Arpin deficiency increases actomyosin contractility and vascular permeability
Figures
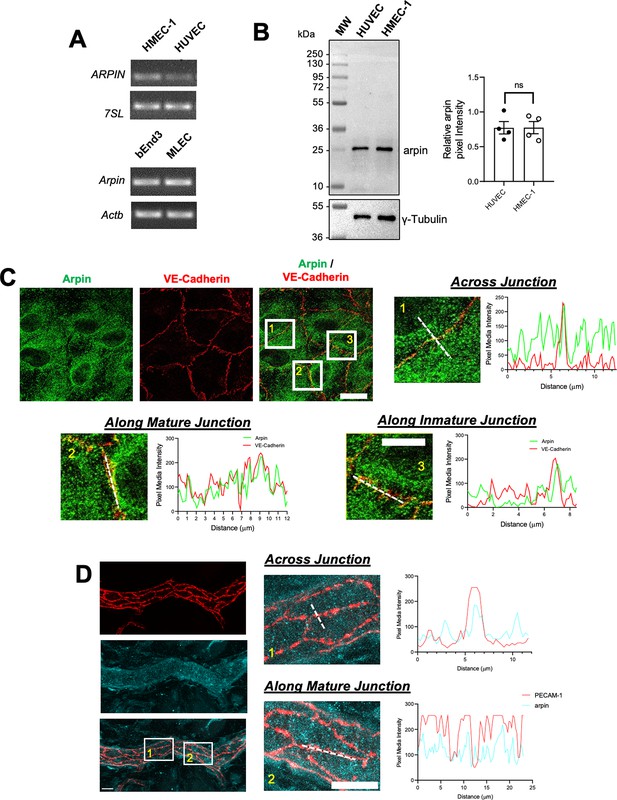
Arpin is expressed in endothelial cells.
(A) End-point RT-PCR for ARPIN in the human microvascular endothelial cell line (HMEC-1) and human umbilical vein endothelial cells (HUVEC), 7SL was used as housekeeping gene (top); and Arpin in the mouse brain endothelial cell line bEnd.3 and primary murine lung endothelial cells (MLEC); Actb was used as a housekeeping gene (bottom). A representative gel of three independent experiments is shown. (B) Representative western blot for arpin in HUVEC and HMEC-1. MW = molecular weight bands. The graph shows the quantification of the relative pixel intensity of the arpin band normalized to γ-tubulin as a loading control (n=4). Data are represented as mean ± standard error of the mean (SEM); ns: non-significant; two-tailed student’s t-test. (C) Representative immunostaining of arpin and vascular endothelial (VE)-Cadherin in HUVEC to analyze arpin localization at cell-cell junctions (40 X objective; scale bar = 20 μm). Magnified views of boxed regions are shown (4.3 digital zooms; scale bar = 5 μm). The graphs show the dashed line scans performed with ImageJ software along the white lines in the magnified images. Profiles of the pixel intensity mean of arpin and VE-Cadherin across junctions (1), along mature junctions (2), and along immature junctions (3) are depicted. 25 images each were analyzed from three independent experiments. (D) Representative immunostaining of arpin and PECAM-1 in postcapillary venules of mouse cremaster muscles to analyze arpin localization at cell-cell junctions in vivo (40 X objective; scale bar = 20 μm). Magnified views of dashed boxed regions are shown (3.3 X digital zoom; scale bar = 10 μm). The graphs show the line scans performed with ImageJ software along the white lines in the magnified images. Profiles of the pixel intensity mean of arpin and PECAM-1 across junctions (1), and along mature junctions (2) are depicted. 15 venules each were analyzed from four mice.
-
Figure 1—source data 1
Uncropped and labeled gels and membranes for Figure 1.
- https://cdn.elifesciences.org/articles/90692/elife-90692-fig1-data1-v1.zip
-
Figure 1—source data 2
Raw unedited membranes and gels for Figure 1.
