Modulation of biophysical properties of nucleocapsid protein in the mutant spectrum of SARS-CoV-2
Figures
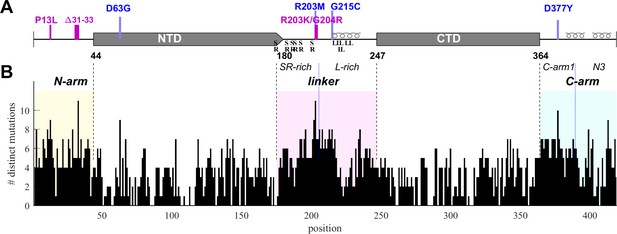
Structural organization and sequence plasticity of N-protein.
(A) Schematics of folded regions (NTD and CTD, rectangles) and disordered regions (N-arm, linker, and C-arm, straight line) along the N-protein sequence. Defining mutations from the Delta variant are indicated in blue, those from Omicron variants in magenta. Transient helices in the disordered regions are highlighted, as well as SR-rich and L-rich linker sequences and the C-terminal N3 region. (B) Histogram of the number of distinct amino acid mutations at each position. For clarity and reference to other figures, intrinsically disordered regions (IDRs) are shaded with N-arm highlighted in yellow, linker in magenta, and C-arm in cyan.
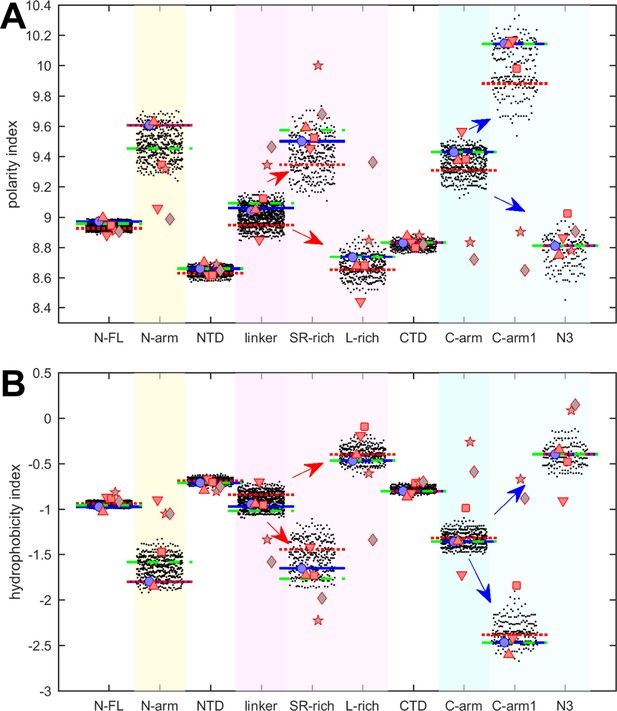
Beehive plots showing the distributions of polarity and hydrophobicity of viable N-protein species across the mutant spectrum.
The polarity index (A) and hydrophobicity index (B) was calculated based on amino acid composition for all distinct sequences of N-FL, the folded domains (NTD and CTD), and the intrinsically disordered regions (IDRs) (N-arm, linker, and C-arm). Further subdivisions of the linker into the SR-rich and L-rich regions, and subdivisions of the C-arm into the N3 region and the C-terminal remainder of the C-arm (C-arm1) are indicated in the arrows. Highlighted by horizontal lines are the values for the corresponding peptides from the ancestral sequence Wuhan-Hu-1 (blue), and including the defining mutations of the Delta variant (dotted red) and the Omicron variant (dashed green), respectively. Symbols indicate values for SARS-CoV-2 (ancestral reference, light blue circles), and corresponding peptides from SARS-CoV-1 (red up triangles), Middle East respiratory syndrome coronavirus (MERS) (red down triangles), murine hepatitis virus (MHV) (red squares), human coronavirus NL63 (gray pentagrams), and the bat coronavirus APD51511.1 (gray diamonds).
