Conserved structural elements specialize ATAD1 as a membrane protein extraction machine
Figures
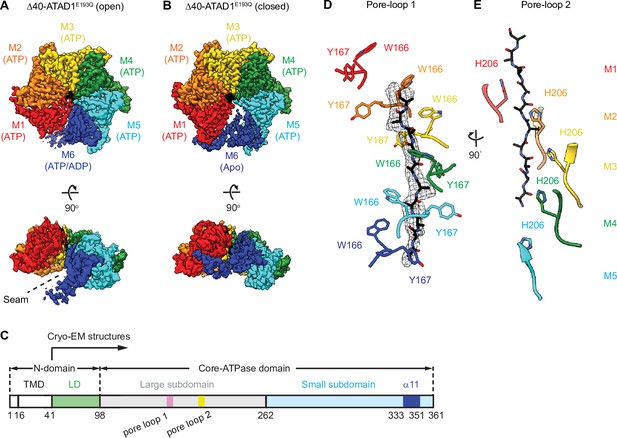
Architecture of the Δ40-ATAD1E193Q-substrate complexes.
(A and B) Final reconstructions of Δ40-ATAD1E193Q (open) and Δ40-ATAD1E193Q (closed) complexes shown in top and side views. Each subunit (M1–M6) is assigned a distinct color, and the substrate is shown in black. The spiral seam of the open conformation (panel A) is denoted with dashed lines. In the top views, the membrane-facing side of ATAD1 is shown. (C) Schematic of individual domains and structural elements of human ATAD1. (D) Pore-loops 1 (showing those of the closed conformation) form a staircase around the substrate. The peptide density is shown in black mesh. (E) Pore-loops 2 form a second staircase below pore-loops 1. H206s directly contact the peptide backbone carbonyls.
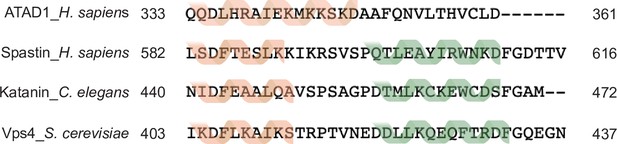
Sequence alignment of AAAMC proteins.
Sequence alignment of AAAMC proteins showing the secondary structure of the C-terminal helices. While ATAD1 has a longer α11, the other members of the AAAMC subfamily have two shorter helices (α11 and α12) separated by a linker. Secondary structure assignment is based on experimentally determined structures: ATAD1 from this study; Spastin from PDB ID 6PEN; Vps4 from PDB ID: 6AP1, and Katanin from PDB ID: 6UGE.
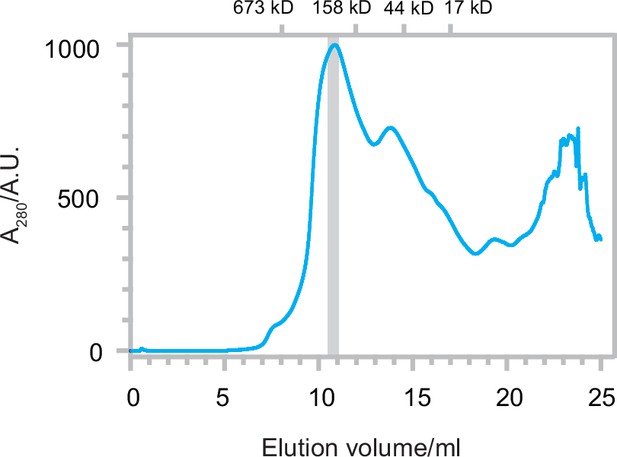
Size exclusion chromatography (SEC) trace of Δ40-ATAD1E193Q.
The SEC trace of Δ40-ATAD1E193Q shows that ATAD1 forms oligomeric species on the SEC column. The gray bar indicated the fraction that was imaged by cryo-EM. Size markers are indicated on the top.
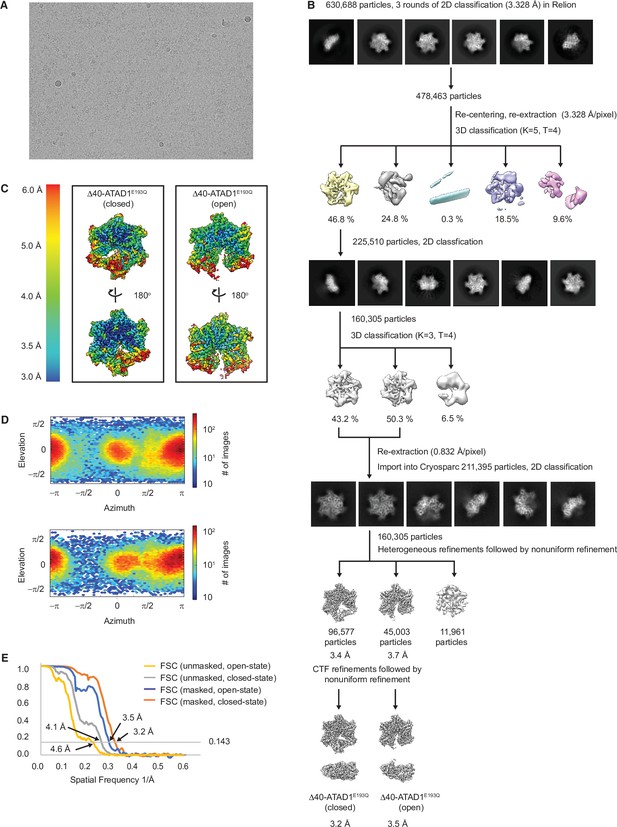
Cryo-EM processing of the Δ40-ATAD1E193Q-substrate complexes.
