Fermentation: Teaming up to make kombucha
From cheese to salami, to beer or miso soup, chances are that your favorite delicacy owes its unique flavors to humble communities of microorganisms which ferment sugars into substances that preserve and improve food (Bourdichon et al., 2021). Humans have been enthusiastically brewing or pickling since the Bronze Age, yet surprisingly little is known about the intricacies of the fermentation process (Farag et al., 2019; Yang et al., 2014).
Fermenting food requires dozens if not hundreds of microbial species which work closely together, each producing substances which the others take up, use and transform into new chemicals important for other species in the community (Tamang et al., 2016). These complex interactions make it challenging to disentangle how individual actors contribute to the overall process, and to identify the ones essential for the final product. Now, in eLife, Xiaoning Huang, Yongping Xin and Ting Lu report having methodically reduced the complex microbial system which creates the tangy drink known as kombucha tea, down to a single pair of species (Huang et al., 2022).
Kombucha is created by a thriving community of yeast and bacteria which work together to ferment sugary tea. Huang et al. first focused on the features that this culture must have to produce the famous concoction. Three key characteristics emerged: both yeast and bacteria should be present; a characteristic jelly-like film or ‘pellicle’ should form at the surface; and the culture should consume sucrose while accumulating acetate, ethanol, and small amounts of sucrose constituents such as glucose. Preserving these features ensured that a core community of microbes would capture the essential metabolism of the native culture found in kombucha (Figure 1; Step 1).
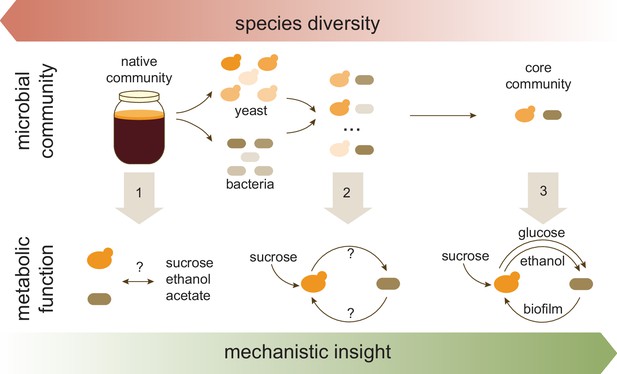
Approach used by Huang et al. to investigate the role of specific members of the microbial community found in kombucha tea.
The species richness of the kombucha microbial community was systematically reduced (top), with each step (gray arrows) improving the understanding of the metabolic function of core species in the culture (bottom). Step 1: Analyzing the native kombucha community revealed the identity and relative abundance of its various microbial members; gross metabolic changes were also recorded (such as consumption of sucrose and production of ethanol and acetate), but they were unassigned to any microorganisms. Step 2: Isolating five yeast and five bacterial species and analyzing their twenty-five pairwise combination cultures revealed that the bacteria depended on yeast degrading sucrose. Step 3: In-depth analysis of a representative yeast-bacteria pair revealed the specific interactions underlying their collaboration (cross-feeding of glucose and ethanol from yeast to bacteria, and bacterial production of biofilm which potentially protects the community).
Next, the team (who are based at the University of Illinois Urbana-Champaign and the China Agricultural University) isolated five yeast and five bacterial species, examining each of them individually or as yeast-bacteria pairs. Some bacteria completely depended on yeast to break down sucrose into glucose and into other essential molecules required for their survival (Figure 1; Step 2). Although all yeast species could survive on their own, the distinctive properties of kombucha (such as its pellicle, high acidity and acetate production) occurred only in co-cultures, indicating that bacteria did contribute to these community functions.
To understand how the community worked at an even finer scale, Huang et al. focused on a single yeast-bacteria pair which could create all three features characteristic of native kombucha. This co-culture was remarkably stable: no matter the ratio of yeast to bacteria at the start of the process, the final communities had roughly equal numbers of each species once stable. They also all produced concoctions which closely resembled traditional kombucha, with similar levels of acidity, sugars, ethanol, and acetate.
Next, these two species were individually cultured on diverse nutrient sources to closely monitor which compounds they could consume and produce (Figure 1; Step 3). The manipulation revealed that only the yeast could make glucose and ethanol; this likely involves the cells secreting an enzyme that processes sucrose into glucose, which is then available for ‘public use’ (Tran et al., 2020; Smith and Schuster, 2019). In turn, the bacteria could only create a pellicle when they consumed glucose and ethanol at the same time. This experiment helped to finally piece together how the two species interact: yeast feed and stimulate bacteria with glucose and ethanol, while bacteria wrap the community in a film that may shield it from the environment (Yin et al., 2019).
If two species alone can thrive and produce kombucha-like tea, then why does this process normally involve many more microorganisms? This taxonomic diversity may improve adaptability (Willi et al., 2006), or it may just emerge through random processes (Sloan et al., 2006); it could even be an artefact due to sampling at an inadequately large scale (Fierer and Lennon, 2011). Further studies are needed to investigate these possibilities.
