Remodeling of skeletal muscle myosin metabolic states in hibernating mammals
Figures
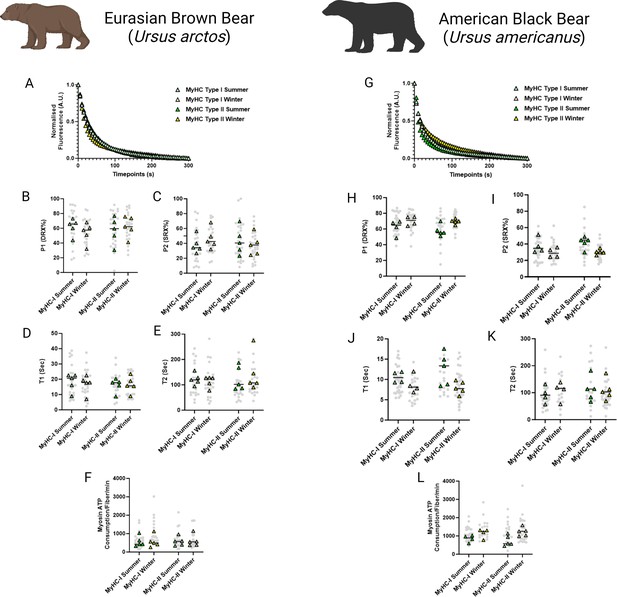
Myosin dynamics and myosin ATP consumption is unchanged in Ursus arctos and Ursus americanus during hibernation.
(A) Representative fluorescence mant-ATP decays from single muscle fibers isolated from Ursus arctos skeletal muscle measured over 300 s. (B–C) Percentage of myosin heads in the P1/DRX (B) or P2/SRX (C) from Ursus arctos single muscle fibers obtained during summer (active) or winter (hibernating) periods. Values were separated based on each individual fiber was MyHC type I or MyHC type II. (D) T1 value in seconds denoting the ATP turnover lifetime of the DRX. (E) T2 value in seconds denoting the ATP turnover lifetime in seconds of the SRX. (F) Calculated myosin ATP consumption values of each single muscle fiber per minute. This was calculated using the equation shown in the Materials and methods section. (G) Representative fluorescence mant-ATP decays from single muscle fibers isolated from Ursus americanus skeletal muscle measured over 300 s. (H–I) Percentage of myosin heads in the P1/DRX (G) or P2/SRX (H) from Ursus americanus single muscle fibers obtained during summer (active) or winter (hibernating) periods. Values were separated based on each individual fiber was MyHC type I or MyHC type II. (J) T1 value in seconds denoting the ATP turnover lifetime of the DRX. (K) T2 value in seconds denoting the ATP turnover lifetime in seconds of the SRX. (L) Calculated myosin ATP consumption values of each single muscle fiber per minute. Grey circles represent the values from each individual muscle fiber which was analyzed. Colored triangles represent the mean value from an individual animal, 8–12 fibers analyzed per animal. Statistical analysis was performed upon mean values. One-way ANOVA was used for statistical testing. n=5 individual animals per group. Figure created using BioRender.com and published using a CC BY-NC-ND license with permission.
© 2024, BioRender Inc. Figure 1 was created using BioRender, and is published under a CC BY-NC-ND 4.0. Further reproductions must adhere to the terms of this license
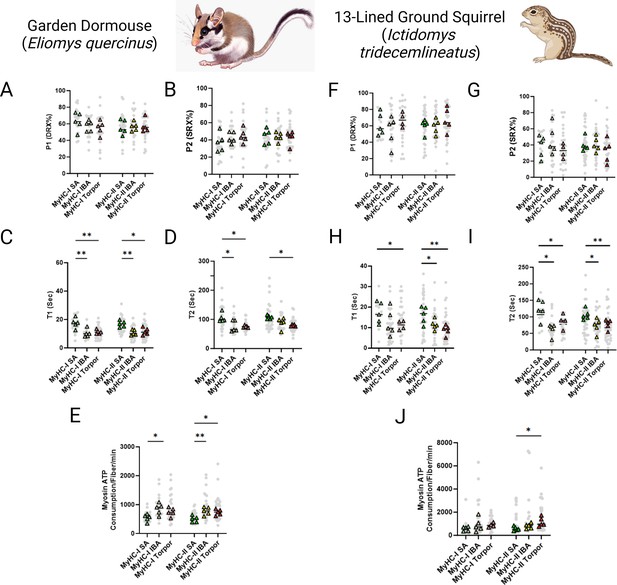
Myosin ATP turnover lifetime is reduced during hibernation in small hibernators, Eliomys quercinus and Ictidomys tridecemlineatus, resulting in an increase in myosin ATP consumption at ambient temperatures.