(A) Representative micrograph showing the quality of data used for the final reconstruction of the Δ40-ATAD1E193Q structures. (B) Data processing scheme showing the 2D and 3D classification done using the RELION software. After two rounds of 3D classification, particles corresponding to ATAD1 are imported into Cryosparc for another round of 2D classification to further purify particles. Particles selected from the 2D classification were then subjected to heterogeneous refinement in which the open and the closed conformations emerged as two distinct classes. CTF refinement followed by nonuniform refinement generated the final reconstruction at 3.2 and 3.5 Å for the closed and the open conformations, respectively. (C) Local resolution maps of the open and the closed conformations show that the core of the protein complex including the central pore and the nucleotide-binding pockets are the best resolved regions. (E) Fourier shell correlation (FSC) plots of the 3D reconstructions of Δ40-ATAD1E193Q: Δ40-ATAD1E193Q (closed) masked (orange), Δ40-ATAD1E193Q (closed) unmasked (gray), Δ40-ATAD1E193Q (open) masked (blue), Δ40-ATAD1E193Q (open) unmasked (yellow).
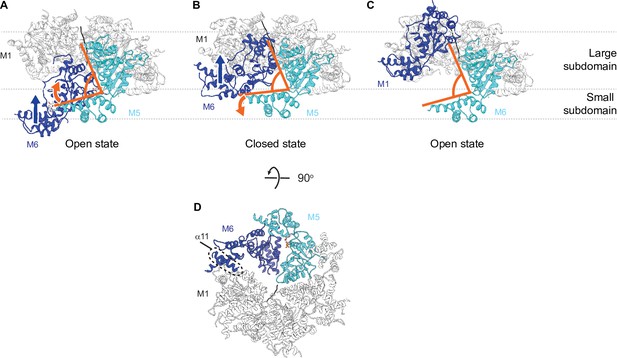
Hinge motion between the large and the small subdomain accompanies subunit movements.
(A) In the open state, all six subunits have similar domain organization. M6 loses its bound nucleotide as it translocates from the bottom position to the closed position. The angle between the large and the small subdomain of M5 narrows (indicated by the orange lines). (B) M6 rebinds an ATP molecule as it continues to translocate upward to assume the top position in the open spiral (C). M5 loses contacts with M6 and the angle between its two subdomains widens. M6 is colored in dark blue, M5 in cyan, the rest of the subunits in white, and the peptide substrate in black. The blue arrow indicates the direction of M6 translocation and the orange arrow indicates the direction of hinge movement between the two subdomains of M5. (D) 90-degree flip from panel (B) showing the contact points between M6 and adjacent subunits. Multiple contact points exist between the M6 and the M5 subunits, whereas fewer contacts are made between the M6 and the M1 subunits. Among those, one is made by helix α11 (highlighted in the dashed circle).
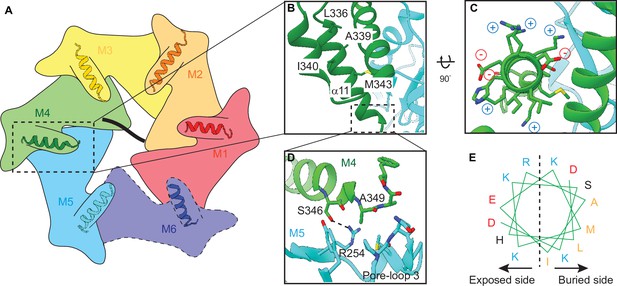
Helix α11 resides at the intersubunit interface of ATAD1.
(A) Schematic showing the position of helix α11 within each ATAD1 subunit. ATAD1 subunits are colored as in Figure 1. α11 is shown in cartoon representation. Different from Figure 1, here, the cytosol-facing side (instead of the membrane-facing side) of ATAD1 is shown, thus the order of subunits from M1, M2 to M6 goes counterclockwise. (B) to (D) zoomed-in view of α11 interaction with the neighboring subunits. (E) Helical wheel showing the amphipathic property of α11. Hydrophobic amino acids are colored in orange, positive charged amino acids in blue, negative charged amino acids in red, and polar amino acids in purple.
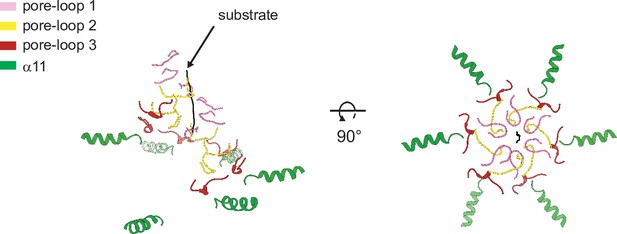
Helices α11 form an additional spiral staircase beneath pore-loop 3.
Cartoon representation of Δ40-ATAD1E193Q (open) showing the multiple staircases formed by three pore-loops and α11 that surround the substrate. Pore-loops 1, 2, and 3 are colored in pink, yellow, and dark red, respectively. Helix α11 is colored in green and the substrate peptide in black.
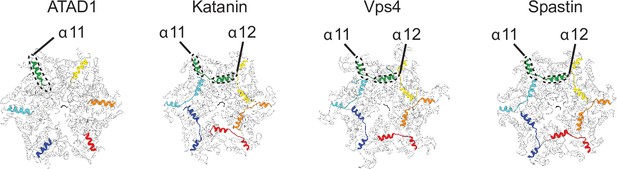
ATAD1 possesses a unique helix at the C-terminus that is structured differently from those in other AAAMC proteins.