The reductionist approach developed by Huang et al. allows scientists to pinpoint the core subgroups of microbes which perform the primary functions of a wider community, and to disentangle the role of individual species. This framework is useful to understand the metabolic processes responsible for the signature look, taste and smell of fermented foods. The next steps would potentially involve finetuning the method to study microbial communities which are harder to define, such as those that interact with host organisms or the wider environment.
References
-
The forgotten role of food culturesFEMS Microbiology Letters 368:fnab085.https://doi.org/10.1093/femsle/fnab085
-
The generation and maintenance of diversity in microbial communitiesAmerican Journal of Botany 98:439–448.https://doi.org/10.3732/ajb.1000498
-
Quantifying the roles of immigration and chance in shaping prokaryote community structureEnvironmental Microbiology 8:732–740.https://doi.org/10.1111/j.1462-2920.2005.00956.x
-
Public goods and cheating in microbesCurrent Biology 29:R442–R447.https://doi.org/10.1016/j.cub.2019.03.001
-
Review: Diversity of microorganisms in global fermented foods and beveragesFrontiers in Microbiology 7:377.https://doi.org/10.3389/fmicb.2016.00377
-
Limits to the adaptive potential of small populationsAnnual Review of Ecology, Evolution, and Systematics 37:433–458.https://doi.org/10.1146/annurev.ecolsys.37.091305.110145
-
Proteomics evidence for kefir dairy in early Bronze Age ChinaJournal of Archaeological Science 45:178–186.https://doi.org/10.1016/j.jas.2014.02.005
-
Biofilms: the microbial “protective clothing” in extreme environmentsInternational Journal of Molecular Sciences 20:E3423.https://doi.org/10.3390/ijms20143423
Article and author information
Author details
Publication history
Copyright
© 2022, Ponomarova
This article is distributed under the terms of the Creative Commons Attribution License, which permits unrestricted use and redistribution provided that the original author and source are credited.
Metrics
-
- 2,381
- views
-
- 168
- downloads
-
- 0
- citations
Views, downloads and citations are aggregated across all versions of this paper published by eLife.
Download links
Downloads (link to download the article as PDF)
Open citations (links to open the citations from this article in various online reference manager services)
Cite this article (links to download the citations from this article in formats compatible with various reference manager tools)
Further reading
-
- Computational and Systems Biology
- Developmental Biology
Understanding the principles underlying the design of robust, yet flexible patterning systems is a key problem in developmental biology. In the Drosophila wing, Hedgehog (Hh) signaling determines patterning outputs using dynamical properties of the Hh gradient. In particular, the pattern of collier (col) is established by the steady-state Hh gradient, whereas the pattern of decapentaplegic (dpp), is established by a transient gradient of Hh known as the Hh overshoot. Here, we use mathematical modeling to suggest that this dynamical interpretation of the Hh gradient results in specific robustness and precision properties. For instance, the location of the anterior border of col, which is subject to self-enhanced ligand degradation is more robustly specified than that of dpp to changes in morphogen dosage, and we provide experimental evidence of this prediction. However, the anterior border of dpp expression pattern, which is established by the overshoot gradient is much more precise to what would be expected by the steady-state gradient. Therefore, the dynamical interpretation of Hh signaling offers tradeoffs between robustness and precision to establish tunable patterning properties in a target-specific manner.
-
- Computational and Systems Biology
- Neuroscience
Hypothalamic kisspeptin (Kiss1) neurons are vital for pubertal development and reproduction. Arcuate nucleus Kiss1 (Kiss1ARH) neurons are responsible for the pulsatile release of gonadotropin-releasing hormone (GnRH). In females, the behavior of Kiss1ARH neurons, expressing Kiss1, neurokinin B (NKB), and dynorphin (Dyn), varies throughout the ovarian cycle. Studies indicate that 17β-estradiol (E2) reduces peptide expression but increases Slc17a6 (Vglut2) mRNA and glutamate neurotransmission in these neurons, suggesting a shift from peptidergic to glutamatergic signaling. To investigate this shift, we combined transcriptomics, electrophysiology, and mathematical modeling. Our results demonstrate that E2 treatment upregulates the mRNA expression of voltage-activated calcium channels, elevating the whole-cell calcium current that contributes to high-frequency burst firing. Additionally, E2 treatment decreased the mRNA levels of canonical transient receptor potential (TPRC) 5 and G protein-coupled K+ (GIRK) channels. When Trpc5 channels in Kiss1ARH neurons were deleted using CRISPR/SaCas9, the slow excitatory postsynaptic potential was eliminated. Our data enabled us to formulate a biophysically realistic mathematical model of Kiss1ARH neurons, suggesting that E2 modifies ionic conductances in these neurons, enabling the transition from high-frequency synchronous firing through NKB-driven activation of TRPC5 channels to a short bursting mode facilitating glutamate release. In a low E2 milieu, synchronous firing of Kiss1ARH neurons drives pulsatile release of GnRH, while the transition to burst firing with high, preovulatory levels of E2 would facilitate the GnRH surge through its glutamatergic synaptic connection to preoptic Kiss1 neurons.