(A–B) Percentage of myosin heads in the P1/DRX (A) or P2/SRX (B) from E. quercinus single muscle fibers obtained during active, interbout arousal (IBA) or torpor periods. Values were separated based on each individual fiber was MyHC type I or MyHC type II. (C) T1 value in seconds denoting the ATP turnover lifetime of the DRX in E. quercinus. (D) T2 value in seconds denoting the ATP turnover lifetime in seconds of the SRX in E. quercinus. (E) Calculated myosin ATP consumption values of each single muscle fiber per minute in E. quercinus. This was calculated using the equation shown in the Materials and methods section. (F–G) Percentage of myosin heads in the P1/DRX (F) or P2/SRX (G) from I. tridecemlineatus single muscle fibers obtained during summer active (SA), interbout arousal (IBA) or torpor periods. (H) T1 value in seconds denoting the ATP turnover lifetime of the DRX in I. tridecemlineatus. (I) T2 value in seconds denoting the ATP turnover lifetime in seconds of the SRX in I. tridecemlineatus. (J) Calculated myosin ATP consumption values of each single muscle fiber per minute in I. tridecemlineatus. Grey circles represent the values from each individual muscle fiber which was analyzed. Colored triangles represent the mean value from an individual animal, 8–12 fibers analyzed per animal. Statistical analysis was performed upon mean values. One-way ANOVA was used to calculate statistical significance. *=p < 0.05, **=p < 0.01. n=5 individual animals per group. Figure created using BioRender.com and published using a CC BY-NC-ND license with permission.
© 2024, BioRender Inc. Figure 2 was created using BioRender, and is published under a CC BY-NC-ND 4.0. Further reproductions must adhere to the terms of this license
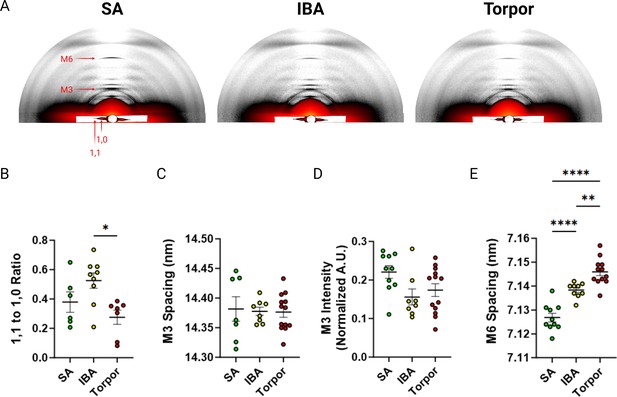
X-ray diffraction experiments of skeletal muscle from Ictidomys tridecemlineaus demonstrate changes in M6 myosin meridional spacing during torpor.
(A) Representative X-ray diffraction recordings from permeabilized skeletal muscle bundles from Ictidomys tridecemlineatus from summer active (SA), interbout arousal (IBA) and torpor. The M3 and M6 meridional reflections and the 1,0 and 1,1 equatorial reflections are indicated. (B) Ratio of the 1,1–1,0 equatorial reflections from active, IBA and torpor skeletal muscle. (C) M3 meridional spacing, measured in nm. (D) Normalized intensity (A.U.) of the M3 meridional reflection. (E) M6 meridional spacing, measured in nm. Colored circles represent the mean value obtained from each skeletal muscle bundle which was recorded. Data is displayed as mean ± SEM. One-way ANOVA was used to calculate statistical significance. *=p < 0.05, **=p < 0.01, ***=p < 0.001. n=5 individual animals per group.