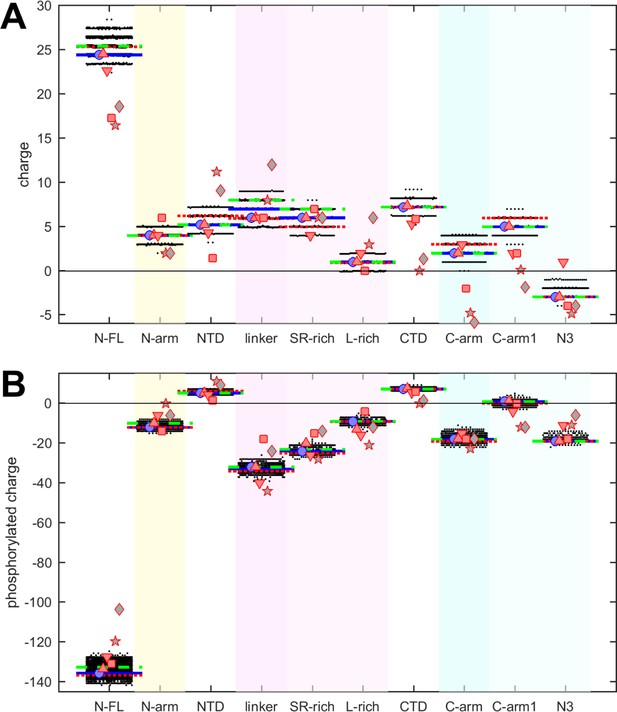
Beehive plots showing the distributions of charges of viable N-protein species.
(A) Charges were calculated based on the amino acid composition of different N-protein regions as in Figure 2. Highlighted by horizontal lines are the values for the corresponding peptides from the ancestral sequence Wuhan-Hu-1 (blue), and including the defining mutations of the Delta variant (dotted red) and the Omicron variant (dashed green), respectively. Symbols indicate values for SARS-CoV-2 (ancestral sequence, blue circles), SARS-CoV-1 (red up triangles), Middle East respiratory syndrome coronavirus (MERS) (red down triangles), murine hepatitis virus (MHV) (red squares), NL63 (gray pentagrams), and bat coronavirus APD51511.1 (gray diamonds). (B) Same as in (A), with added charges from maximally phosphorylated serine, threonine, and tyrosine residues in the intrinsically disordered regions (IDRs).
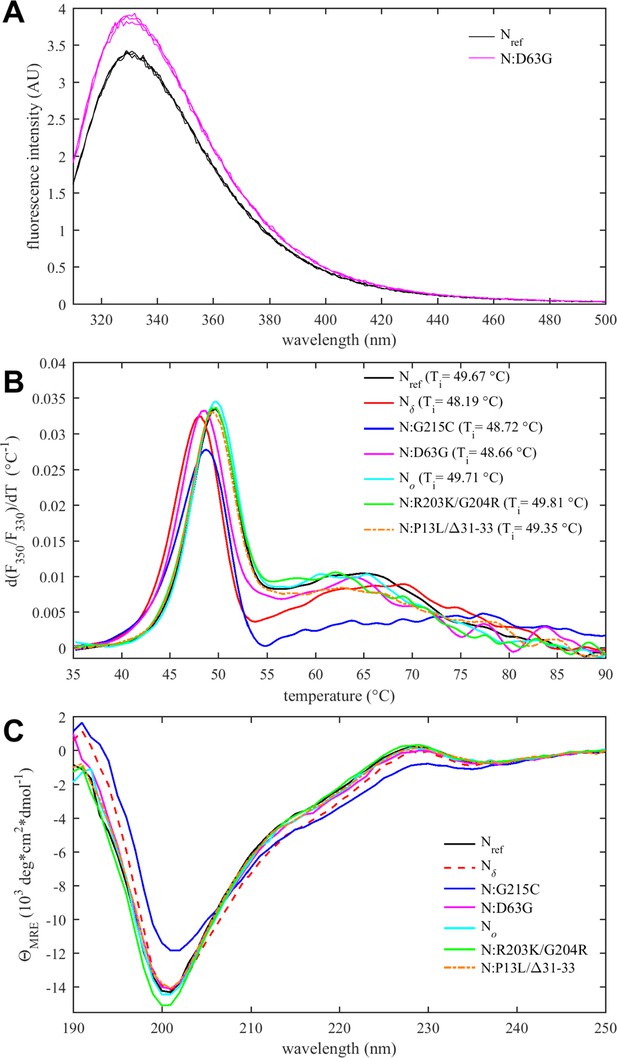
Thermodynamic stability and structural differences of N-protein reference and mutant species.
