Resurrecting essential amino acid biosynthesis in mammalian cells
Figures
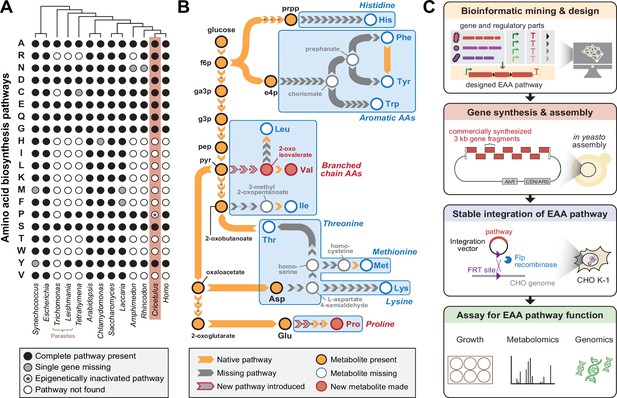
Engineering Essential Amino Acid (EAA) biosynthesis in metazoan cells.
(A) Presence of amino acid biosynthesis pathways across representative diverse organisms on Earth. (B) Schematic of EAA biosynthesis pathway steps that require engineering in mammalian cells to enable complete amino acid prototrophy if imported from Escherichia coli. Proline and Valine pathways shown in this work are highlighted in red. (C) Workflow diagram of a synthetic genomics approach involving pathway design, construction, integration and testing towards mammalian EAA restoration.
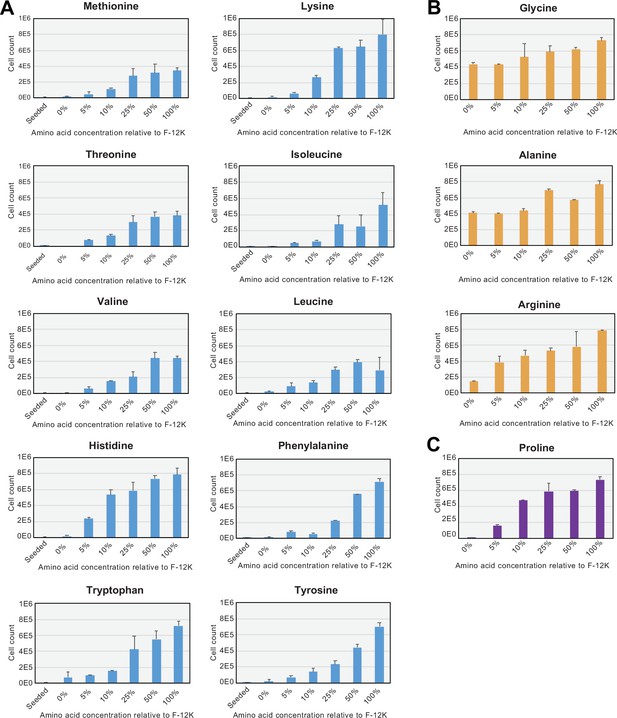
Amino acid dropout growth assays in CHO-K1.
(A) Growth assays on Chinese hamster ovary (CHO)-K1 cells seeded into media with reduced or omitted essential amino acids (Figure 1—figure supplement 1—source data 1). Percentages represent the relative media amino acid concentration compared to standard F-12K medium. 100% corresponds to 0.02 mM (Trp), 0.05 mM (Tyr), 0.06 mM (Ile, Met, Phe), 0.2 mM (Ala, Asn, Asp, Glu, Gly, Leu, Ser, Thr, Val), 0.22 mM (His), 0.4 mM (Cys, Lys), 0.6 mM (Pro), and 2 mM (Arg, Gln), respectively. Error bars show SD of three replicates. (B) CHO-K1 cells grow with minimal defects in F-12K medium lacking glycine and alanine, but are more sensitive to arginine starvation (Figure 1—figure supplement 1—source data 1). (C) CHO cells do not grow without exogenous proline, confirming the known epigenetic proline auxotrophy found in this cell line (Figure 1—figure supplement 1—source data 1). Error bars represent data from three replicates.
-
Figure 1—figure supplement 1—source data 1
Raw cell count data for amino acid dropout curves.
- https://cdn.elifesciences.org/articles/72847/elife-72847-fig1-figsupp1-data1-v2.zip
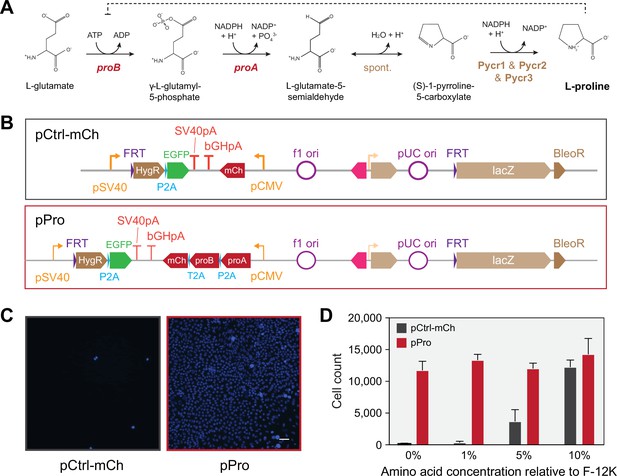
Imported bacterial genes rescue CHO epigenetic proline auxotrophy.