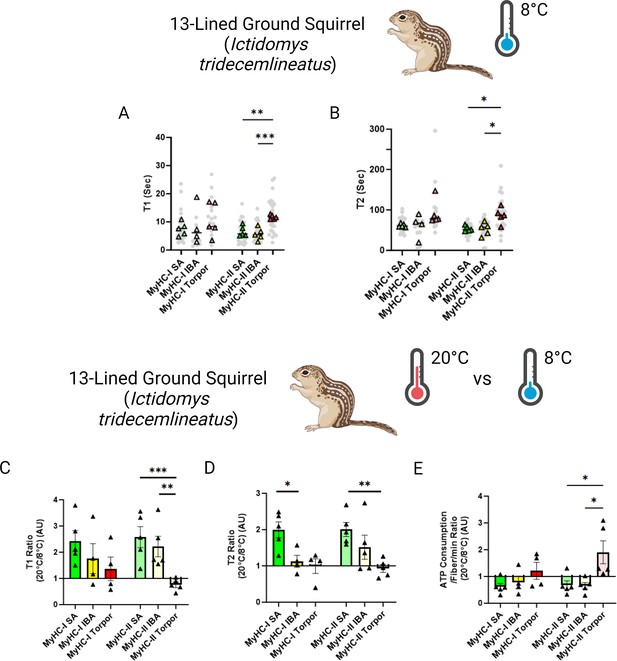
Myosin dynamics of Ictidomys tridecemlineatus are protected from temperature induced change during torpor, preventing an increase in myosin ATP consumption.
(A) T1 value in seconds denoting the ATP turnover lifetime of the DRX in I. tridecemlineatus at 8 °C. (B) T2 value in seconds denoting the ATP turnover lifetime in seconds of the SRX in I. tridecemlineatus at 8 °C. (C) Ratio of the T1 expressed as the mean value for each matched animal at 20 °C/8 °C, separated for fiber type. (D) Ratio of the T2 expressed as the mean value for each matched animal at 20 °C/8 °C, separated for fiber type. (E) Ratio of calculated myosin ATP consumption expressed as 20 °C/8 °C, separated for fiber type. Black triangles represent the mean ratio value for each animal. One-way ANOVA was used to calculate statistical significance. *=p < 0.05, **=p < 0.01, ***=p < 0.001. n=5 individual animals per group. Figure created using BioRender.com and published using a CC BY-NC-ND license with permission.
© 2024, BioRender Inc. Figure 4 was created using BioRender, and is published under a CC BY-NC-ND 4.0. Further reproductions must adhere to the terms of this license
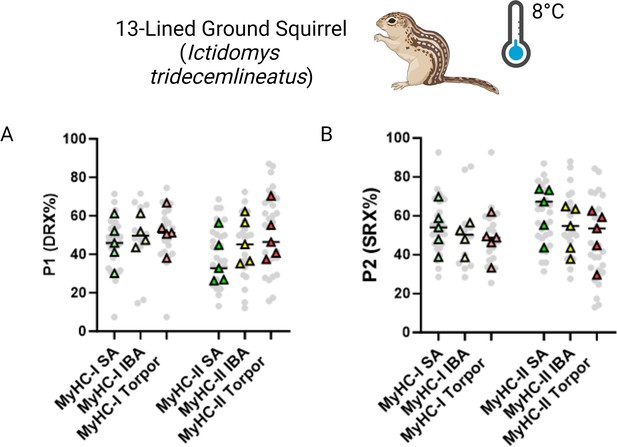
Myosin ATP turnover lifetime is altered following exposure to cold temperature in MyHC-II muscle fibers from I. tridecemlineatus during active and IBA periods but not torpor.
(A–B) Percentage of myosin heads in the P1/DRX (A) or P2/SRX (B) from I. tridecemlineatus single muscle fibers obtained during summer active (SA), interbout arousal (IBA) or torpor periods at 8 °C. Grey circles represent the values from each individual muscle fiber which was analyzed. Colored triangles represent the mean value from an individual animal, 8–12 fibers analyzed per animal. Statistical analysis was performed upon mean values. One-way ANOVA was used to calculate statistical significance. n=5 individual animals per group. Figure created using BioRender.com and published using a CC BY-NC-ND license with permission.