(A) Intrinsic fluorescence spectrum of N:D63G in comparison with Nref, showing spectra in triplicate. (B) Differential scanning fluorometry, with the temperature of maximum fluorescence ratio derivative (Ti-values, with an estimated precision 0.3°C). (C) Circular dichroism spectra of all N-protein species (spectra with error bars are shown in Figure 4—figure supplement 3).
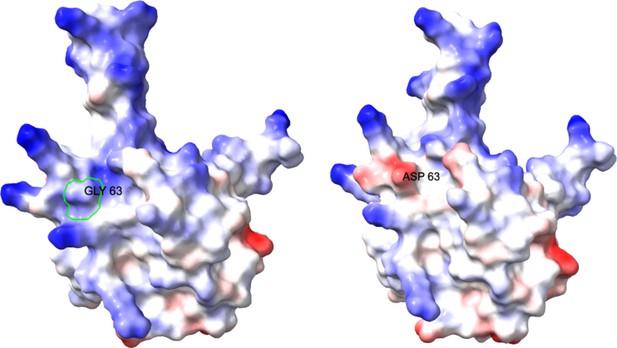
Structural comparison of N:D63G mutant and ancestral N-protein.
Structures are predicted using ColabFold for N:D63G (left) and the ancestral protein (right).
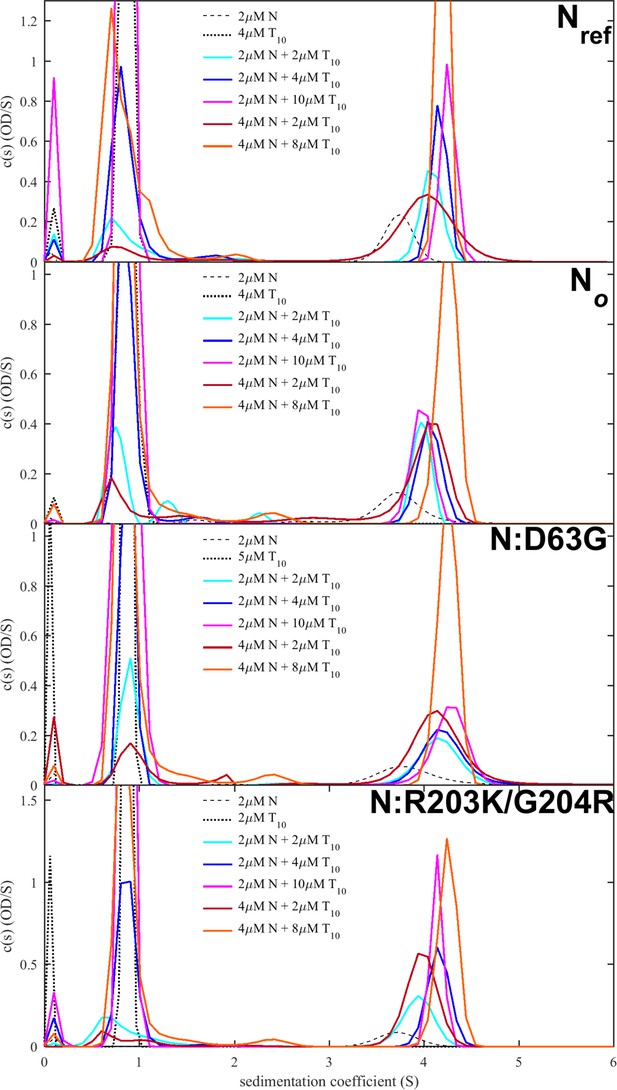
N-protein affinity for binding nucleic acids (NA) probed by sedimentation velocity analytical ultracentrifugation (SV-AUC) of N-protein mixtures with oligonucleotide T10.