- https://cdn.elifesciences.org/articles/90692/elife-90692-fig1-data2-v1.zip
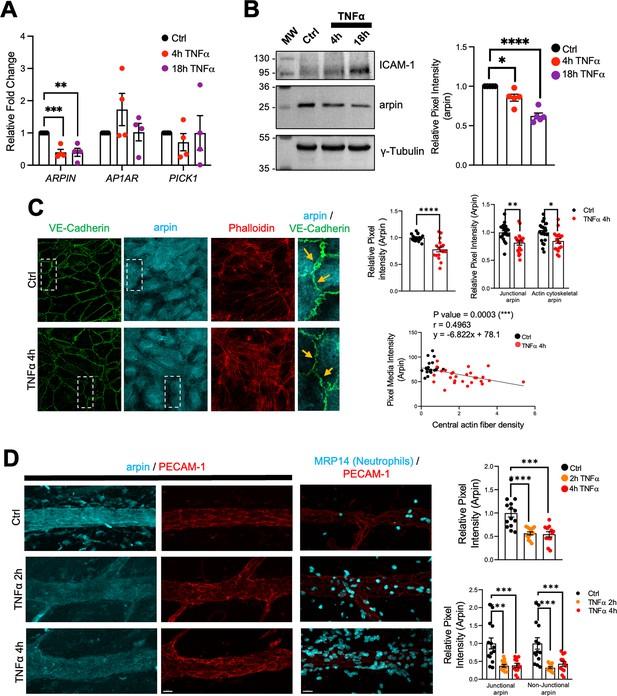
Arpin is downregulated by TNFα.
(A) qRT-PCR for the Arp2/3 complex inhibitors ARPIN, AP1AR, and PICK1 using cDNA from human umbilical vein endothelial cells (HUVEC) treated or not with TNFα for the indicated times (n=4). Data are shown as relative expression (fold change) normalized to the housekeeping gene 7SL. (B) Representative western blot for arpin in HUVEC treated or not with TNFα for the indicated times (n=5). MW = molecular weight bands. ICAM-1 was used as a positive control of the induction of inflammation. The graph shows the mean pixel intensity of arpin bands normalized to tubulin as loading control and to the control condition (set to 1). (C) Representative immunostaining of arpin and vascular endothelial (VE)-Cadherin together with phalloidin F-actin staining in HUVEC treated or not with TNFα for 4 hr (40 X Objective, scale bar = 20 μm). Magnifications of the right show with the orange arrows loss of arpin at junctions with TNFα treatment. The graphs show arpin pixel intensity quantification after treatment normalized to the average of ctrl HUVECs: top-left, total arpin in 17 images each was analyzed from four independent experiments; top-right, junctional, and actin cytoskeletal arpin in 19 images each were analyzed from three independent experiments; and bottom graph: Pearson’s correlation analysis showing that arpin is inversely correlated to central actin fiber density (Pearson’s correlation coefficient, r; 49 cells were analyzed from three independent experiments). (D) Representative immunostaining for arpin (blue) and PECAM-1 (red) in postcapillary venules treated or not with TNFα at the indicated times. PECAM-1 and MRP14 (neutrophils) stainings (right) are shown as positive controls for the induction of inflammation (40 x objective, scale bar = 20 μm). The top graph shows total arpin pixel intensity quantification after TNFα treatment normalized to the average of control venules (12–14 venules were analyzed from four mice in each group). The bottom graph shows junctional and non-junctional arpin quantification after TNFα treatment normalized to the average of control venules (12–14 venules were analyzed from four mice in each group). All data are represented as mean ± SEM; *p<0.05; **p<0.01; ***p<0.001; ****p<0.0001; two-tailed student’s t-test.
-
Figure 2—source data 1
Uncropped and labeled membranes for Figure 2.
- https://cdn.elifesciences.org/articles/90692/elife-90692-fig2-data1-v1.zip
-
Figure 2—source data 2
Raw unedited membranes for Figure 2.
- https://cdn.elifesciences.org/articles/90692/elife-90692-fig2-data2-v1.zip

Arpin is reduced by IL-1β.
