Parasites: Do trypanosome turncoats wait before they commit?
African trypanosomes cause sleeping sickness in humans, which is potentially lethal if left untreated. However, in order to spread to new hosts, these deadly parasites must be transmitted by tsetse flies, and this need to alternate between life in the mammal and life in the insect has made their biology unique. This includes the ability to undergo a metamorphosis from a form that rapidly proliferates within the bloodstream to a non-proliferative form that is prepared for life in the fly (Figure 1A; MacGregor et al., 2011, 2012). Normally, this change takes place when the concentration of the proliferative form of the parasite in the bloodstream reaches a certain density.
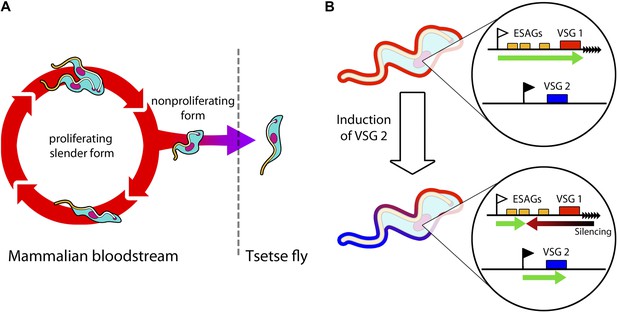
Trypanosomes must radically change in order to survive in both mammals and tsetse flies.
(A) Trypanosomes proliferating in the mammalian bloodstream change into a non-proliferative form that is capable of transferring into a tsetse fly. All bloodstream trypanosomes have a dense surface VSG coat. (B) Trypanosomes express VSG proteins from a VSG expression site at a chromosome end (black triangles). The VSG expression sites include expression site associated genes (ESAGs; yellow boxes) as well as the telomeric VSG genes (red box). In the top panel, VSG1 is being expressed, with transcription (green arrow) proceeding from a promoter (white flag). Batram et al. found that inducing parasites to express a different VSG—labelled here as VSG 2 (blue box)—from another genomic location (black flag) results in a repressive gradient silencing the active expression site (red arrow). This not only silences VSG 1, but also reduces the expression of ESAGs near VSG 1.
In the mammal, trypanosomes are covered with a protective coat made of variant surface glycoprotein (VSG). In order to avoid detection by host antibodies, trypanosomes repeatedly switch to new VSG coats (Horn and McCulloch, 2010). Now, in eLife, Markus Engstler and colleagues from the University of Würzburg—including Christopher Batram as first author—argue that the process of switching the VSG coat could play a central role in both helping the trypanosome to avoid the mammalian immune system and helping it to escape from the mammal into the insect (Batram et al., 2014).
In the bloodstream, trypanosomes express only one VSG coat at a time out of a repertoire of hundreds. The active VSG is expressed in a strictly mutually exclusive fashion from an expression site located at the end of a chromosome (Figure 1B). Batram et al. forced the trypanosome to express a different VSG from elsewhere in the genome. Surprisingly, a repressive gradient that extends from the chromosome end resulted in the active VSG expression site shutting down. This silencing was dependent on a histone methyltransferase (Figueiredo et al., 2008), indicating that chromatin remodelling was involved. Amazingly, this expression site silencing also appeared to trigger trypanosomes to change from the proliferative form that undergoes continuous division in the bloodstream to become the non-proliferative form that is adapted for life in the insect.
The VSG expression sites also contain expression site associated genes (ESAGs; Hertz-Fowler et al., 2008). Using genetic techniques to knock down these genes also triggered the trypanosome to change from the proliferative to the non-proliferative form. It therefore appears that some expression site associated genes could function as ‘sensors’ that cue trypanosomes to become quiescent when the expression site shuts down. This suggests that VSG expression site silencing—which could potentially be reversed—may be involved in trypanosomes preparing for life in the tsetse fly.
This reveals an interesting and unexpected connection between the silencing of the active VSG expression site and the escape of trypanosomes out of the infected host. As silencing the active expression site is reversible, the proliferating form of the parasite may be able to adopt a ‘wait and see’ state before committing to becoming non-proliferative. This challenges the paradigm that commitment to a non-proliferative form depends on the population density of the parasites, and is an irreversible process.
However, these experiments raise more questions than they answer. It is not clear if this causative link between expression site silencing and progression through the life cycle also occurs in a natural infection. Alternatively, it could be a byproduct of forcing the trypanosome to express high levels of a second surface coat gene. How often would a trypanosome normally find itself in a situation where high levels of a second VSG are expressed, but not the associated ESAGs?
Batram et al. provide illuminating insight into how trypanosomes can silence their active VSG expression site, and show that this can proceed directionally from the chromosome end as well as from the promoter (Figure 1B). However, Batram et al. also propose an unexpected link between VSG coat switching and how the parasite progresses through its life cycle. When trypanosomes reach high densities in the mammalian host, do they follow predetermined genetic programming in the hope that they will be picked up by a tsetse fly? Or alternatively, can trypanosomes reversibly probe various options? The answers will certainly prove informative as to how this seemingly simple single-celled parasite outsmarts its more highly evolved hosts.
References
-
Molecular mechanisms underlying the control of antigenic variation in African trypanosomesCurrent Opinion in Microbiology 13:700–705.https://doi.org/10.1016/j.mib.2010.08.009
-
Trypanosomal immune evasion, chronicity and transmission: an elegant balancing actNature Reviews Microbiology 10:431–438.https://doi.org/10.1038/nrmicro2779
Article and author information
Author details
Publication history
Copyright
© 2014, Ooi and Rudenko
This article is distributed under the terms of the Creative Commons Attribution License, which permits unrestricted use and redistribution provided that the original author and source are credited.
Metrics
-
- 967
- views
-
- 42
- downloads
-
- 0
- citations
Views, downloads and citations are aggregated across all versions of this paper published by eLife.
Download links
Downloads (link to download the article as PDF)
Open citations (links to open the citations from this article in various online reference manager services)
Cite this article (links to download the citations from this article in formats compatible with various reference manager tools)
Further reading
-
How the parasite that causes sleeping sickness keeps one step ahead of its host.
-
- Cell Biology
Distal appendages are nine-fold symmetric blade-like structures attached to the distal end of the mother centriole. These structures are critical for formation of the primary cilium, by regulating at least four critical steps: ciliary vesicle recruitment, recruitment and initiation of intraflagellar transport (IFT), and removal of CP110. While specific proteins that localize to the distal appendages have been identified, how exactly each protein functions to achieve the multiple roles of the distal appendages is poorly understood. Here we comprehensively analyze known and newly discovered distal appendage proteins (CEP83, SCLT1, CEP164, TTBK2, FBF1, CEP89, KIZ, ANKRD26, PIDD1, LRRC45, NCS1, CEP15) for their precise localization, order of recruitment, and their roles in each step of cilia formation. Using CRISPR-Cas9 knockouts, we show that the order of the recruitment of the distal appendage proteins is highly interconnected and a more complex hierarchy. Our analysis highlights two protein modules, CEP83-SCLT1 and CEP164-TTBK2, as critical for structural assembly of distal appendages. Functional assays revealed that CEP89 selectively functions in RAB34+ ciliary vesicle recruitment, while deletion of the integral components, CEP83-SCLT1-CEP164-TTBK2, severely compromised all four steps of cilium formation. Collectively, our analyses provide a more comprehensive view of the organization and the function of the distal appendage, paving the way for molecular understanding of ciliary assembly.