T10 can occupy the NA binding grove of the NTD of N-protein, but does not permit multi-valent binding. Titration series of N-protein with T10 allows separation of concentration-dependent populations of free and bound/co-migrating T10 in the mixtures. This provides the basis for the determination of equilibrium binding constants through non-linear regression of the isotherm of signal weighted-average sedimentation coefficients using a two-site binding model of T10 to N-protein dimers. Best-fit KD-values and 95% confidence intervals are 1.1 [0.8–1.6] µM for Nref, 2.8 [1.6–4.9] µM for No, 2.4 [1.1–5.0] µM for N:D63G, and 1.3 [0.9–1.9] µM for N:R203K/G204R, respectively. SV-AUC experiments were carried out in buffer high-salt (HS). Similarly, no significant difference was measured in binding affinity between Nref and N:D63G was observed in buffer low-salt (LS).
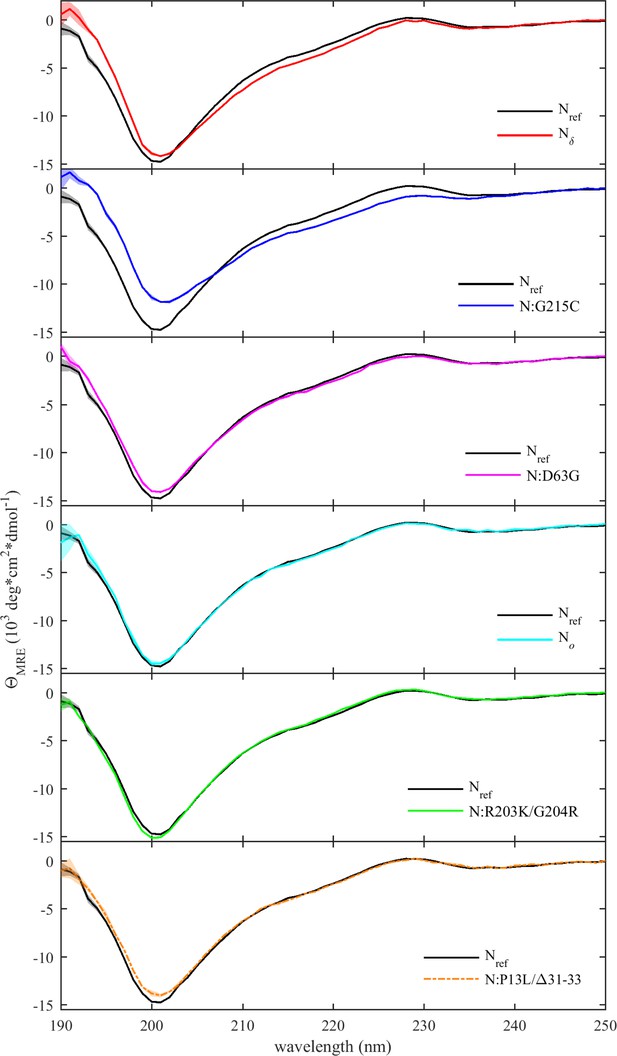
Individual comparison of circular dichroism (CD) spectra.
The data from Figure 4C are reproduced and plotted in comparison with Nref. Standard deviations from three acquired spectra are depicted as shaded bands.
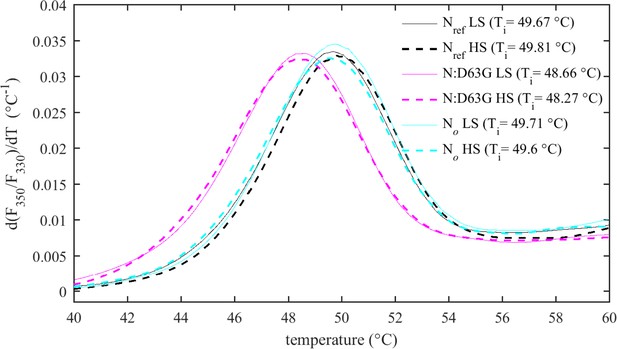
Comparison of differential scanning fluorometry (DSF) data of N-protein species in low-salt (LS) and high-salt (HS) buffer.