(A) Proline biosynthetic pathway from glutamate. (B) Schematic representation of two constructs to test bacterial gene rescue of Chinese hamster ovary (CHO)-K1 auxotrophy, one expressing the E. coli proA and proB genes, encoding glutamate-5-semialdehyde dehydrogenase and glutamate-5-kinase, respectively (pPro), and a control construct expressing only mCherry (pCtrl-mCh). Constructs are shown as they appear following integration into the Flp-In CHO line. (C) Hoechst 33348 live nuclei microscopy shows cells expressing the pPro construct grow in the absence of proline and appear healthy. Scale bar represents 50 μm and is shared across images (D) Growth assay of cells seeded into proline-free F-12K medium and grown for 5 days (Figure 1—figure supplement 2—source data 1). The bacterial gene pPro pathway resulted in robust growth recovery in proline-free medium. Error bars represent data from three replicates.
-
Figure 1—figure supplement 2—source data 1
Raw and processed data for proline auxotrophy rescue by proAB construct.
- https://cdn.elifesciences.org/articles/72847/elife-72847-fig1-figsupp2-data1-v2.zip
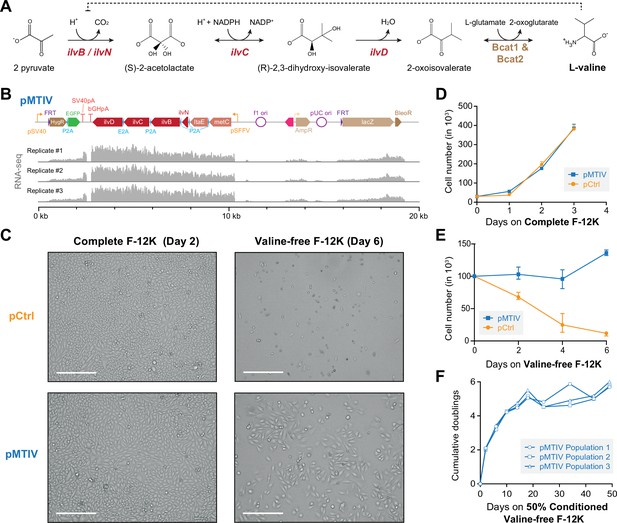
Restoration of a valine biosynthesis pathway in CHO-K1 cells.
(A) Three enzymatic steps encoded by E. coli genes ilvN (regulatory subunit, acetolactate synthase), ilvB (catalytic subunit, acetolactate synthase), ilvC (ketol-acid reductoisomerase), and ilvD (dihydroxy-acid dehydratase) are required for valine biosynthesis in Chinese hamster ovary (CHO)-K1 cells. (B) Schematic of pMTIV construct after genomic integration and RNA-seq read coverage showing successful incorporation and active transcription. (C) Microscopy images of CHO-K1 cells with integrated pCtrl or pMTIV constructs in complete F-12K medium after 2 days or valine-free F-12K medium after 6 days. Scale bar represents 300 µm. (D) Growth curve of CHO-K1 cells with pCtrl or pMTIV in complete F-12K medium (Figure 2—source data 1). Day-0 indicates number of seeded cells. Error bars represent data from three replicates. (E) Growth curve of CHO-K1 cells with pCtrl or pMTIV in valine-free F-12K medium (Figure 2—source data 1). Day-0 indicates number of seeded cells. Error bars represent data from three replicates. (F) CHO-K1 with pMTIV cultured over nine passages in 50% conditioned valine-free F-12K medium (Figure 2—source data 1).
-
Figure 2—source data 1
Raw cell count data for pMTIV valine-free and complete F-12K medium tests.
- https://cdn.elifesciences.org/articles/72847/elife-72847-fig2-data1-v2.zip
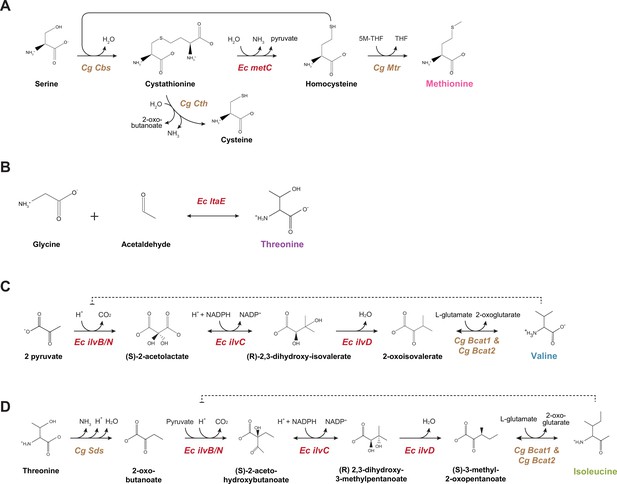
Schematic outlines of the MTIV pathways.
(A) Pathway for methionine biosynthesis. (B) Pathway for threonine biosynthesis. Note the shared multifunctional enzymes in (C) and (D) responsible for both isoleucine and valine production. In all panels ‘Ec’ refers to E. coli, and ‘Cg’ to C. griseus, the native Chinese hamster ovary (CHO) parental organism. Red genes indicate steps missing in CHO metabolism.