© 2024, BioRender Inc. Figure 4-figure supplement 1 was created using BioRender, and is published under a CC BY-NC-ND 4.0. Further reproductions must adhere to the terms of this license
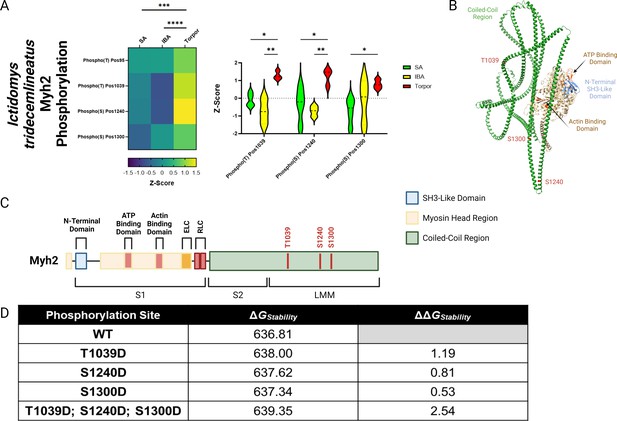
MYH2 protein in Ictidomys tridecemlineatus is hyper-phosphorylated during torpor, which is predicted to increase protein stability.
(A) Peptide mapping of differentiated phosphorylation sites upon MYH2 protein during SA, IBA and torpor periods. Heat map demonstrates all sites observed to be differentiated following the calculation of z-scores for each site. Z-scores >0 equal hyper-phosphorylation and z-scores<0 equal hypo-phosphorylation for each residue. Violin plot demonstrates significantly differentiated residues using z-scores. Two-way ANOVA with Šídák’s multiple comparisons test was used to calculate statistical significance. *=p < 0.05, **=p < 0.01, ***=p < 0.001, ****=p < 0.0001. n=5 individual animals per group. (B) Chimera of MYH2 protein created using ChimeraX software. Important regions of the protein are annotated including coiled-coil region, ATP binding domain, actin binding domain and N-terminal SH3-like domain. Also, significantly hyper-phosphorylated residues are highlighted in red. (C) Schematic of MYH2 protein with regions and hyper-phosphorylated resides annotated in red. Figure made in BioRender. (D) EvoEF calculations of protein stability in both wild type and phosphor-mimetic mutants. Aspartic acid was used to mimic phospho-threonine/phospho-serine due to their chemical similarity. ΔGStability indicates the stability score for the protein in its corresponding configuration. ΔΔGStability represents the change in stability in mutant proteins versus the wild type protein. ΔΔGStability of >0 represents an increase in the stability of a mutant versus wild type. Panel C created using BioRender.com and published using a CC BY-NC-ND license with permission.
© 2024, BioRender Inc. Figure 5 was created using BioRender, and is published under a CC BY-NC-ND 4.0. Further reproductions must adhere to the terms of this license
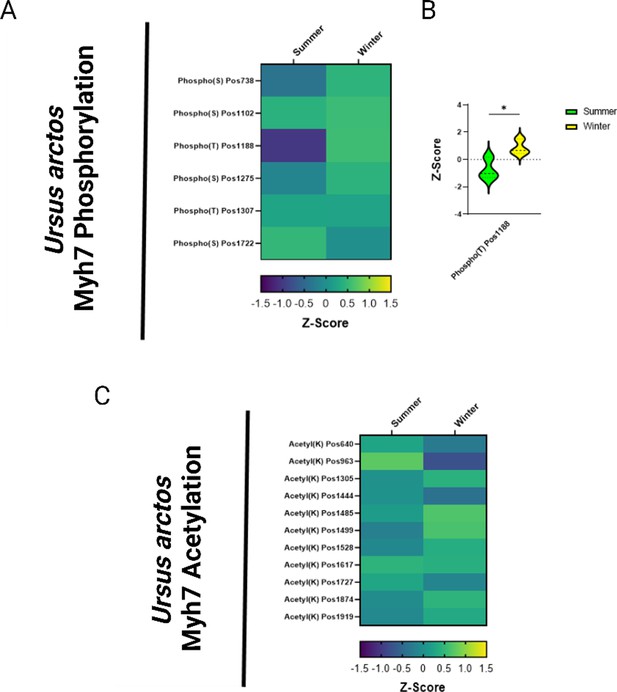
MYH7 protein phosphorylation and acetylation in U. arctos is relatively unchanged during winter periods.