Protein preparations were dialyzed in either HS buffer consisting of 20 mM HEPES, 150 mM NaCl, pH 7.5, or LS buffer consisting of 10.1 mM Na2PO4, 1.8 mM KH2PO4, 2.7 mM KCl, 10 mM NaCl, pH 7.4. DSF experiments show no significant shift in Ti for the same protein species in LS or HS buffer.
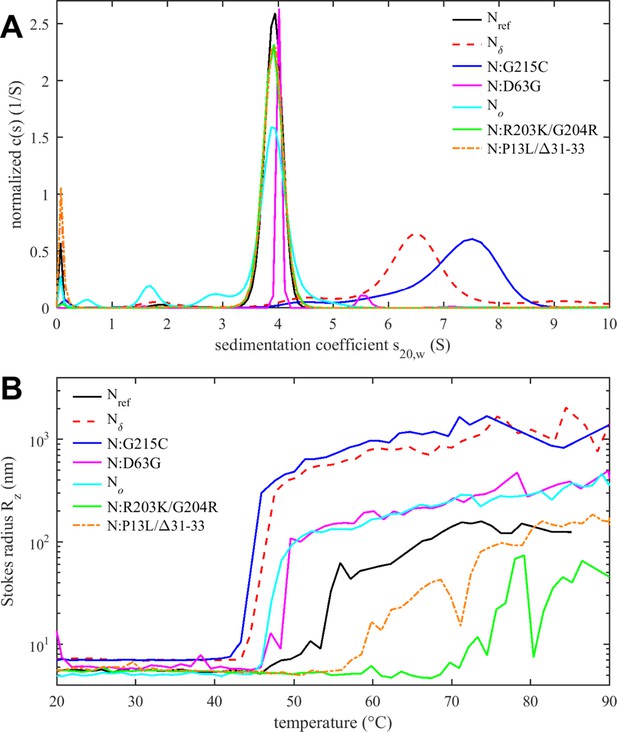
Tertiary and quaternary structure of N-protein species.
(A) Sedimentation coefficient distributions c(s) from sedimentation velocity analytical ultracentrifugation (SV-AUC) experiments show ≈4 S dimers and higher oligomers. Data for N:G215C and Nδ are reproduced from Zhao et al., 2022. (B) Temperature-dependent particle formation reported as average Stokes radius measured by dynamic light scattering.
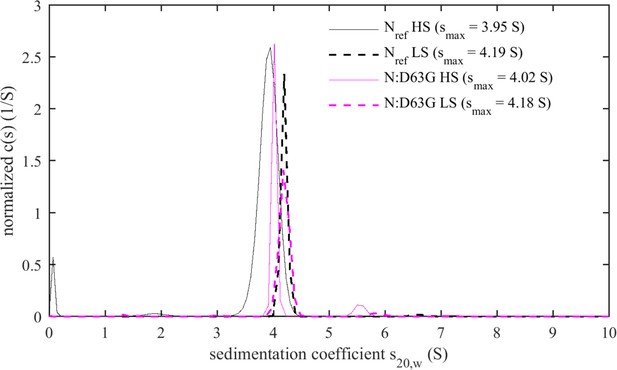
Comparison of solution state of N-protein species in low-salt (LS) and high-salt (HS) buffer.
Protein preparations were dialyzed in either HS buffer consisting of 20 mM HEPES, 150 mM NaCl, pH 7.5, or LS buffer consisting of 10.1 mM Na2PO4, 1.8 mM KH2PO4, 2.7 mM KCl, 10 mM NaCl, pH 7.4. (A) Differential scanning fluorometry (DSF) experiments show no significant shift in Ti for the same protein species in LS or HS buffer. (B) Sedimentation velocity analytical ultracentrifugation (SV-AUC) exhibit sedimentation coefficient distributions with peak s-values increased by ≈5% in LS buffer relative to HS buffer. This apparent change is negligible compared to the ≈60–90% increase in sedimentation coefficients from altered oligomeric states observed for N:G215C and Nδ (Figure 5A).