Structures of different AAAMC proteins shown in cartoon representation. The cytosol-facing side is shown. The C-terminal helices of each protein subunit (α11 for ATAD1, and α11–α12 for the others) are colored as in Figure 1. The rest of the protein is colored in white. Whereas α11 of ATAD1 contacts the counterclockwise subunit, the α12 of katanin (PDB ID: 6UGE), Vps4 (PDB ID: 6AP1), and spastin (PDB ID: 6PEN) reach across the intersubunit interface and contacts the clockwise subunit.
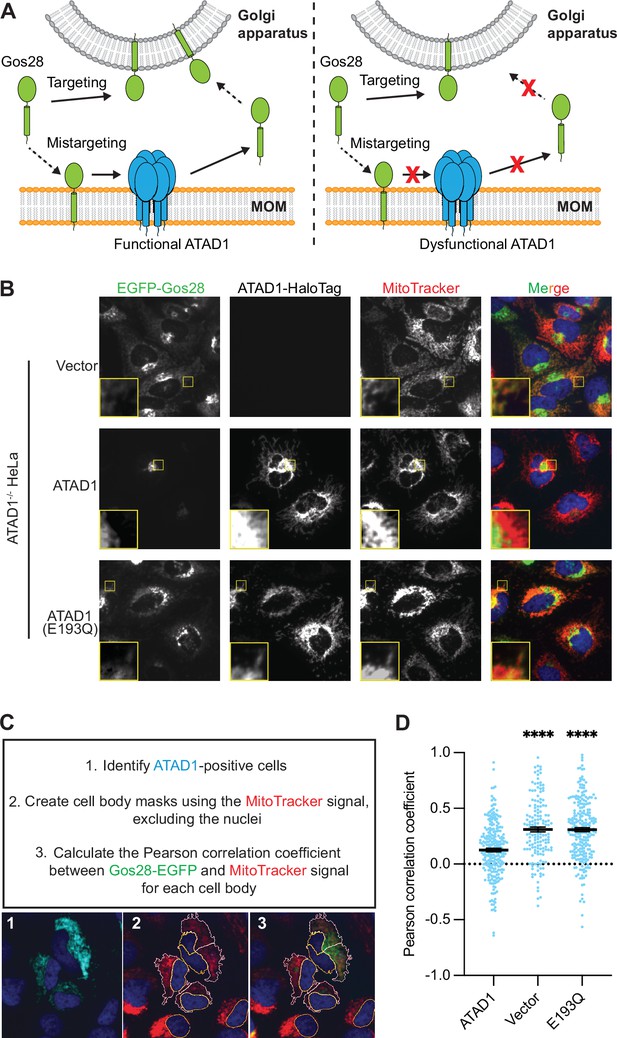
Microscopy assay allows for the direct measurement and quantification of ATAD1’s activity.
(A) Model for ATAD1-depedent extraction of Gos28: in cells expressing a functional ATAD1 (left panel), mislocalized Gos28 on the mitochondrial outer membrane (MOM) is extracted by ATAD1 and given a second chance to return to the Golgi apparatus. In cells expressing a dysfunctional ATAD1 (right panel), Gos28 accumulates on the MOM. (B) Representative average intensity projection images of live HeLa ATAD1-/- cells stably expressing EGFP-Gos28 (green channel) and transiently expressing empty vector (top row), ATAD1-HaloTag (middle row), and ATAD1(E193Q)-HaloTag (bottom row). Mitochondria are stained with MitoTracker (red channel). The individual channels are shown in black and white, and overlay of the EGFP and the MitoTracker channels are shown in the right-most column with Hoechst-stained nuclei in blue. Insets are included to better show the absence or presence of colocalization between EGFP-Gos28 and the mitochondria. (C) Workflow of the CellProfiler pipeline for measuring EGFP-Gos28 mislocalization. (D) Mean Pearson correlation coefficient (PCC) values and the SEM between EGFP-Gos28 and the mitochondria when expressing the indicated construct. Individual cell PCC values are represented as a single dot. Significance values were calculated using the Mann-Whitney test. ****p<0.0001. Figure 3—source data 1 describes the data analysis pipeline and the raw image files used in this pipeline are available on Dryad.
-
Figure 3—source data 1
Data analysis pipeline for live-cell imaging results.
- https://cdn.elifesciences.org/articles/73941/elife-73941-fig3-data1-v2.zip
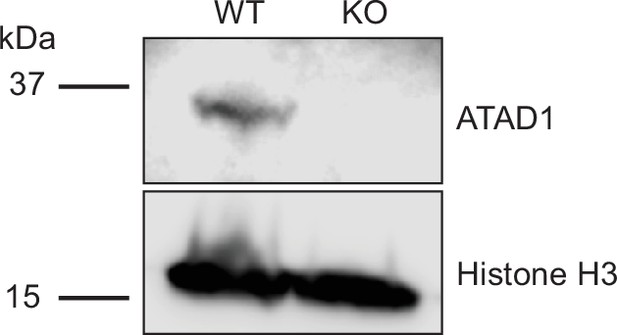
Verification of ATAD1 knockout by Western blot.
Representative western on total protein extracts prepared from wild-type HeLa cells (left lane) and ATAD1-/- HeLa cells (right lane). Knockout of ATAD1 was shown by the absence of ATAD1 signal (41 kDa). Histone H3 (17 kDA) served as the loading control.