(A) Representative western blot for PICK1 in human umbilical vein endothelial cells (HUVEC) treated or not with TNFα for the indicated times (n=3). The graph shows the mean pixel intensity of PICK1 bands normalized to tubulin as a loading control and to the control condition (set to 1). (B) Representative Western blot for arpin HUVECs treated or not with IL-1β for the indicated times. ICAM-1 was used as a positive control of the induction of inflammation. The graph shows the quantification of the relative pixel intensity of arpin bands normalized to the untreated control (set to 1) and γ-tubulin as a loading control (n=4). (C) Representative western blot for PICK1 in HUVEC treated or not with IL-1β for the indicated times (n=3). The graph shows the mean pixel intensity of PICK1 bands normalized to tubulin as a loading control and to the control condition (set to 1). (D) Representative immunostaining of vascular endothelial (VE)-Cadherin and arpin with F-actin staining using phalloidin in HUVEC treated or not with IL-1β (Scale bar = 20 μm). The upper left graph shows total arpin pixel intensity quantification normalized to the average of control HUVEC (top-left; n=11 in three independent experiments). The upper right graph shows junctional and actin cytoskeletal arpin quantification in control and IL-1β treatment HUVEC (n=11–15 in each group in three independent experiments). The graph at the bottom shows that arpin is inversely correlated to central actin fiber density (Pearson’s correlation coefficient, r, n=45 cells). All data are represented as mean ± SEM; ns: non-significant; *p<0.05; **p<0.01; ***p<0.001; two-tailed student’s t-test.
-
Figure 2—figure supplement 1—source data 1
Uncropped and labeled membranes for Figure 2—figure supplement 1.
- https://cdn.elifesciences.org/articles/90692/elife-90692-fig2-figsupp1-data1-v1.zip
-
Figure 2—figure supplement 1—source data 2
Raw unedited membranes for Figure 2—figure supplement 1.
- https://cdn.elifesciences.org/articles/90692/elife-90692-fig2-figsupp1-data2-v1.zip
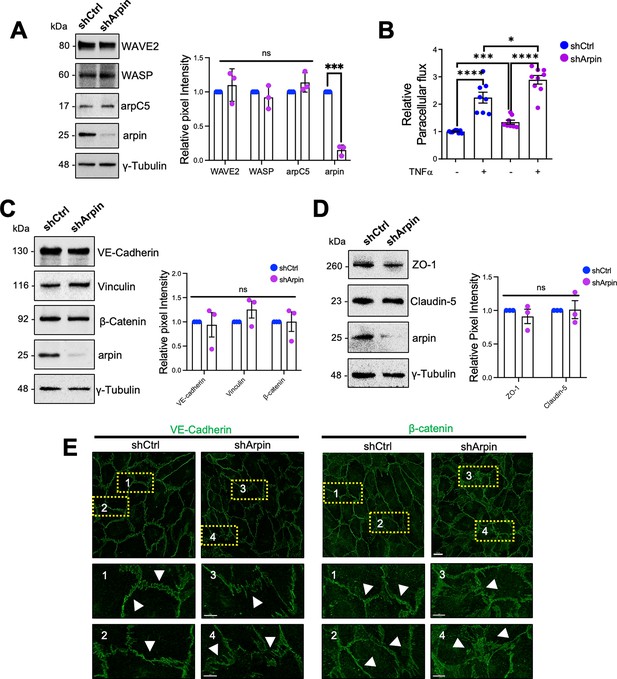
Arpin depletion induces hyperpermeability and irregular junction patterns.
(A) Representative Western blots for WASP family Verprolin homolog 2 (WAVE2), WASP, the Arp2/3 subunit ArpC5, and arpin in lysates of control (shCtrl) and arpin-depleted (shArpin) human umbilical vein endothelial cells (HUVEC). The graph shows the mean pixel intensities of the protein bands normalized to the respective control bands. All bands were normalized to γ-tubulin as loading control (n=3). (B) Paracellular flux of 150 kDa FITC-dextran across confluent control and arpin-depleted HUVEC monolayers cultured on transwell filters (0.4 μm pore size) untreated or treated with TNFα for 18 hr. Data are represented as relative permeability normalized to control HUVEC set to 1 (n=9 in three independent experiments). (C) Representative Western blots for vascular endothelial (VE)-Cadherin, β-catenin, vinculin, and arpin in lysates of control and arpin-depleted HUVEC. The graph shows the mean pixel intensities of the protein bands normalized to the respective control bands (set to 1). All bands were normalized to γ-tubulin as a loading control (n=3). (D) Representative Western blots for ZO-1, claudin-5, and arpin in lysates of control and arpin-depleted HUVEC. γ-Tubulin was used as a loading control. The graph shows the quantification of the relative pixel intensity of ZO-1 and claudin-5 bands normalized to the untreated control (set to 1) and γ-tubulin (n=3). (E) Immunostaining of VE-Cadherin and β-catenin in control and arpin-depleted HUVEC (40 X objective, scale bar = 20 μm; dashed boxes indicate magnified areas that highlight changes in junctional architecture; 3.3 digital zoom, scale bars = 5 μm). Representative images of four independent experiments are shown. Arrows depict linear and mature junctions in shctrl HUVEC and interrupted and gap junction formation and junction internalization in shArpin HUVEC. All data are represented as mean ± SEM; ns: non-significant; *p<0.05; ***p<0.001; ****p<0.0001; two-tailed student’s t-test.