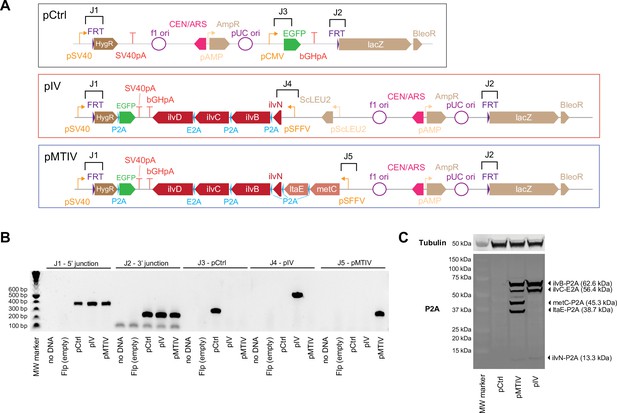
Confirmation of BCAA biosynthetic construct integration and 2A peptide processing by PCR and Western blot.
(A) Construct were designed with PCR landing pads flanking fragment junctions for rapid screening during construct assembly in yeasto, shown above construct diagrams with brackets. (B) PCRs across these junctions confirm correct assembly of the control, pMTIV, and pIV constructs (Figure 2—figure supplement 2—source data 1). (C) Immunoblotting against P2A peptides in pMTIV and pIV cells confirms 2A peptides are processed as expected (Figure 2—figure supplement 2—source data 2). Note that ilvD (65.5 kDa) is not detectable because it lacks a terminal P2A tag.
-
Figure 2—figure supplement 2—source data 1
Raw and annotated images of assembly junction PCR gel.
- https://cdn.elifesciences.org/articles/72847/elife-72847-fig2-figsupp2-data1-v2.zip
-
Figure 2—figure supplement 2—source data 2
Raw and annotated images of 2 A Western blot.
- https://cdn.elifesciences.org/articles/72847/elife-72847-fig2-figsupp2-data2-v2.zip
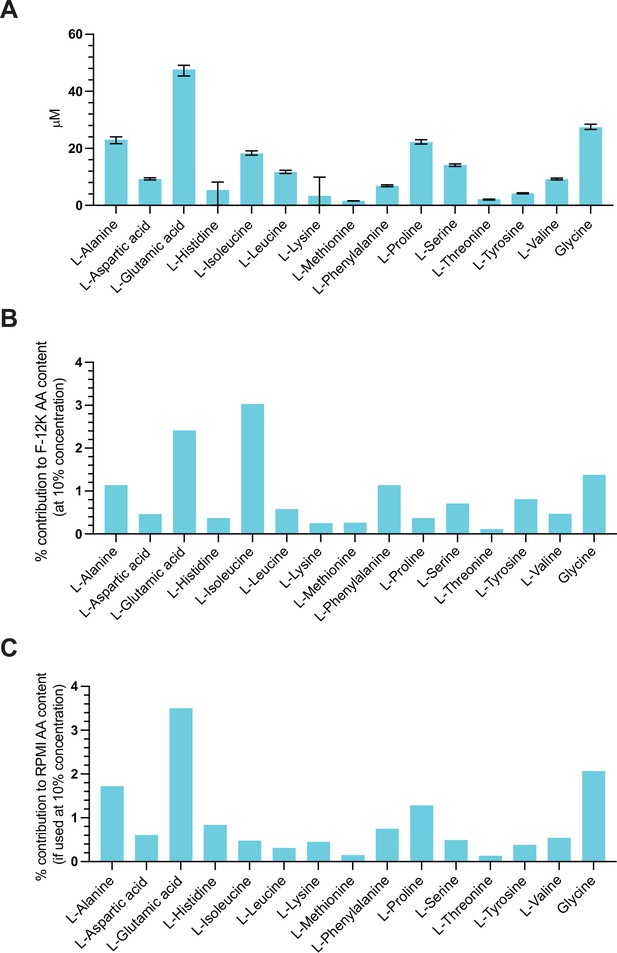
Amino acid content in dialyzed fetal bovine serum.
(A) Concentration of 15 different proteinogenic amino acids in dialyzed fetal bovine serum. Error bars represent data from three replicates (Figure 2—figure supplement 3—source data 1). (B) The amino acid contribution of dialyzed fetal bovine serum to non-supplemented F-12K medium when supplemented at 10% concentration. (C) The amino acid contribution of dialyzed fetal bovine serum to non-supplemented Roswell Park Memorial Institute (RPMI) medium when supplemented at 10% concentration.
-
Figure 2—figure supplement 3—source data 1
Raw and processed MS/MS data for dialyzed FBS amino acid measurements.
- https://cdn.elifesciences.org/articles/72847/elife-72847-fig2-figsupp3-data1-v2.zip
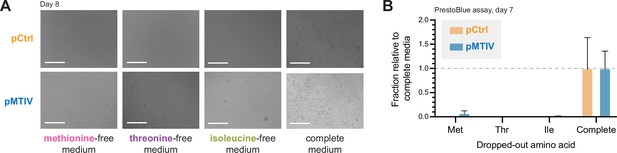
Lack of rescue of methionine, threonine, and isoleucine auxotrophy by pMTIV construct in Chinese hamster ovary (CHO)-K1 cells.
(A) Phase-contrast images of cells carrying either pMTIV and pCtrl constructs after 8 days growth on EAA-free F-12K media (three leftmost panels) or complete F-12K medium (rightmost panel). Scale bar represents 300 µm. (B) PrestoBlue cell viability assay of growth on EAA-free or complete media after 7 days’ growth (Figure 2—figure supplement 4—source data 1). Error bars represent data from three replicates.
-
Figure 2—figure supplement 4—source data 1
Raw PrestoBlue assay values for MTIV construct methionine, threonine and isoleucine dropout tests Figure 2—figure supplement 4B.