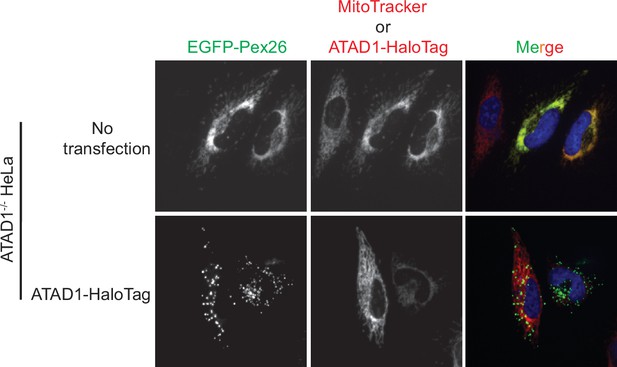
Live-cell imaging showing the ATAD1-dependent localization of EGFP-Pex26.
Representative average intensity projection images of live HeLa ATAD1-/- cells stably expressing EGFP-Pex26 (top row) and transiently expressing ATAD1-HaloTag (bottom row). Mitochondria are stained with MitoTracker red. The individual channels are shown in black and white, and overlay of the EGFP and the MitoTracker red channels are shown in the right-most column with Hoechst-stained nuclei in blue.
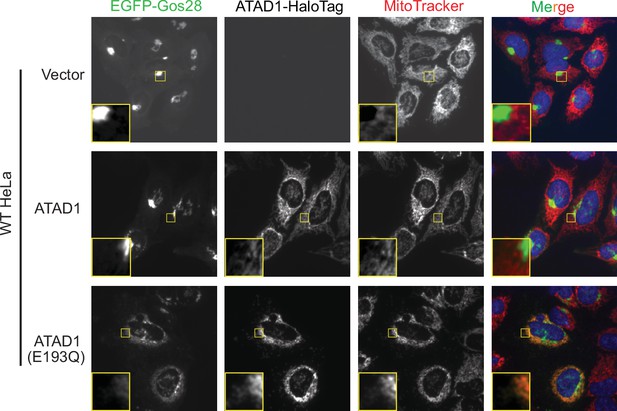
Live-cell imaging showing the ATAD1-dependent localization of EGFP-Gos28 in wild-type (WT) HeLa cells.
Representative average intensity projection images of live WT HeLa cells stably expressing EGFP-Gos28 (green channel) and transiently expressing empty vector (top row), ATAD1-HaloTag (middle row), and ATAD1(E193Q)-HaloTag (bottom row). Mitochondria are stained with MitoTracker (red channel). The individual channels are shown in black and white, and overlay of the EGFP and the MitoTracker channels are shown in the right-most column with Hoechst-stained nuclei in blue. Insets are included to better show the absence or presence of colocalization between EGFP-Gos28 and the mitochondria.
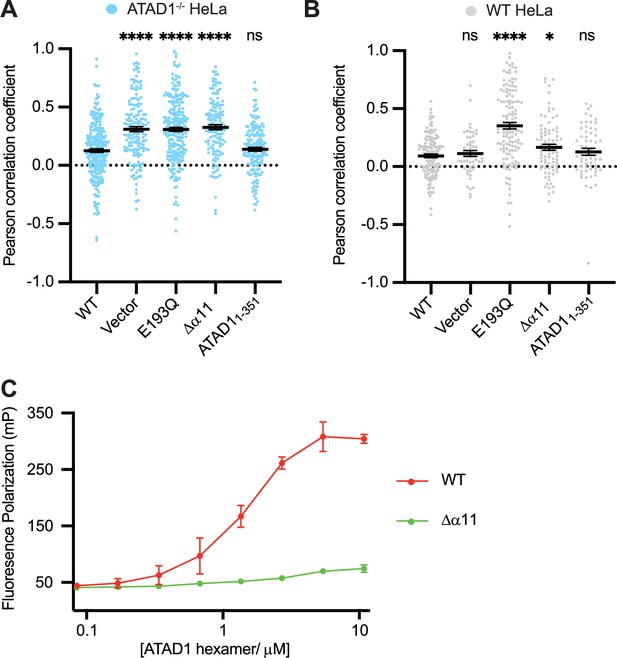
Helix α11 mediates hexamer assembly.
(A) Mean Pearson correlation coefficient (PCC) values and the SEM between EGFP-Gos28 and the mitochondria in ATAD1-/- HeLa cells, transiently expressing controls or the ATAD1Δα11 mutant. (B) Mean PCC values and the SEM between EGFP-Gos28 and the mitochondria in wild-type (WT) HeLa cells, transiently expressing controls or the ATAD1Δα11 mutant. Significance values were calculated using the Mann-Whitney test. *p<0.05, ****p<0.0001. The raw image files used for this analysis are available on Dryad. (C) Fluorescence polarization assay showing the different peptide-binding abilities of the Δ40-ATAD1 (WT) versus Δ40-ATAD1Δα11 (Δα11). ATAD1 concentrations are expressed as hexamer concentrations. Each dot represents an average of two replicates and the error bar represents SEM.
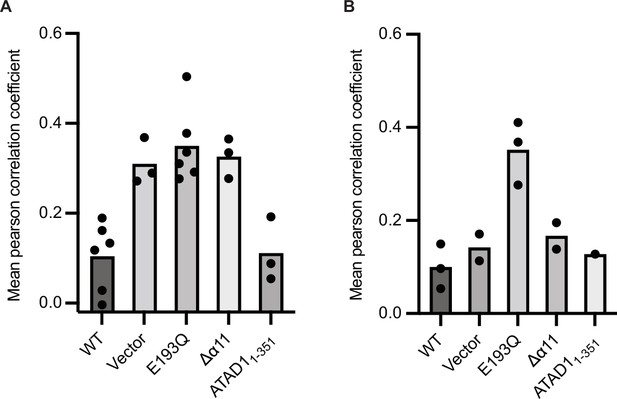
Reproducibility of EGFP-Gos28 mislocalization in live-cell imaging with expression of ATAD1Δα11.