-
Figure 3—source data 1
Uncropped and labeled membranes for Figure 3.
- https://cdn.elifesciences.org/articles/90692/elife-90692-fig3-data1-v1.zip
-
Figure 3—source data 2
Raw unedited membranes for Figure 3.
- https://cdn.elifesciences.org/articles/90692/elife-90692-fig3-data2-v1.zip

Arpin does not participate in junction assembly.
(A) Representative immunostaining of VE-Cadherin in control and arpin-depleted human umbilical vein endothelial cells (HUVEC) in calcium-switch assays over time (63 X objective; scale Bar = 20 μm). (B) The graph shows the quantification of junctional length, calculated in imageJ software, per the number of cells (n=3). All data are represented as mean ± SEM; *p<0.05; **p<0.01; ****p<0.0001; two-way ANOVA.
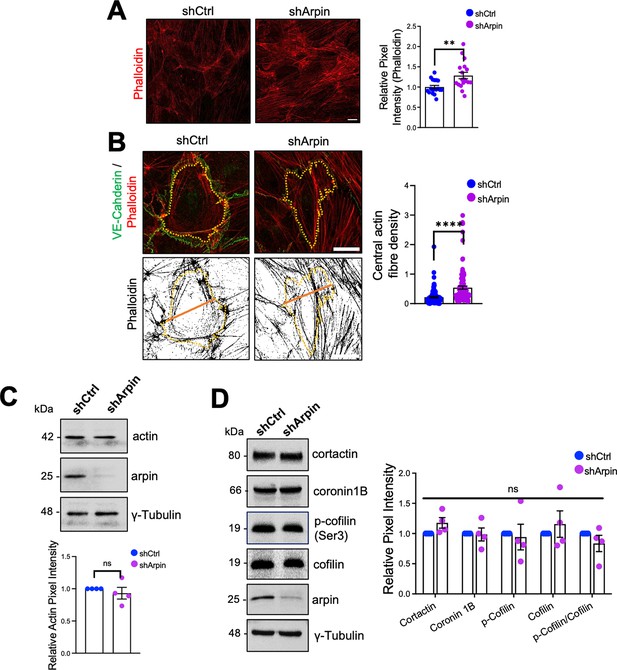
Arpin depletion induces actin filament formation.
(A) Representative F-actin staining using phalloidin of control and arpin-depleted human umbilical vein endothelial cells (HUVEC) (40 X objective, scale bar = 20 μm). The graph shows phalloidin pixel intensity quantification normalized to the average of control HUVEC. 18 images were analyzed in each group from four independent experiments. (B) Representative immunostaining of vascular endothelial (VE)-Cadherin with F-actin staining using phalloidin (top) in control and arpin-depleted HUVEC. The method of central actin fiber quantification using the phalloidin signal is depicted on the bottom as reported before (García Ponce et al., 2016) (63 X objective, scale bar = 5 μm). Yellow dashed lines delineate an individual cell. The graph shows the mean central actin fiber density quantification along the orange lines. 109 cells were analyzed in each group from three independent experiments. (C) Representative Western blot for total actin in lysates of control and arpin-depleted HUVEC. The graph shows the mean pixel intensity of the actin bands normalized to the control and to γ-tubulin as a loading control (n=4). (D) Representative Western blots for cortactin, coronin1B, p-cofilin, cofilin, and arpin in lysates of control and arpin-depleted HUVEC. The graph shows the mean pixel intensity of the protein bands normalized to the respective control bands (set to 1). All bands were normalized to γ-tubulin as a loading control (n=4). All data are represented as mean ± SEM; ns: non-significant; **p<0.01; ****p<0.0001; two-tailed student’s t-test.