- https://cdn.elifesciences.org/articles/72847/elife-72847-fig2-figsupp4-data1-v2.zip
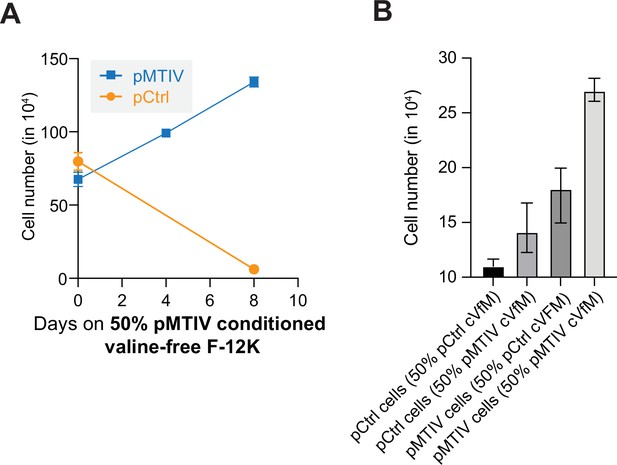
Growth behaviors on conditioned valine-free F-12K medium.
(A) Growth and death of pMTIV and pCtrl cells on 50% conditioned valine-free F-12K medium. Error bars represent data from three replicates. (Figure 2—figure supplement 5—source data 1). (B) Comparison of pCtrl and pMTIV cells grown on 50% conditioned valine-free F-12K medium using medium conditioned by pCtrl (50% pCtrl cVfM) and medium conditioned by pMTIV cells (50% pMTIV cVfM) as indicated (Figure 2—figure supplement 5—source data 1). Error bars represent data from three replicates.
-
Figure 2—figure supplement 5—source data 1
Raw cell count data for pCtrl and pMTIV cells cultured in 50% conditioned valine-free F-12K medium.
- https://cdn.elifesciences.org/articles/72847/elife-72847-fig2-figsupp5-data1-v2.zip
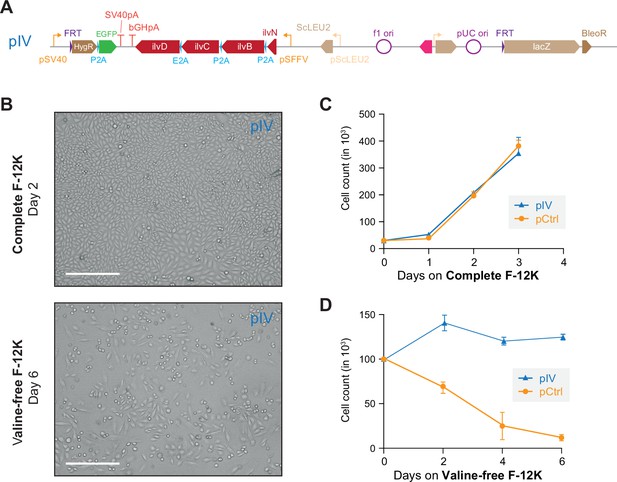
The pIV construct rescues valine auxotrophy.
(A) The minimized four-gene construct pIV lacking ltaE-encoded L-threonine aldoase and metC-encoded cystathionine-ß-lyase after integration into the Chinese hamster ovary (CHO) genome. (B) Microscopy of pIV cells in both complete and valine-free F-12K medium. The pIV constructs rescue cellular morphology in valine-free F-12K medium. Scale bar represents 300 µm. (C) Growth of pIV cells in complete F-12K medium (Figure 2—figure supplement 6—source data 1). Error bars represent data from three replicates. (D) Live cell counting of pIV cells grown on valine-free F-12K (Figure 2—figure supplement 6—source data 1). Error bars represent data from three replicates.
-
Figure 2—figure supplement 6—source data 1
Raw cell count data for pIV valine-free and complete F-12K medium tests.
- https://cdn.elifesciences.org/articles/72847/elife-72847-fig2-figsupp6-data1-v2.zip
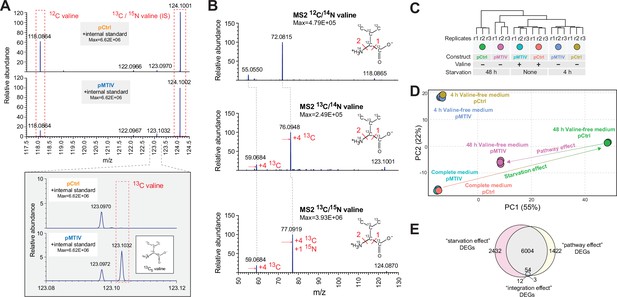
Heavy-carbon validation of endogenous valine production and transcriptomic signatures associated with rescue of nutritional starvation.
(A) Mass spectra showing an MS1 peak corresponding to the expected m/z for 13C-valine when pMTIV cells were grown on valine-free RPMI medium supplemented with 13C-glucose and 13C-pyruvate, indicating autogenous production of intracellular valine (Figure 3—source data 1). (B) MS2 performed on peaks extracted from pMTIV sample, which corresponded to m/z values expected for 12C-valine, 13C-valine and internal standard 13C/15N-valine. MS2 fragmentation patterns for each of these metabolites matched expectations (Figure 3—source data 1). (C) RNA-seq dendrogram of pCtrl cells and pMTIV cells grown on complete F-12K medium or starved of valine for 4 hr or 48 hr. (D) Principal Component Analysis (PCA) space depiction of pCtrl cells and pMTIV cells grown on complete F-12K medium, or starved of valine for 4 hr or 48 hr. (E) Overlap between differently expressed genes (DEGs) comparing pCtrl cells cultured in valine-free F-12K medium to pCtrl cells cultured in complete F-12K medium (the ‘starvation effect’), pCtrl cells and pMTIV cells after 48 hr starvation on valine-free F-12K medium (the ‘pathway effect’), and pCtrl cells and pMTIV cells grown on complete F-12K medium (the ‘integration effect’).