Mean Pearson correlation coefficient (PCC) values derived from the localization pipeline from each biological replicate were plotted for each mutant in (A) ATAD-/- HeLa cells and (B) wild-type (WT) HeLa cells. Each dot represents a biological replicate, with an average of 47 cells per replicate.
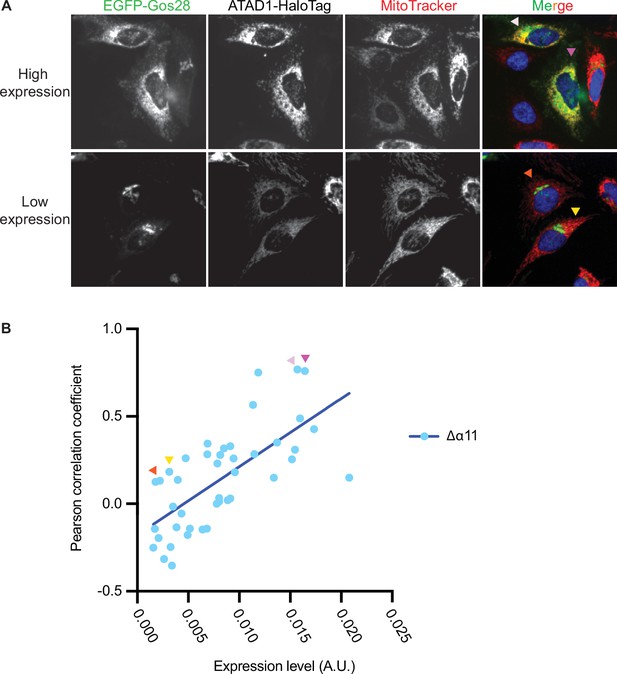
ATAD1Δα11 expression level impacts substrate mislocalization in wild-type (WT) HeLa cells.
(A) Representative average intensity projection images of live WT HeLa cells stably expressing EGFP-Gos28 and transiently expressing ATAD1Δα11-HaloTag. EGFP-Gos28 was only seen on the Golgi apparatus (bottom row). However, a small population of cells (top row) showed mislocalization of EGFP-Gos28 to the mitochondria. These cells also had a higher level of ATAD1Δα11-HaloTag expression (the Pearson correlation coefficient [PCC] of cells shown in the images are indicated in panel B by arrows of matching colors). (B) The expression level of ATAD1Δα11 was plotted against the PCC for each cell. Linear regression analysis was preformed and there was a significant positive correlation between the two variables (p<0.0001).
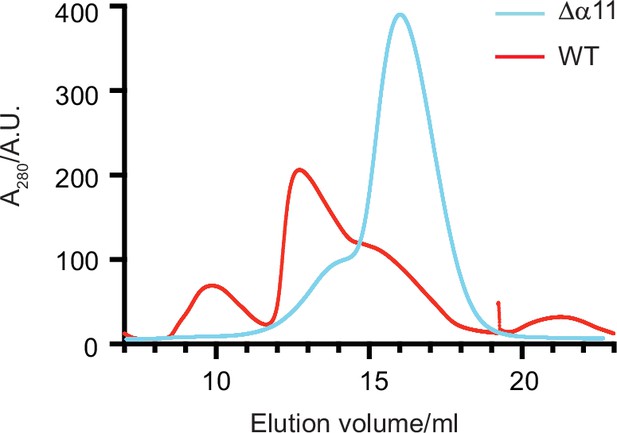
Size exclusion chromatography (SEC) of Δ40-ATAD1 and Δ40-ATAD1Δα11.
The SEC traces of Δ40-ATAD1 (wild-type [WT]) and Δ40-ATAD1Δα11 (Δα11) show that the former forms oligomeric species on the SEC column while the latter is predominantly a monomer. The SEC trace of Δ40-ATAD1 is shown in a red line, and the SEC trace of Δ40-ATAD1Δα11 in a blue curve.
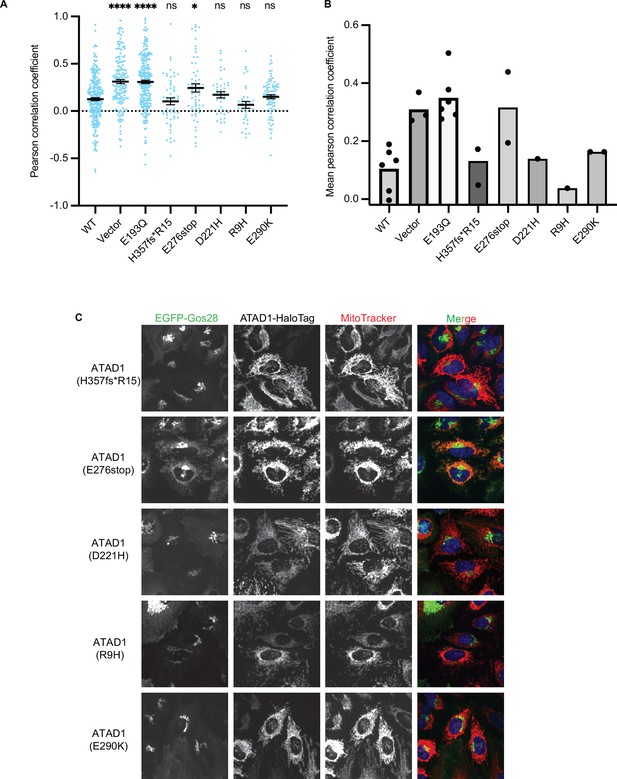
Testing the effect of the disease-relevant mutations of ATAD1.