-
Figure 4—source data 1
Uncropped and labeled membranes for Figure 4.
- https://cdn.elifesciences.org/articles/90692/elife-90692-fig4-data1-v1.zip
-
Figure 4—source data 2
Raw unedited membranes for Figure 4.
- https://cdn.elifesciences.org/articles/90692/elife-90692-fig4-data2-v1.zip
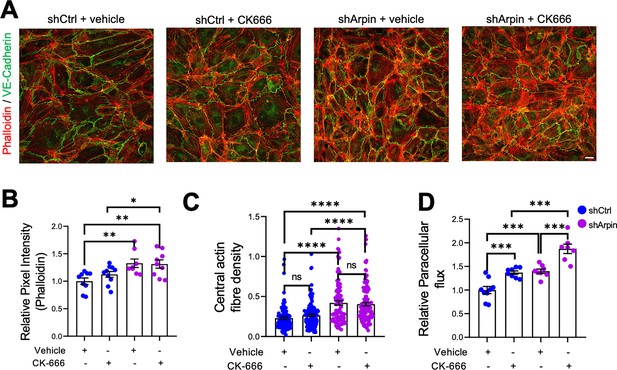
Inhibition of the Arp2/3 complex in arpin-depleted human umbilical vein endothelial cells (HUVEC) does not rescue hyperpermeability and actin cytoskeleton alterations.
(A) Immunostaining of vascular endothelial (VE)-Cadherin with F-actin staining using phalloidin in control and arpin-depleted HUVEC treated with 100 μM of the Arp2/3 inhibitor CK-666 or vehicle (40 X objective, scale bar = 20 μm). Representative images of three independent experiments are shown. (B) Total phalloidin pixel intensity quantification normalized to the average of control HUVEC. Nine images were analyzed in each group from three independent experiments. (C) Central actin fiber density quantification done as described in Figure 4B. 100 cells were analyzed in each group from three independent experiments. (D) Paracellular flux assays using confluent control and arpin-depleted HUVEC monolayers on 0.4 µm pore transwell filters treated with 100 μM CK-666 or vehicle (n=7–9 from three independent experiments). Data are normalized to control HUVEC treated with the vehicle set to 1. All data are represented as mean ± SEM; ns: non-significant; *p<0.05; **p<0.01; ***p<0.001; ****p<0.0001; two-tailed student’s t-test.
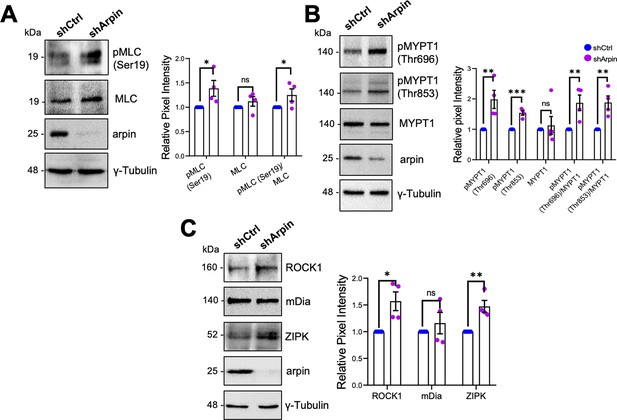
Arpin depletion increases the formation of actin stress fibers.
(A) Representative Western blots for pMLC (Ser19), myosin light chain (MLC), and arpin in lysates of control and arpin-depleted human umbilical vein endothelial cells (HUVEC). The graph shows the mean pixel intensity of the protein bands normalized to the respective control band (set to 1). All bands were normalized to γ-tubulin as a loading control (n=4). (B) Representative Western blots for pMYPT1 (Thr696), pMYPT1 (Thr853), MYPT1, and arpin in lysates of control and arpin-depleted HUVEC. The graph shows the mean pixel intensity of the protein bands normalized to the respective control band (set to 1). All bands were normalized to γ-tubulin as a loading control (n=4). (C) Representative Western blots for Rho-associated protein kinase 1 (ROCK1), mDia, Zipper interacting protein kinase (ZIPK), and arpin in lysates of control and arpin-depleted HUVEC. The graph shows the mean pixel intensity of the protein bands normalized to the respective control band (set to 1). All bands were normalized to γ-tubulin as a loading control (n=4). All data are represented as mean ± SEM; ns: non-significant; *p<0.05; **p<0.01; ***p<0.001; two-tailed student’s t-test.