-
Figure 3—source data 1
Raw MS/MS data for 13C valine labeling experiments.
- https://cdn.elifesciences.org/articles/72847/elife-72847-fig3-data1-v2.zip
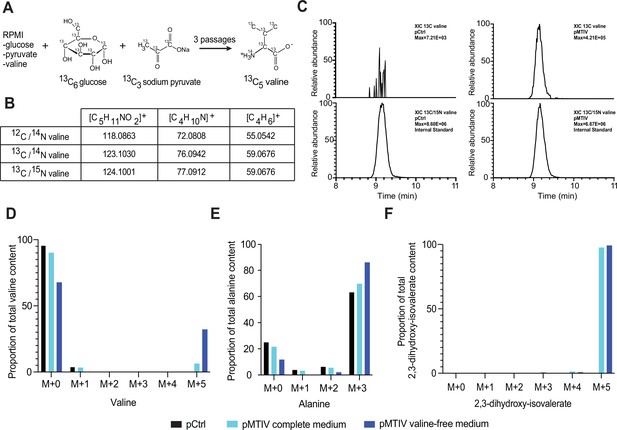
Heavy-labeled metabolomics confirms endogenous valine production.
(A) Schematic outline of the 13C-labeling strategy for detection of biosynthesized valine. (B) Expected m/z ratios for 12C-valine, 13C-valine, and spiked-in internal standard 13C/15N-valine in unfragmented and fragmented states. (C) Extracted ion chromatography shows that the presumed 13C-valine found in pMTIV cells runs similarly to spiked-in internal standard 13C/15N-valine, further confirming that 13C-valine is produced in pMTIV cells (Figure 3—figure supplement 1—source data 1). The presence of 13C-valine is specific to pMTIV cells and absent from control cells. (D). In theory, partial 13C-labeling of valine is possible if just one of the two pyruvate substrates is 13C-labeled. Partially 13C-labeled (13C2, 13C3, 13C4) valine species were below the level of detection. Therefore, the proportion of 13C5-valine relative to total valine abundance within the cell can serve as a proxy for the degree to which intracellular valine biosynthesis is meeting cellular valine demands. The lesser occurrence of 13C1-valine can be explained by the natural abundance of 13C. 32.2% of total valine abundance in pMTIV cells cultured in valine-free RPMI medium was 13C5-labeled, whereas the corresponding proportion for pMTIV cells cultured in complete RPMI medium was 6.4% (Figure 3—figure supplement 1—source data 1). (E) Non-essential amino acid alanine is biosynthesized from pyruvate and is not found in RPMI medium. Alanine can thus serve a proxy for proteome turnover assuming similar usage rates for alanine and valine. 86.1% of alanine was 13C3-alanine in pMTIV cells cultured in valine-free RPMI medium (Figure 3—figure supplement 1—source data 1). (F) 13C-labeled pathway intermediate 2,3-dihydroxy-isovalerate was detected in pMTIV cells cultured in complete and in valine-free RPMI conditions (Figure 3—figure supplement 1—source data 1).
-
Figure 3—figure supplement 1—source data 1
Further raw MS/MS data for 13C valine labeling experiments.
- https://cdn.elifesciences.org/articles/72847/elife-72847-fig3-figsupp1-data1-v2.zip
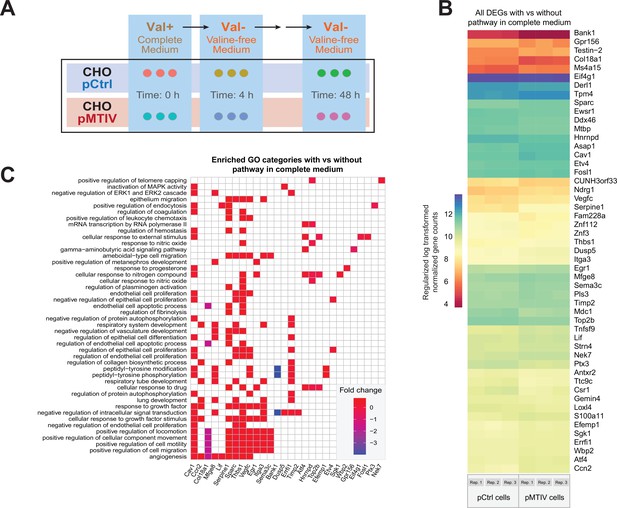
Transcriptomic analysis of pMTIV pathway effect in complete F-12K medium.
(A) Schematic representation of the starvation regimen applied prior to collecting samples for transcriptomic analysis. (B) Regularized log fold changes in expression across the 51 differentially expressed genes when comparing pMTIV cells to pCtrl cells grown in complete medium. Values from three replicates are plotted side-by-side in each column. (C) Gene ontology (GO) category enrichment among the 51 differentially expressed genes.