(A) Mean Pearson correlation coefficient (PCC) values and the SEM between EGFP-Gos28 and the mitochondria when expressing the ATAD1 bearing disease-relevant mutations. Individual cell PCC values are represented as a single dot. Significance values were calculated using the Mann-Whitney test. ****p<0.0001, *p<0.05. The raw image files used for this analysis are available on Dryad. (B) Mean PCC values derived from the localization pipeline from each biological replicate were plotted for each mutant in ATAD-/- HeLa cells. Each dot represents a biological replicate, with an average of 43 cells per replicate. (C) Representative average intensity projection images of live HeLa ATAD1-/- cells stably expressing EGFP-Gos28 and transiently expressing ATAD1 mutants (as indicated on the far-left panel). The individual channels are shown in black and white, and overlay of the EGFP and the MitoTracker channels are shown in the right-most column with Hoechst-stained nuclei in blue.
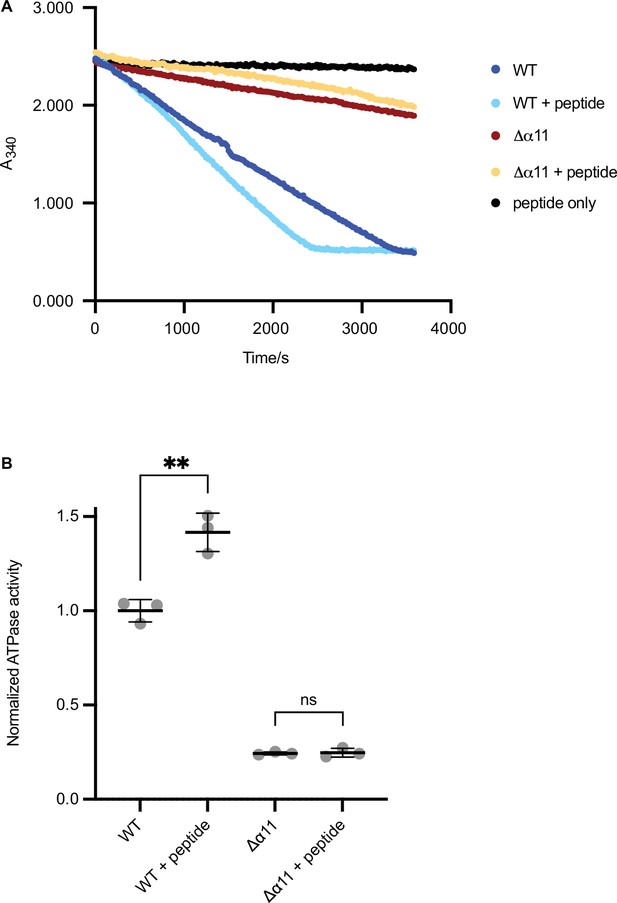
ATPase assay of Δ40-ATAD1 and Δ40-ATAD1Δα11.
(A) Representative ATPase assay showing that the ATPase activity of Δ40-ATAD1 (wild-type [WT]) is stimulated by the substrate (P13) whereas Δ40-ATAD1Δα11 (Δα11) is not. (B) Quantification of panel (A). Each bar represents an average of three replicates and the error bar represents the SD. **p<0.01.
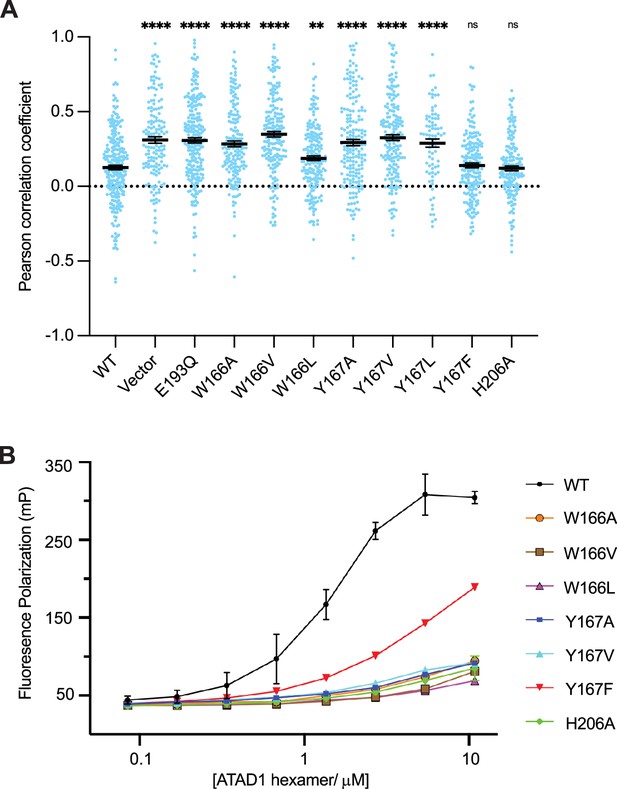
Pore-loop 1 aromatic amino acids are important for ATAD1’s function both in vivo and in vitro.