-
Figure 6—source data 1
Uncropped and labeled membranes for Figure 6.
- https://cdn.elifesciences.org/articles/90692/elife-90692-fig6-data1-v1.zip
-
Figure 6—source data 2
Raw unedited membranes for Figure 6.
- https://cdn.elifesciences.org/articles/90692/elife-90692-fig6-data2-v1.zip
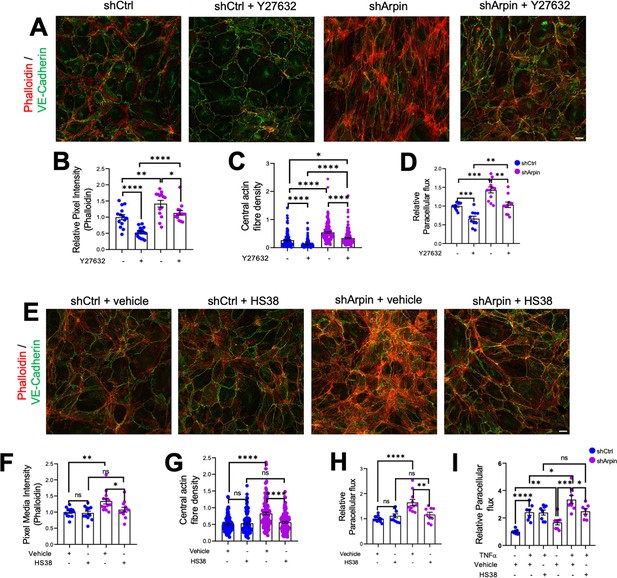
ROCK1/2 and Zipper interacting protein kinase (ZIPK) inhibition partially rescues the increase in stress fibers and permeability in arpin-depleted human umbilical vein endothelial cells (HUVEC).
(A) Representative immunostaining of vascular endothelial (VE)-Cadherin with F-actin staining using phalloidin in control and arpin-depleted HUVEC treated or not with 10 μM of the ROCK1/2 inhibitor Y27632 (40 X objective, scale bar = 20 μm). (B) Phalloidin pixel intensity quantification normalized to the average of control HUVEC. 14 images were analyzed in each group from three independent experiments. (C) Central actin fiber density quantification of at least 140 cells in each group from three independent experiments. (D) Paracellular flux assays using confluent control and arpin-depleted HUVEC monolayers on 0.4 µm pore transwell filters treated with 10 μM Y27632 or vehicle (n=10–12 from four independent experiments). (E) Representative immunostaining of VE-Cadherin with F-actin staining using phalloidin in control and arpin-depleted HUVEC treated with 10 μM of the ZIPK inhibitor HS38 or vehicle (40 x objective; scale bar = 20 μm). (F) Phalloidin pixel intensity quantification normalized to the average of control HUVEC treated with the vehicle. 13 images were analyzed in each group from three independent experiments. (G) Central actin fiber density quantification of at least 125 cells in each group from three independent experiments. (H–I) Paracellular flux assays using confluent control and arpin-depleted HUVEC monolayers on 0.4 µm pore transwell filters treated with (H) 10 μM HS38 or vehicle (n=9–12 in four independent experiments) or (I) treated or not with 15 ng/mL TNFα and with 10 μM HS38 or vehicle (7–8 in three independent experiments). All immunofluorescences are representative of three independent experiments. All data are represented as mean ± SEM; ns: non-significant; *p<0.05; **p<0.01; ***p<0.001; ****p<0.0001; two-tailed student’s t-test.