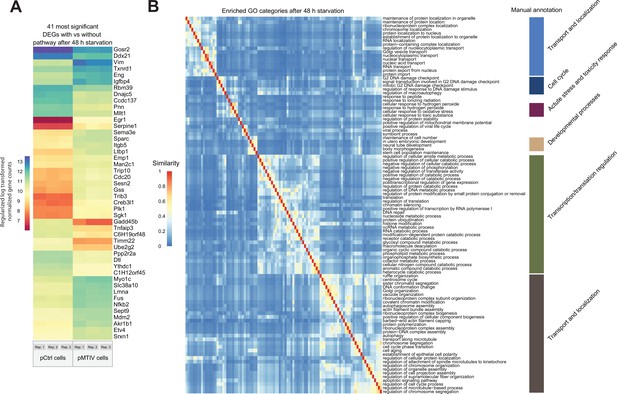
Transcriptomic analysis of pMTIV pathway effect in valine-free F-12K medium.
(A) Regularized log transformed expression of the 50 most significant differentially expressed genes when comparing pMTIV cells to pCtrl cells after 48 hr in valine-free F-12K medium. Values from three replicates are plotted side-by-side in each column. (B) Gene ontology (GO) category enrichment among these genes, clustered and colored using a GO ontology distance metric, and manually grouped into larger supercategories, on the right.
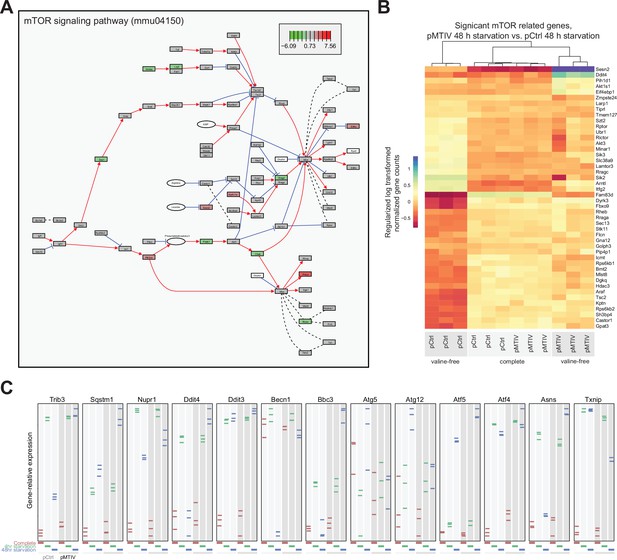
Transcriptomic analysis of pMTIV pathway effect on mTOR and the integrated stress response.
(A) Kyoto Encyclopedia of Genes and Genomes (KEGG) representation of the mouse mTOR pathway showing individual node gene up/down regulation. (B) Significantly differently expressed mTOR-related genes after 48 hr valine starvation in pMTIV cells. (C) Significantly differently expressed Integrated Stress Response-related genes after 48 hr valine starvation in pMTIV cells.
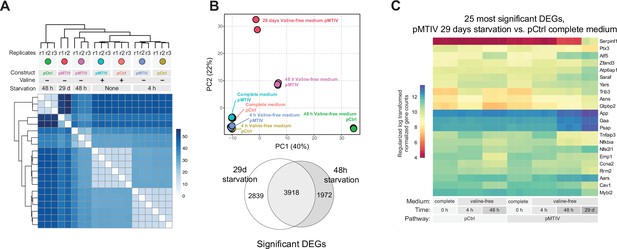
Transcriptomic analysis of long-term (29 days) pMTIV pathway effect in conditioned valine-free F-12K medium.
(A) Regularized log transformed transcriptomic signatures of cells harboring the pMTIV or pCtrl constructs grown on complete F-12K medium or starved of valine for 4 hr, 48 hr, or 29 days (5 passages). (B) A PCA plot of starved pMTIV and pCtrl cells, and Euler diagram of the overlap between DEGs from 29 days of starvation and 48 hr starvation compared to pCtrl cells grown on complete F-12K medium. (C) A heatmap of the 25 most significant differently expressed genes between pMTIV cells starved of valine for 29 days and pCtrl cells grown on complete F-12K medium.
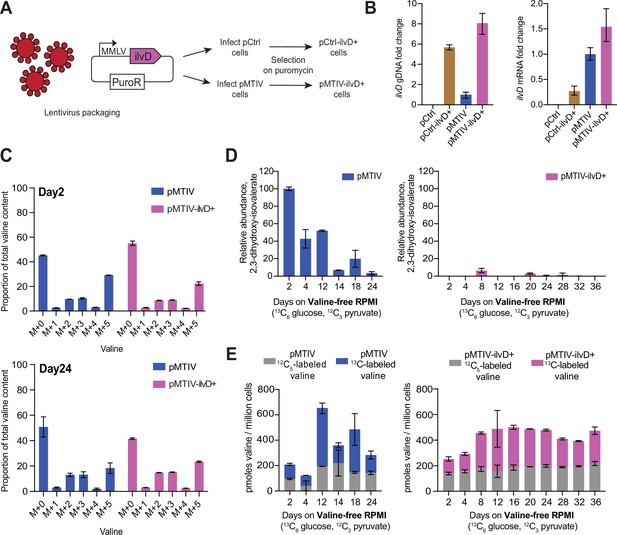
Optimization of prototrophic functionality reduces pathway bottlenecking and stabilizes valine availability at late time points in valine-free conditions.