(A) Mean Pearson correlation coefficient (PCC) values and the SEM between EGFP-Gos28 and the mitochondria in cells expressing ATAD1 variants. Individual cell PCC values are represented as a single dot. Significance values were calculated relative to the wild-type (WT) condition using the Mann-Whitney test. **p<0.01, ****p<0.0001. The raw image files used for this analysis are available on Dryad. (B) Different ATAD1 variants’ peptide-binding abilities as measured by fluorescence polarization. ATAD1 concentrations are expressed as hexamer concentrations. Here, wild-type (WT) refers to Δ40-ATAD1, and each mutant refers to Δ40-ATAD1 bearing that mutation. Each dot represents the average of two replicates, and the error bar represents the SEM.
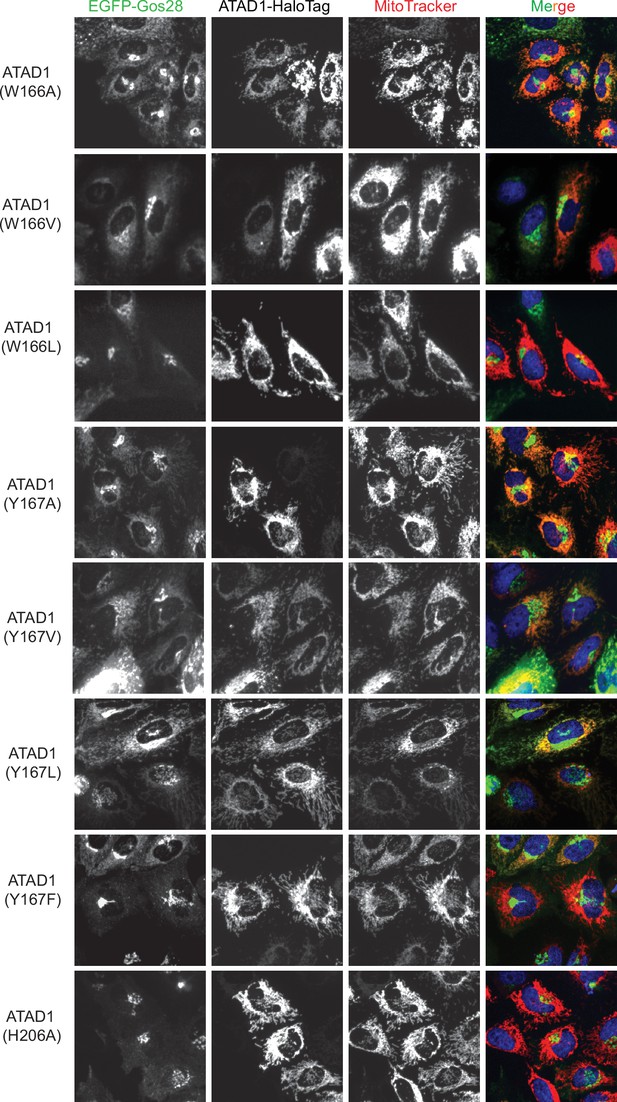
Live-cell imaging showing the pore-loop dependent localization of EGFP-Gos28 in ATAD1-/- HeLa cells.
Representative average intensity projection images of live HeLa ATAD1-/- cells stably expressing EGFP-Gos28 and transiently expressing ATAD1-HaloTag pore-loop mutants (as indicated on the far-left panel). The individual channels are shown in black and white, and overlay of the EGFP and the MitoTracker channels are shown in the right-most column with Hoechst-stained nuclei in blue.
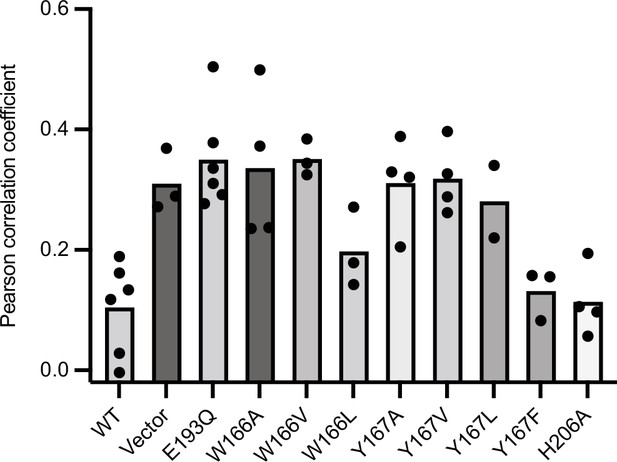
Reproducibility of EGFP-Gos28 mislocalization in live-cell imaging with expression of pore-loop mutants.
Mean Pearson correlation coefficient (PCC) values derived from the localization pipeline from each biological replicate were plotted for each pore-loop mutant in ATAD-/- HeLa cells. Each dot represents a biological replicate, with an average of 50 cells per replicate.

Structure details of the linker domain (LD).
(A) EM map of D40-ATAD1 (open) showing that helix a0 within the LD is well-folded in the central four subunits and disordered in M1 and M6. (B) and (C) showing most of the hydrophobic amino acids within the LD are buried by the well-folded a0 and revealed when a0 becomes disordered. These hydrophobic amino acids make up a plausible substrate binding site. a0 is colored in white and hydrophobic amino acids in gold. Helix a0 is colored in green, hydrophobic amino acids in gold, and the substrate peptide is colored in black.
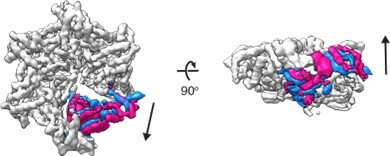
3D variability analysis of D 40-ATAD1E193QDa11 (open) 3D variability analysis was performed in cryoSPARC to capture the movements of the flexible regions of the molecule.