(A) Peptide mapping of differentiated phosphorylation sites upon MYH7 protein during summer and winter periods. Heat map demonstrates all sites observed to be differentiated following the calculation of z-scores for each site. Z-scores >0 equal hyper-phosphorylation and z-scores <0 equal hypo-phosphorylation for each residue. (B) Violin plot demonstrates significantly differentiated residue using z-scores. (C) Peptide mapping of differentiated acetylation sites upon MYH7 protein during summer and winter periods. Heat map demonstrates all sites observed to be differentiated following the calculation of z-scores for each site. Z-scores >0 equal hyper-acetylation and z-scores <0 equal hypo-acetylation for each residue. Two-way ANOVA with Šídák’s multiple comparisons test was used to calculate statistical significance. *=p < 0.05. n=5 individual animals per group.
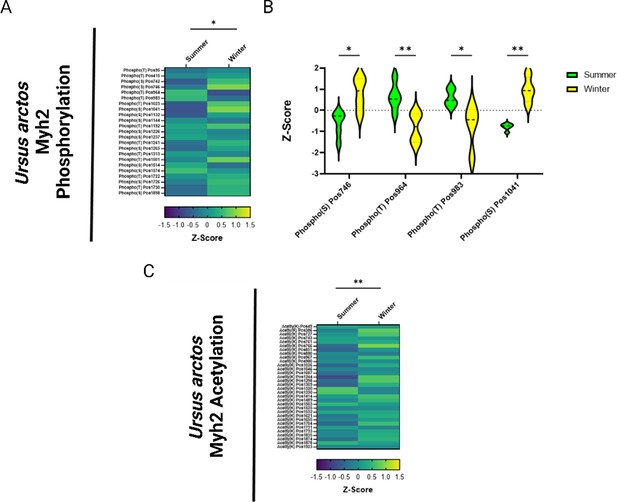
MYH2 protein phosphorylation and acetylation in U.
arctos is altered during winter periods. (A) Peptide mapping of differentiated phosphorylation sites upon MYH2 protein during summer and winter periods. Heat map demonstrates all sites observed to be differentiated following the calculation of z-scores for each site. Z-scores >0 equal hyper-phosphorylation and z-scores <0 equal hypo-phosphorylation for each residue. (B) Violin plot demonstrates significantly differentiated residues using z-scores. (C) Peptide mapping of differentiated acetylation sites upon MYH7 protein during summer and winter periods. Heat map demonstrates all sites observed to be differentiated following the calculation of z-scores for each site. Z-scores >0 equal hyper-acetylation and z-scores <0 equal hypo-acetylation for each residue. Two-way ANOVA with Šídák’s multiple comparisons test was used to calculate statistical significance. *=p < 0.05, **=p < 0.01. n=5 individual animals per group.
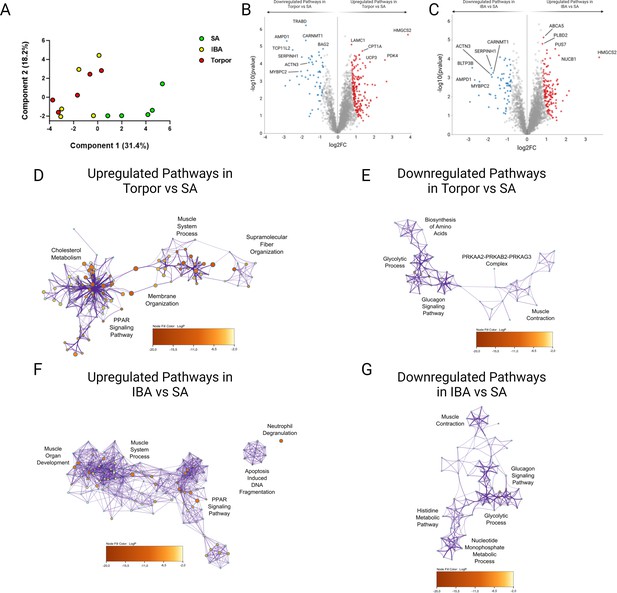
Global proteome analysis demonstrates changes to metabolic and sarcomeric changes in skeletal muscle fibers from Ictidomys tridecemlineatus during IBA and torpor.