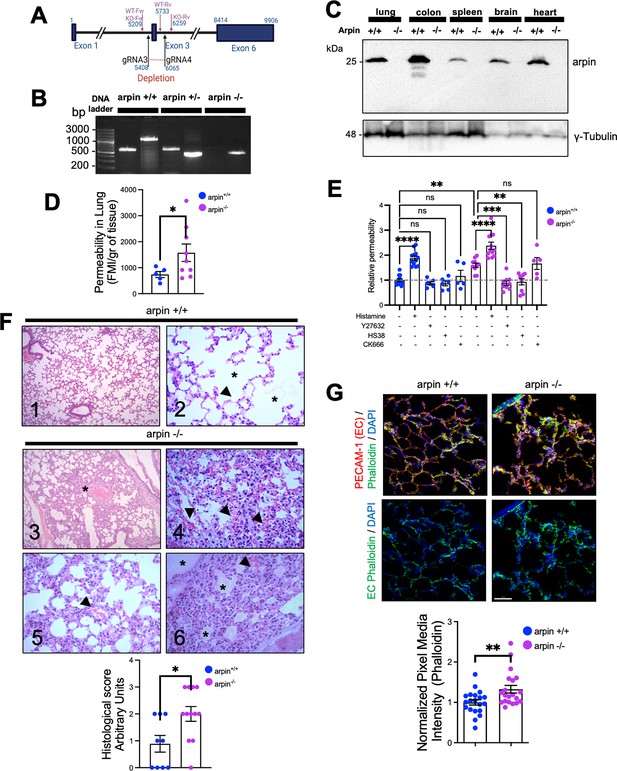
Arpin-deficient mice are viable but show increased vascular permeability in the lung and skin.
(A) Arpin mouse gene showing exon 3, which was deleted using the CRISP/Cas9 technology to generate the complete Arpin-/- mouse model. (B) Representative genotypification PCR of mice carrying the wild-type (WT) (Arpin+/+), heterozygous (Arpin+/-), and deleted (KO, Arpin -/-) alleles. (C) Representative Western blots for arpin in lysates of the indicated organ tissues from Arpin+/+ and Arpin-/- mice (n=3). (D) Permeability assays in the lungs. 150 kDa FITC-dextran was injected into Arpin+/+ and Arpin-/- mice via the cannulated artery carotid. Animals were sacrificed, and perfused, and lungs were collected and homogenized in PBS. The homogenized tissue containing the leaked FITC-dextran was quantified using a fluorometer. (five Arpin+/+ and eight Arpin-/- mice were analyzed). Data are represented as mean ± SEM; *p<0.05; two-tailed student’s t-test with Welch’s correction. (E) Miles assays to determine vascular permeability in the back skin of Arpin+/+ and Arpin-/- mice under basal, inflammatory conditions with histamine, 10 μM of ROCK1/2 inhibitor Y27632, 10 μM of ZIPK inhibitor HS38 and 100 μM or Arp2/3 complex inhibitor CK-666. Data are shown as relative permeability with untreated Arpin+/+ group set to 1 (n=at least of 7 mice in each group) and are represented as mean ± SEM. ns: non-significant; *p<0.05; **p<0.01; ***p<0.001; ****p<0.0001; two-tailed student’s t-test. (F) Representative images of the hematoxylin and eosin staining of lung tissues of Arpin+/+ (Images 1 and 2) and Arpin-/- (Images 3–6) mice. Image 1 shows normal histology (10 X objective). Image 2 shows the normal structure of the alveoli (*) and the blood vessels (arrowheads) without any pathology (40 X objective). Image 3 shows some areas with alveolar volume reduced (*) in Arpin-/- mice (10 X objective). Image 4 shows discrete interalveolar hemorrhages (arrowheads, 40 X objective). Image 5 shows congestion and dilatation of the capillaries (arrowheads, 40 X objective). Image 6 shows interalveolar edema (*) and microhemorrhage (arrowhead, 40 X objective). The histological score of the lung tissue is shown in the graph below. A score of 0 indicates no inflammation; 1 is low inflammation; 2 is moderate inflammation; 3 is high inflammation (9 images from Arpin+/+ and 12 images from Arpin-/- mice were analyzed). Data are represented as mean ± SEM; *p<0.05; two-tailed student’s t-test. (G) Representative immunostaining for PECAM-1 and F-actin staining using phalloidin in cryosections from lungs of Arpin+/+ and Arpin-/- mice. Images in the bottom show endothelial cell (EC) F-actin extracted using PECAM-1 as a templete of ECs using Imaris software (40 x objective, scale bar = 50 μm). Graph shows mean F-actin pixel intensity quantification in arpin-/- lungs normalized to the average of images from Arpin+/+ lungs (20 images were analyzed from three mice in each group). Data are represented as mean ± SEM; **p<0.01; two-tailed student’s t-test.