(A) Infection of pCtrl and pMTIV cells using a lentivirus carrying extra copies of ilvD led to the generation of the pCtrl-ilvD + and pMTIV-ilvD + cell lines. (B) ilvD qPCR on gDNA and cDNA from each cell line (Figure 4—source data 1). Fold change levels were relativized to pMTIV. cDNA was reverse transcribed using oligo(dT) primers from RNA templates collected from each cell line. Error bars show SD of three technical replicates. (C)13C-labeling of valine in pMTIV and pMTIV-ilvD + samples collected on day-2 and day-24 of culture in unconditioned, reduced-isoleucine (0.06 mM), valine-free RPMI medium containing 13C6-glucose with 2 mM 12C-sodium pyruvate spiked-in on plates coated with 0.1% gelatin (Figure 4—source data 1). Error bars represent data from two replicates. (D) Relative abundance of pathway intermediate 2,3-dihydroxy-isovalerate in pMTIV and pMTIV-ilvD + cells cultured as in (C) (Figure 4—source data 1). Error bars represent data from two replicates. (E) Molar concentration of valine in pMTIV and pMTIV-ilvD + cells cultured as in (C) (Figure 4—source data 1). 12C-labeled refers to valine carrying 12C exclusively while 13C-labeled refers to valine carrying at least one 13C. Error bars represent data from two replicates.
-
Figure 4—source data 1
Control and ilvD qPCR data for pMTIV and pMTIV-ilvD + cells, raw cell count data for pMTIV cultured in valine-free RPMI with different BCAA concentrations, and MS/MS data for long-term pMTIV and pMTIV-ilvD+ 13C valine labeling experiments.
- https://cdn.elifesciences.org/articles/72847/elife-72847-fig4-data1-v2.zip
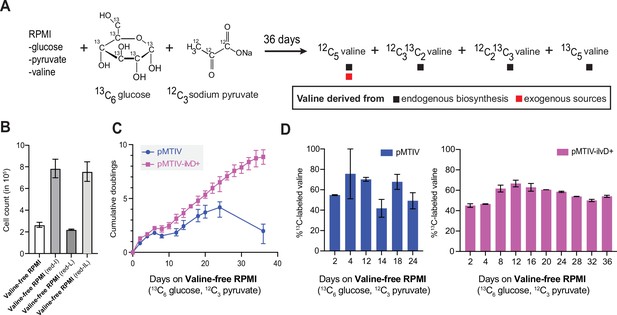
Long-term culturing of cells in unconditioned isotopically heavy valine-free RPMI medium.
(A) Schematic outline of the 13C-labeling strategy for detection of biosynthesized valine. Culturing regimen in isotopically heavy RPMI using 13C6-glucose and 12C3-sodium pyruvate should in principle result in the generation of 12C5-valine (from 2 12C3 sodium pyruvate), 12C313C2 valine and 12C213C3 valine (from 1 12C3 and 1 13C3-sodium pyruvate), and 13C5 (from 2 13C3-sodium pyruvate). (B) pMTIV cells cultured in valine-free RPMI (0.38 mM Ile, 0.38 mM Leu), reduced-isoleucine valine-free RPMI (0.06 mM Ile, 0.38 mM Leu), reduced-leucine valine-free RPMI (0.38 mM Ile, 0.2 mM Leu), and in reduced-isoleucine reduced-leucine valine-free RPMI (0.06 mM Ile, 0.2 mM Leu) (Figure 4—figure supplement 1—source data 1). Triplicate values are plotted. (C) Population doubling level of pMTIV and pMTIV-ilvD + cells cultured in isotopically heavy valine-free RPMI medium as described in (A). Error bars represent data from three replicates. (Figure 4—figure supplement 1—source data 1). (D) % of valine carrying at least one 13C in pMTIV and pMTIV-ilvD + cells cultured on unconditioned, reduced-isoleucine, valine-free RPMI medium containing 13C6-glucose and 2 mM 12C-sodium pyruvate on plates coated with 0.1% gelatin. Error bars represent data from two replicates. (Figure 4—figure supplement 1—source data 1).
-
Figure 4—figure supplement 1—source data 1
Growth curve and MS/MS data for long-term pMTIV and pMTIV-ilvD+ 13C valine labeling experiments.
- https://cdn.elifesciences.org/articles/72847/elife-72847-fig4-figsupp1-data1-v2.zip
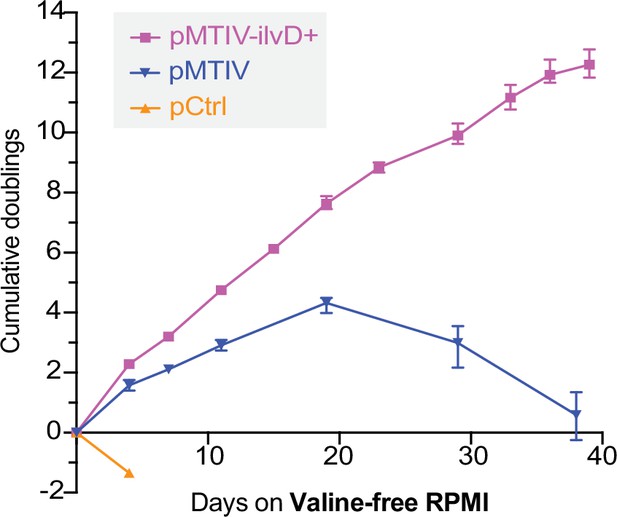
Optimized prototrophic cells double every 3.2 days in unconditioned valine-free RPMI medium over 39 days.