(A) Principal component analysis for all animals analyzed during SA, IBA and torpor periods. (B) Volcano plot displaying proteins which are differentially expressed during torpor vs active periods. FDR < 0.01. Red circles are upregulated proteins and blue circles are downregulated proteins. Highly differentiated proteins of interest are annotated with their respective protein name. (C) Volcano plot displaying proteins which are differentially expressed during IBA vs SA periods. Red circles are upregulated proteins and blue circles are downregulated proteins. Highly differentiated proteins of interest are annotated with their respective protein name. (D) Ontological associations between proteins upregulated during torpor vs SA periods. The top five association clusters are annotated on the network. A full list of clusters and the proteins lists included in clusters are available in Figure 6—figure supplement 1 and Supplementary file 2. (E) Ontological associations between proteins downregulated during torpor vs SA periods. The top five association clusters are annotated on the network. A full list of clusters and the proteins lists included in clusters are available in Figure 6—figure supplement 1 and Supplementary file 2. (F) Ontological associations between proteins upregulated during IBA vs SA periods. The top five association clusters are annotated on the network. A full list of clusters and the proteins lists included in clusters are available in Figure 6—figure supplement 2 and Supplementary file 3. (G) Ontological associations between proteins downregulated during IBA vs SA periods. The top five association clusters are annotated on the network. A full list of clusters and the proteins lists included in clusters are available in Figure 6—figure supplement 2 and Supplementary file 3. Gene ontology networks were established using Metascape and visualized using Cytoscape. Detailed information upon the statistical testing used is available in the methods section. FDR < 0.01 significantly differentially expressed proteins were used to establish networks. Purple lines indicate a direct interaction. Circle size is determined by enrichment and color is determined by p value. n=5 individual animals per group.
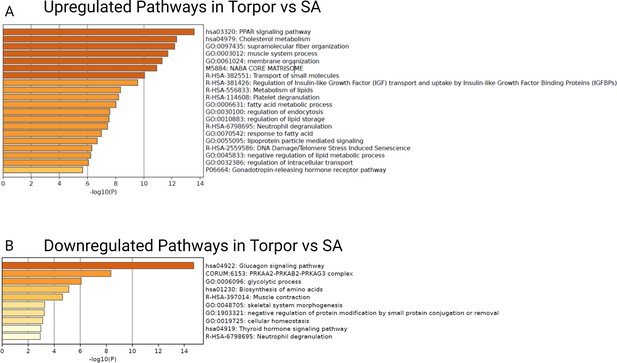
All ontological clusters altered in I. tridecemlineatus in torpor vs SA periods.
(A) All upregulated pathways identified in Metascape in torpor vs SA periods. Pathways are listed in order of significance by -log10(p). (B) All downregulated pathways identified in Metascape in torpor vs SA periods. Pathways are listed in order of significance by -log10(p).
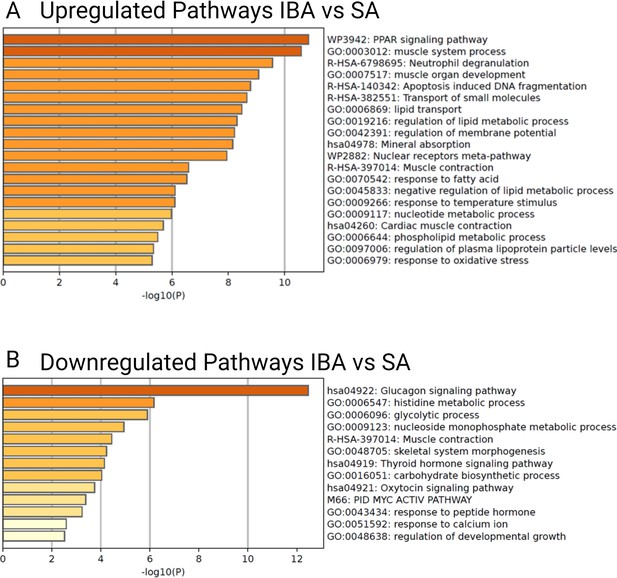
All ontological clusters altered in I. tridecemlineatus in IBA vs SA periods.
A. All upregulated pathways identified in Metascape in IBA vs SA periods. Pathways are listed in order of significance by -log10(p). (B) All downregulated pathways identified in Metascape in IBA vs SA periods. Pathways are listed in order of significance by -log10(p).