Twenty different frames were used to capture the full trajectory of the movements. Within the twenty frames, the start and the end frames (representing the two extreme states of the movement) are overlayed and shown in the figure. The M6 subunit is colored in blue (start frame) or pink (end frame) and the rest of the protein in light grey.

Quantification of ATAD1 variant expression levels in the WT background.
The average fluorescent intensity for cells expressing ATAD1-HaloTag were plotted for each biological replicate as a proxy for expression levels. ATAD1Da11 is expressed at similar, if not higher, levels when compared to ATAD1E193Q.
Tables
Cryo-EM data analysis.
Structure | Δ40-ATAD1E193Q (closed)PDB ID: 7UPREMD-26674 | Δ40-ATAD1E193Q (open)PDB ID: 7UPTEMD-26675 |
---|---|---|
Data collection | ||
Microscope | Titan Krios | |
Voltage (keV) | 300 | |
Nominal magnification | 105,000× | |
Exposure navigation | Image shift | |
Electron dose (e-/Å2) | 67 | |
Dose rate (e-/pixel/s) | 15 | |
Detector | K3 summit | |
Pixel size (Å) | 0.832 | |
Defocus range (μm) | 0.6–2.0 | |
Micrographs | 6937 | |
Reconstruction | ||
Total extracted particles (no.) | 478,463 | |
Final particles (no.) | 96,577 | 45,003 |
Symmetry imposed | C1 | C1 |
FSC average resolution, masked (Å) | 3.2 | 3.5 |
FSC average resolution, unmasked (Å) | 4.1 | 4.6 |
Applied B-factor (Å) | 121.9 | 119.2 |
Reconstruction package | Cryosparc v2.15 and Relion 3.0.8 | |
Refinement | ||
Protein residues | 1678 | 1694 |
Ligands | 19 | 19 |
RMSD bond lengths (Å) | 0.002 | 0.003 |
RMSD bond angles (°) | 0.571 | 0.802 |
Ramachandran outliers (%) | 0.00 | 0.06 |
Ramachandran allowed (%) | 7.10 | 7.34 |
Ramachandran favored (%) | 92.90 | 92.60 |
Poor rotamers (%) | 4.18 | 11.28 |
CaBLAM outliers (%) | 5.69 | 5.39 |
Molprobity score | 2.33 | 2.79 |
Clash score (all atoms) | 7.82 | 10.14 |
B-factors (protein) | 128.89 | 172.35 |
B-factors (ligands) | 106.10 | 162.46 |
EMRinger score | 1.45 | 1.19 |
Refinement package | Phenix 1.17.1-3660-000 |
Reagent type (species) or resource | Designation | Source or reference | Identifiers | Additional information |
---|---|---|---|---|
Gene (Homo sapiens) | ATAD1 | IDT | For human expression | |
Gene (Homo sapiens) | ATAD1 | IDT | Codon-optimized for E. coli expression | |
Gene (Homo sapiens) | EGFP-GOS28 | TwistBioscience | ||
Gene (Homo sapiens) | EGFP-PEX26 | TwistBioscience | ||
Strain, strain background (Escherichia coli) | BL21(DE3) | Life Technologies | Cat# C601003 | |
Cell line (Homo sapiens) | HeLa | ATCC | ||
Cell line (Homo sapiens) | HeLa, ATAD1KO | Synthego | ||
Antibody | Anti-ATAD1 (Mouse, monoclonal) | NeuroMab | Cat# N125/10 | WB (1:500) |
Antibody | Anti-Histone H3 (Rabbit, polyclonal) | Abcam | Cat# ab1791 | WB (1:1500) |
Recombinant DNA reagent | 6xHis-thrombin-ATAD1E193Q (plasmid) | This paper | Materials and methods section ‘Molecular cloning’ | |
Recombinant DNA reagent | CMVd3-ATAD1-HaloTag | This paper | Materials and methods section ‘Molecular cloning’ | |
Recombinant DNA reagent | CMV-GOS28-EGFP | This paper | Materials and methods section ‘Molecular cloning’ | |
Recombinant DNA reagent | CMV-PEX26-EGFP | This paper | Materials and methods section ‘Molecular cloning’ | |
Peptide, recombinant protein | P13 | GenScript | 5-FAM-FSRLYQLRIR | |
Commercial assay or kit | In-Fusion Snap Assembly Master Mix | Takaro Bio | Cat# 638948 | |
Commercial assay or kit | QuikChange II XL Site-Directed Mutagenesis Kit | Agilent | Cat# 200521 | |
Chemical compound, drug | Janelia Fluor 549 dye | Janelia Fluor Dyes | Gift of Dr Luke Lavis | |
Chemical compound, drug | MitoTracker Deep Red FM | Life Technologies | Cat# M22426 | |
Chemical compound, drug | Hoechst 33342 | Life Technologies | Cat# H1399 | |
Software, algorithm | CellProfiler 4.0.5 | https://doi.org/10.1371/journal.pbio.2005970 | ||
Software, algorithm | MotionCor2 | PMID:28250466 | RRID: SCR_016499 | |
Software, algorithm | Relion | PMID:23000701 | RRID: SCR_016274 | |
Software, algorithm | CryoSPARC | PMID:28165473 | RRID: SCR_016501 | |
Software, algorithm | UCSF Chimera | PMID:15264254 | RRID: SCR_004097 | |
Software, algorithm | GCTF | PMID:26592709 | RRID: SCR_016500 | |
Software, algorithm | Phenix | PMID:20124702 | RRID: SCR_014224 | |
Software, algorithm | Coot | PMID:20383002 | RRID: SCR_014222 |