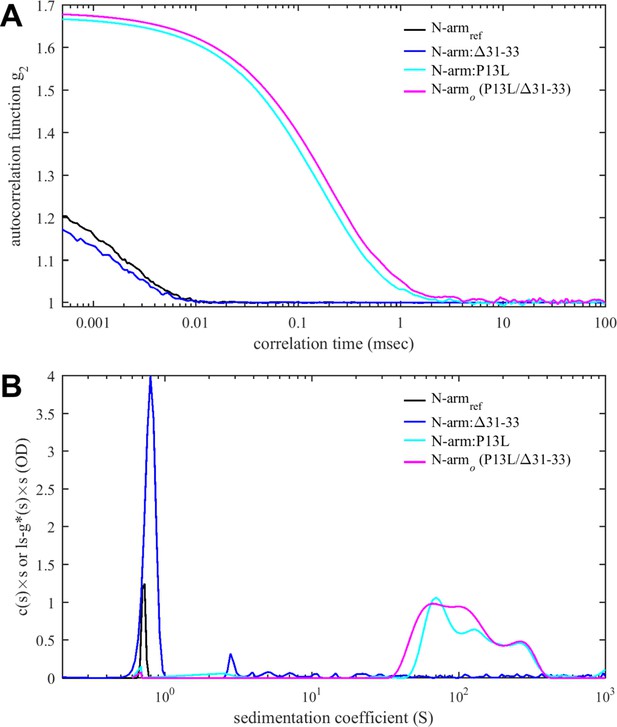
Protein-protein interactions of N-arm peptide containing the Omicron P13L mutation lead to large structures at high concentrations.
(A) Autocorrelation functions from dynamic light scattering (DLS) (A) and sedimentation coefficient distributions from sedimentation velocity analytical ultracentrifugation (SV-AUC) (B) for the ancestral reference Nref:(1–43) (black), N:Δ31–33(1–43) (blue), N:P13L(1–43) (cyan), and N:P13L/Δ31–33(1–43) (identical to the Omicron N-arm, magenta). All peptide concentrations are 400 µM, except for Nref:(1–43) in the SV-AUC experiment which is 275 µM, reproduced from previously reported data (Zhao et al., 2023).
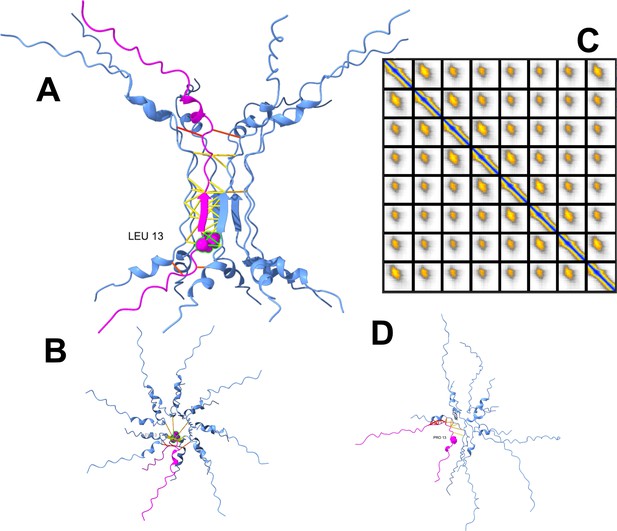
Structural prediction of Omicron N-arm self-interactions.
(A) Best ColabFold prediction of eight Omicron N-arm (1:41) peptides with P13L and Δ31–33 mutations. For one chain shown in magenta, atoms of 13L are depicted and labeled, and contacts of this chain within 3.5 Å are color-coded by confidence. (B) Top view of (A). (C) Predicted alignment error (PAE) map showing symmetry and confidence of predicted interactions. (D) Best analogous prediction of ancestral N-arm interactions, highlighting the absence of order.
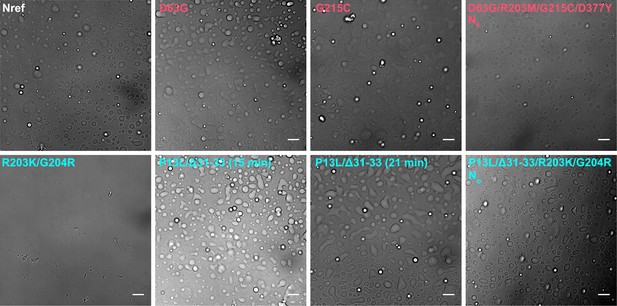
Differences in liquid-liquid phase separation (LLPS) propensity of N-protein mutant species.