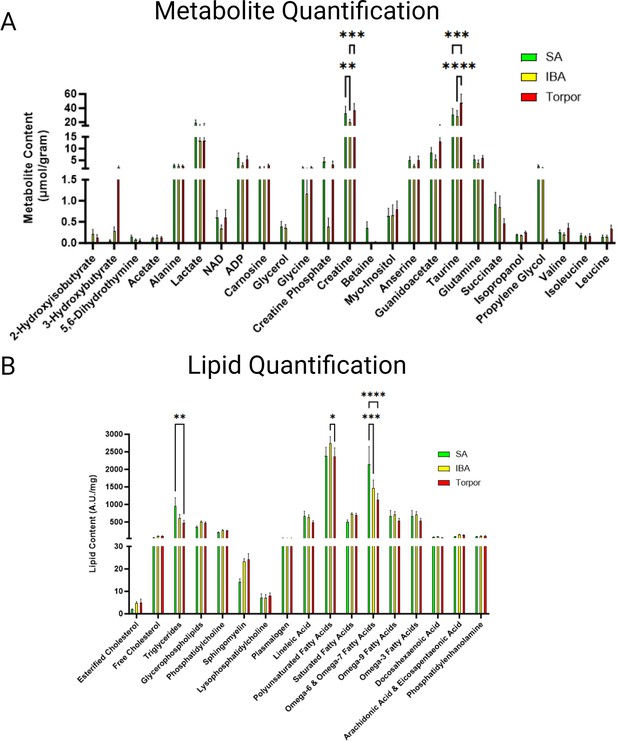
Metabolite and lipid quantification of skeletal muscle from I.tridecemlineatus reveals a decrease in lipid levels during torpor.
(A) Quantification of metabolites from the skeletal muscle tissue of I. tridecemlineatus during SA, IBA, and torpor periods. Presented in µmol of metabolite per gram of tissue. (B) Quantification of lipids from the skeletal muscle tissue of I. tridecemlineatus during SA, IBA, and torpor periods. Presented in A.U. of lipid per mg of tissue. Two-way ANOVA with Turkey’s multiple comparisons test was used to calculate statistical significance. *=p < 0.05, **=p < 0.01, ***=p < 0.001, ****=p < 0.0001. n=5 individual animals per group.
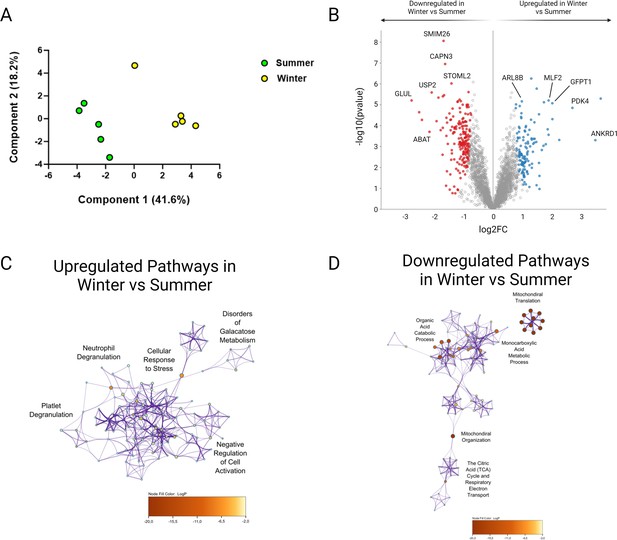
Global proteome analysis of U.arctos skeletal muscle fibers reveal metabolic changes but not sarcomeric changes.
(A) Principal component analysis for all animals analyzed during summer and winter periods. (B) Volcano plot displaying proteins which are differentially expressed during winter vs summer periods. FDR < 0.01. Red circles are upregulated proteins and blue circles are downregulated proteins. Highly differentiated proteins of interest are annotated with their respective protein name. (C) Ontological associations between proteins upregulated during winter vs summer periods. The top five association clusters are annotated on the network. A full list of clusters and the proteins lists included in clusters are available in Figure 6—figure supplement 5 of this figure and Supplementary file 4. (D) Ontological associations between proteins downregulated during winter vs summer periods. The top five association clusters are annotated on the network. A full list of clusters and the proteins lists included in clusters are available in Figure 6—figure supplement 5 of this figure and Supplementary file 4. Gene ontology networks were established using Metascape and visualized using Cytoscape. Detailed information of the statistical testing used is available in the methods section. FDR < 0.01 significantly differentially expressed proteins were used to establish networks. Purple lines indicate a direct interaction. Circle size is determined by enrichment and color is determined by p value. n=5 individual animals per group.
