B) and (D), we extracted the linear patterning rate in fed (B) or starved (D) larvae. We then modelled the relationship between patterning rate and 20E concentration in (B) using a four-parameter log-logistic equation: y = c + (d)(–c)/(1 + e(b(log(x))-log(a))), where c is the lower asymptote, d is the upper asymptote, b is the rate of increase, and a is the inflection point. In (D), we used a three-parameter log-logistic equation: y = d/(1 + e(b(log(x))-log(a))), where d is the upper asymptote, b is the rate of increase, and a is the inflection point. NPGX – fed = 459, NPGX – starved = 409, 63–86 discs were sampled per treatment across all time points.
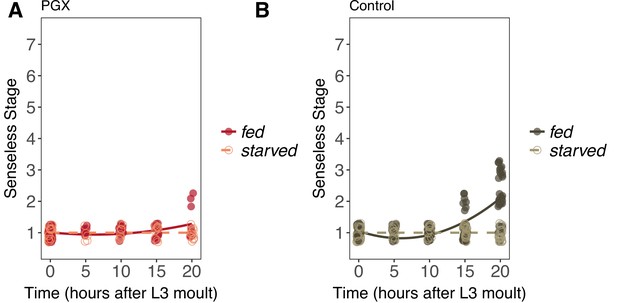
Senseless patterning in wing discs from fed and starved genetically ablated prothoracic gland (PGX) and control larvae.
(A) In PGX larvae, Senseless patterning does not progress at all in starved larvae (linear regression: = 0.057, p=0.82), but does in fed larvae (linear regression: = 9.76, p<0.01). (B) Patterning does not progress in starved control larvae but does in fed control larvae, resulting in a significant interaction between the effects of time and food on Senseless patterning in control larvae (linear regression: = 67.98, p<0.001). Control genotypes are the pooled results from both parental controls (either the phm-GAL4; GAL80ts, or UAS-GRIM parental strain crossed to w1118). Each point corresponds to a wing disc, NPGX - starved = 67, NPGX - fed = 74, Ncontrol - starved = 118, Ncontrol - fed = 151 across all time points.
Additional files
-
Transparent reporting form
- https://cdn.elifesciences.org/articles/72666/elife-72666-transrepform1-v2.docx
-
Supplementary file 1
Tables - Results of Statistical Analyses.
- https://cdn.elifesciences.org/articles/72666/elife-72666-supp1-v2.docx