Population doubling level of pCtrl, pMTIV, pMTIV-ilvD + cells cultured in unconditioned, reduced-isoleucine, valine-free RPMI medium containing 2 mM sodium pyruvate (Figure 5—source data 1). Plates were not coated with gelatin. Error bars represent data from three replicates.
-
Figure 5—source data 1
Raw and processed cell count data for pMTIV and pMTIV-ilvD + cultured long-term in unconditioned valine-free RPMI medium.
- https://cdn.elifesciences.org/articles/72847/elife-72847-fig5-data1-v2.zip
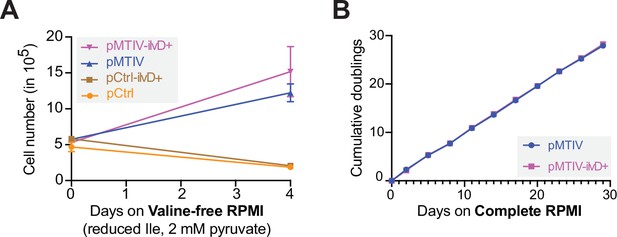
Comparing growth of cell lines with elevated ilvD copy numbers to those without.
(A) Comparison of growth in unconditioned reduced-isoleucine valine-free RPMI containing 2 mM sodium pyruvate (Figure 5—figure supplement 1—source data 1). Error bars represent data from three replicates. (B) Comparison of pMTIV and pMTIV-ilvD + long term growth in reconstituted complete RPMI (Figure 5—figure supplement 1—source data 1). Error bars represent data from three replicates.
-
Figure 5—figure supplement 1—source data 1
Raw cell count data for pCtrl, pMTIV, pCtrl-ilvD + and pMTIV-ilvD + cultured in valine-free and complete RPMI medium.
- https://cdn.elifesciences.org/articles/72847/elife-72847-fig5-figsupp1-data1-v2.zip
Tables
Reagent type (species) or resource | Designation | Source or reference | Identifiers | Additional information |
---|---|---|---|---|
Strain, strain background (E. coli) | TransforMax EPI300 | Lucigen | C300C105 | Chemically competent |
Strain, strain background (E. coli) | NEB 10-beta | New England Biolabs | C3020K | Electrocompetent |
Cell line (C. griseus) | CHO Flp-In | ThermoFisher | R75807 | |
Cell line (C. griseus) | CHO pMTIV | This paper | Cell line maintained in J.D.Boeke lab | |
Cell line (C. griseus) | CHO pCtrl | This paper | Cell line maintained in J.D.Boeke lab | |
Cell line (C. griseus) | CHO pMTIV-ilvD+ | This paper | Cell line maintained in J.D.Boeke lab | |
Cell line (C. griseus) | CHO pCtrl-ilvD+ | This paper | Cell line maintained in J.D.Boeke lab | |
Cell line (C. griseus) | CHO pPro | This paper | Cell line maintained in H.H.Wang lab | |
Cell line (C. griseus) | CHO pCtrl-mCh | This paper | Cell line maintained in H.H.Wang lab | |
Recombinant DNA reagent | pJTR0381 (plasmid) | This paper | Encodes Ec ilvD transcription units for lentiviral transduction | |
Antibody | Anti-2A peptide (mouse monoclonal) | Novus Biologicals | NBP2-59627 | 1:1000 dilution |
Antibody | Anti-α/β-tubulin (rabbit polyclonal) | Cell Signaling Technology | 2148 | 1:1000 dilution |
Antibody | IRDye 800CW Anti-mouse IgG (goat polyclonal) | LI-COR | 926–32210 | 1:20000 dilution |
Antibody | IRDye 680 RD Anti-rabbit IgG (goat polyclonal) | LI-COR | 926–68071 | 1:20000 dilution |
Commercial assay or kit | PrestoBlue Cell Viability Reagent | ThermoFisher | A13261 | |
Commercial assay or kit | NEBNext poly(A) mRNA Magnetic Isolation module | New England Biolabs | E7490 | |
Commercial assay or kit | NEBNext Ultra RNA Library Prep Kit for Illumina | New England Biolabs | E7770 | |
Other | F-12K medium without amino acids, powder base | US Biological | N8545 | Dropout medium base |
Other | RPMI 1640 without amino acids & glucose, powder base | US Biological | R9010-01 | Dropout medium base |
Other | Gibco fetal bovine serum, dialyzed | Fisher Scientific | 26400044 | Lot 2140178 |
-
CHO: Chinese hamster ovary.
Additional files
-
Supplementary file 1
Table of minimal prototrophic pathways set for AA prototrophy in mammalian cells.
- https://cdn.elifesciences.org/articles/72847/elife-72847-supp1-v2.xlsx
-
Supplementary file 2
Protein sequences for each gene listed in Supplementary file 1.
- https://cdn.elifesciences.org/articles/72847/elife-72847-supp2-v2.xlsx
-
Supplementary file 3
Codon-optimized nucleotide sequence for each gene listed in Supplementary file 1.
- https://cdn.elifesciences.org/articles/72847/elife-72847-supp3-v2.xlsx
-
Supplementary file 4
Complete amino acid prototrophy pathway EC numbers.
- https://cdn.elifesciences.org/articles/72847/elife-72847-supp4-v2.xlsx
-
Transparent reporting form
- https://cdn.elifesciences.org/articles/72847/elife-72847-transrepform1-v2.pdf