Optical microscopy images were taken of 10 μM N-protein with 5 μM T40 (except Nδ, which is 4 μM N-protein with 2 μM T40) in low-salt (LS) buffer after incubation for 15 min at room temperature. For N:P13L/Δ31–33, a second image was taken at the 21 min time point highlighting the growth of condensed phases. All scale bars are 10 µm. Histograms of particle areas are in Figure 7—figure supplement 1, and a comparison of two time points for Nref, N:R203K/G204R and N:P13L/Δ31–33, is provided in Figure 7—figure supplement 2.
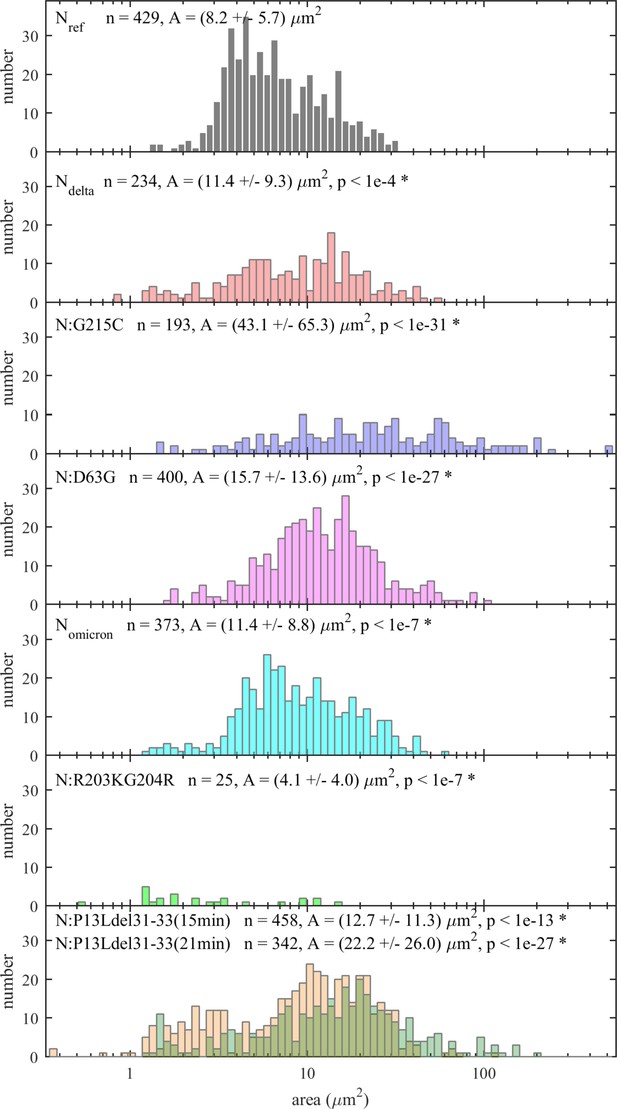
Comparison of area distributions of droplets in images of Figure 7.
For each N-protein species, images were segmented to identify droplets. The values indicated are particle numbers, the mean area, the standard deviation of the area, and the probability that the sample is from the same distribution as Nref based on the two-sample Kolmogorov-Smirnov test.
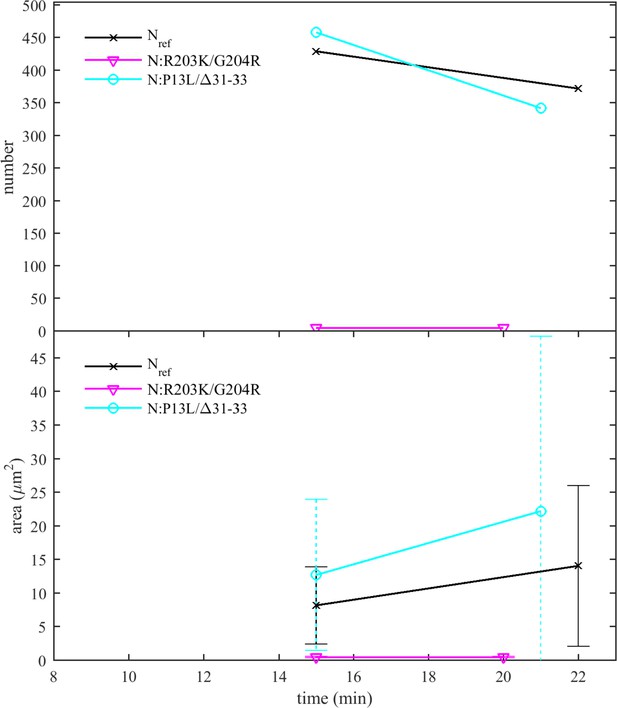
Comparison of droplet area after liquid-liquid phase separation (LLPS) at two points in time.