-
Figure 8—source data 1
Uncropped and labeled membranes for Figure 8.
- https://cdn.elifesciences.org/articles/90692/elife-90692-fig8-data1-v1.zip
-
Figure 8—source data 2
Raw unedited membranes for Figure 8.
- https://cdn.elifesciences.org/articles/90692/elife-90692-fig8-data2-v1.zip

Arpin deficiency increases vascular permeability in the skin and alters venular junction architecture in the cremaster muscle.
(A) The graph shows the average weight of Arpin+/+ and Arpin -/- mice over time (at least 3 Arpin+/+ and Arpin -/- mice were weighted at each time point). (B) Representative Western blots for the indicated proteins in lysates of lung tissue from Arpin+/+ and Arpin -/- mice. The graph shows the quantification of the relative pixel intensity of the protein bands normalized to γ-tubulin as a loading control (n=3). Data are represented as mean ± SEM; ns: non-significant; two-tailed student’s t-test. (C) Representative immunostaining for arpin and vascular endothelial (VE)-Cadherin in postcapillary venules of Arpin+/+ and Arpin -/- mice. (n=3; dashed boxes indicate magnified areas, 40 X objective; scale Bar = 20 μm). Arrows in the magnifications to the right show the altered junction architecture in the absence of arpin (4 X digital zoom; scale Bar = 5 μm). (D) Representative immunostaining for ZO-1 in postcapillary venules of Arpin+/+ and Arpin -/- mice. (n=3; Dashed box indicate delineate magnified area, 40 X objective; Scale Bar = 20 μm), images from the right show junction architecture (4 X digital zoom; Scale Bar = 10 μm).
-
Figure 8—figure supplement 1—source data 1
Uncropped and labeled membranes for Figure 8—figure supplement 1.
- https://cdn.elifesciences.org/articles/90692/elife-90692-fig8-figsupp1-data1-v1.zip
-
Figure 8—figure supplement 1—source data 2
Raw unedited membranes for Figure 8—figure supplement 1.
- https://cdn.elifesciences.org/articles/90692/elife-90692-fig8-figsupp1-data2-v1.zip
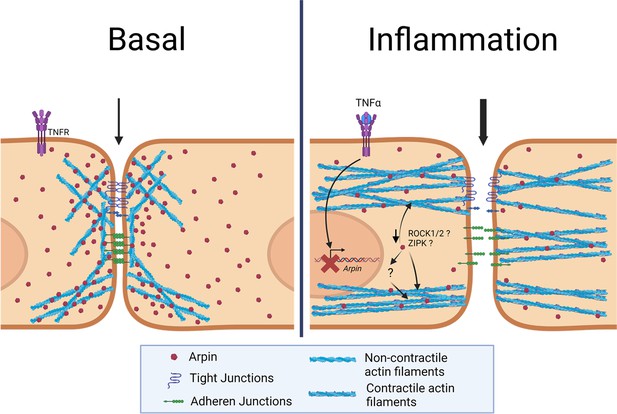
Current working model for arpin functions in endothelial cells.
Under basal conditions, arpin is located throughout the cell and enriched at cellular junctions. During inflammation, arpin is downregulated causing the formation of actomyosin actin stress fibers, junction disruption and increased permeability. Question marks indicate the hitherto unknown mechanism of how arpin controls the activity of Rho-associated protein kinase (ROCK) and Zipper interacting protein kinase (ZIPK) to induce the formation of contractile actin stress fibers.
Additional files
-
Supplementary file 1
Table showing the mating statistics of Arpin+/- mice.
Genotyping of 126 littermates from breedings of heterozygous (Arpin+/-) mice are shown. χ2 test probability is shown. Deviations from Mendelian rules are non-significant.
- https://cdn.elifesciences.org/articles/90692/elife-90692-supp1-v1.docx
-
Supplementary file 2
Hemograms of Arpin+/+ and Arpin-/- mice.
- https://cdn.elifesciences.org/articles/90692/elife-90692-supp2-v1.docx
-
MDAR checklist
- https://cdn.elifesciences.org/articles/90692/elife-90692-mdarchecklist1-v1.docx