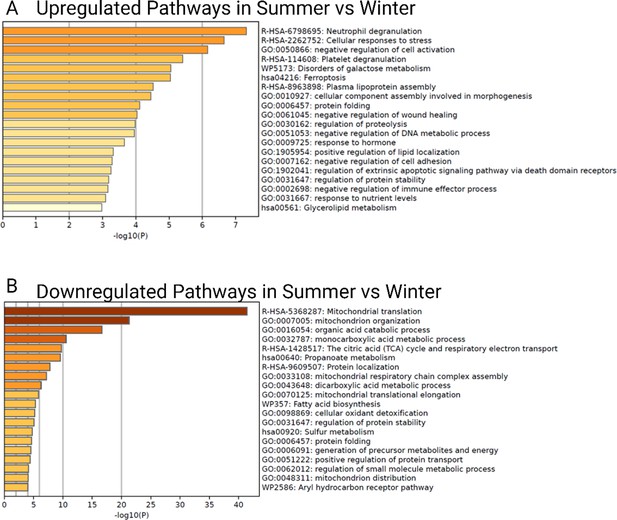
All ontological clusters altered in U.arctos in summer vs winter periods.
(A) All upregulated pathways identified in Metascape in winter vs summer periods. Pathways are listed in order of significance by -log10(p). (B) All downregulated pathways identified in Metascape in winter vs summer periods. Pathways are listed in order of significance by -log10(p).
Additional files
-
Supplementary file 1
Fiber type compositions from animals used for this study.
Table demonstrating the percentage of fibers which were analyzed during Mant-ATP chase assays that were either MyHC type I or MyHC type II. Data is presented as mean for each animal ± SD. One-way ANOVA was used to calculate significance between hibernation periods in I. tridecemlineatus and E. quercinus. Student’s t-test was used to calculate significant between hibernating periods in U. arctos and U. americanus and between MyHC type I and MyHC type II in all animals. ##=p < 0.01 vs MyHC type I in corresponding group. ####=p < 0.0001 vs MyHC type I in corresponding group. n=5 individual animals per group.
- https://cdn.elifesciences.org/articles/94616/elife-94616-supp1-v1.docx
-
Supplementary file 2
Detailed protein lists for top five differentially expressed ontological clusters in I. tridecemlineatus in torpor vs SA periods.
A. Table details the list of proteins which were found to be differentially upregulated in torpor vs SA in each corresponding ontological cluster as identified by Metascape. Clusters are arranged by order of statistical significance. Proteins are listed in alphabetical order within each cluster. B. Table details the list of proteins which were found to be differentially downregulated in torpor vs SA in each corresponding ontological cluster as identified by Metascape. Clusters are arranged by order of statistical significance. Proteins are listed in alphabetical order within each cluster. n=5 individual animals per group.
- https://cdn.elifesciences.org/articles/94616/elife-94616-supp2-v1.docx
-
Supplementary file 3
Detailed protein lists for top five differentially expressed ontological clusters in I. tridecemlineatus in IBA vs SA periods.
A. Table details the list of proteins which were found to be differentially upregulated in IBA vs SA in each corresponding ontological cluster as identified by Metascape. Clusters are arranged by order of statistical significance. Proteins are listed in alphabetical order within each cluster. B. Table details the list of proteins which were found to be differentially downregulated in IBA vs SA in each corresponding ontological cluster as identified by Metascape. Clusters are arranged by order of statistical significance. Proteins are listed in alphabetical order within each cluster. n=5 individual animals per group.
- https://cdn.elifesciences.org/articles/94616/elife-94616-supp3-v1.docx
-
Supplementary file 4
Detailed protein lists for top five differentially expressed ontological clusters in U. arctos in winter vs summer periods.
A. Table details the list of proteins which were found to be differentially upregulated in winter vs summer in each corresponding ontological cluster as identified by Metascape. Clusters are arranged by order of statistical significance. Proteins are listed in alphabetical order within each cluster. B. Table details the list of proteins which were found to be differentially downregulated in winter vs summer in each corresponding ontological cluster as identified by Metascape. Clusters are arranged by order of statistical significance. Proteins are listed in alphabetical order within each cluster. n=5 individual animals per group.
- https://cdn.elifesciences.org/articles/94616/elife-94616-supp4-v1.docx
-
MDAR checklist
- https://cdn.elifesciences.org/articles/94616/elife-94616-mdarchecklist1-v1.docx