Similar to Figure 7, images of LLPS were recorded for Nref, N:R203K/G204R, and N:P13L/Δ31–33 at two time points for the same sample. The upper plot shows droplet numbers. The lower plot shows mean and standard deviations of the droplet area. Images and histograms for the early time points and the later time point of N:P13L/Δ31–33 are shown in Figure 7 and Figure 7—figure supplement 1, respectively.
Tables
Sequence alignment score of segments from related coronaviruses.
Virus | Full-length | N-arm | NTD | Linker | SR-rich | L-rich | CTD | C-arm | C-arm1 | N3 |
---|---|---|---|---|---|---|---|---|---|---|
SARS-CoV-1 | 672* | 68.6 | 263 | 41.6 | 44.7 | 30 | 231 | 60.5 | 75.3 | 77 |
MERS | 276 | 13.9 | 157 | 112 | 14.6 | 23.5 | ||||
MHV | 192 | 114 | 14.6 | 80.5 | 14.6 | 13.4 | ||||
NL63 | 67.4 | 58.9 | 61.6 | |||||||
APD51511.1 | 61.2 | 44.3 | 44.3 |
-
*
Values are BLASTp total alignment scores.
Overview of N-protein species compared in biophysical experiments.
Designation | N-protein mutations | n exclusive instances* | Occur in # of distinct sequences† | Occurs in % of all genomes ‡ | In set of defining VOC mutations § |
---|---|---|---|---|---|
N:R203K/G204R | R203K, G204R | 53,282 | 17,552 | 57% | α, γ, ο |
N:P13L/Δ31–33 | P13L, Δ31–33 | 9548 | 12,503 | 47% | ο |
Nο | P13L, Δ31–33, R203K, G204R | 791,613 | 10,238 | 46% | ο (all BA.1)¶ |
Nδ | D63G, R203M, G215C, D377Y | >1.2 × 106 | 9397 | 33% | δ (all 21J)¶ |
N:G215C | G215C | 60 | 10,562 | 34% | δ |
N:D63G | D63G | 182 | 12,443 | 36% | δ |
Nref | none | 38,929 | NA | 3.6% | NA |
-
*
Number of genomes where the indicated mutations are the only N mutations.
-
†
Number of unique N-protein sequences in which indicated mutations are present, alongside other mutations.
-
‡
Percentage of all sequenced genomes carrying the specific mutation.
-
§
Variants of concern for which indicated mutations are part (or all) of the defining set of N-m.
-
¶
These sets of mutations comprise all defining N-protein mutations of this variant. Literature on definition or biophysical characterization of the mutant.
Additional files
-
Supplementary file 1
Sequence alignment of nucleocapsid protein of related coronaviruses and sequences corresponding to SARS-CoV-2 regions.
N-protein sequences of SARS-CoV-1 P59595.1, MERS YP_009047211.1, MHV NP_045302.1, human coronavirus NL63 Q6Q1R8.1, and 229E-related bat coronavirus APD51511.1 were aligned with SARS-CoV-2 N-protein. Regions corresponding to the SARS-CoV-2 regions (N-arm, NTD, linker, SR-rich, L-rich, CTD, Carm, Carm1, N3) and their alignment score were determined.
- https://cdn.elifesciences.org/articles/94836/elife-94836-supp1-v1.xlsx
-
MDAR checklist
- https://cdn.elifesciences.org/articles/94836/elife-94836-mdarchecklist1-v1.pdf