Fibrosis: How collagen becomes ‘stiff’
When our bodies heal following an injury, fibroblasts and other cells deposit components of the extracellular matrix, such as collagen, that form a ‘scar’ over the damaged area. This process, known as fibrosis, leads to a build-up of stiff fibrotic tissue that interferes with the activity of the underlying organ.
In healthy organs, fibroblasts lay down just enough collagen to maintain the integrity of the tissue (D’Urso and Kurniawan, 2020). However, if the tissue begins to stiffen, these cells produce more collagen, disrupting this equilibrium (Tschumperlin et al., 2018). This increases the rigidity of the organ, triggering the fibroblasts to release even more collagen. While this explains how fibrosis progresses, it is less clear how the cycle starts.
Previous studies showed that fibrosis is initiated by changes in the mechanical properties of collagen that arise from modifications to its structure (Jones et al., 2018; Georges et al., 2007). The experiments found that, after a tissue injury, a family of enzymes called lysyl oxidases (or LOXs for short) increase the bonds between individual collagen fibers in the organ. This modified structure makes the tissue stiffer, driving the fibrotic process. Now, in eLife, Mark Jones from the University of Southampton and co-workers – including Christopher Brereton and Liudi Yao as joint first authors – report on the pathway that leads to this altered collagen architecture in patients with pulmonary fibrosis (Brereton et al., 2022).
First, the team (who are based at various institutes in the United Kingdom, the United States, Austria, Italy and Ireland) studied lung tissue from individuals with idiopathic pulmonary fibrosis, a disease of unknown cause in which patient’s lungs are severely scarred (Richeldi et al., 2017). They found that the genes coding for two enzymes that sequentially modify collagen (Yamauchi and Sricholpech, 2012) – lysyl hydroxylase PLOD2 and LOXL2 – were highly expressed in the same lung cells at the same time. The gene for the most commonly found type of collagen in fibrosis (called type I) was also activated in the lung tissue, but its pattern of expression did not correlate with the genes for PLOD2 or LOXL2. This suggests that it is regulated independently from these two enzymes.
Next, Brereton, Yao et al. extracted fibroblasts from patients with idiopathic pulmonary fibrosis and cultured them in the laboratory for six weeks, treating the cells with several growth factors associated with fibrosis. The experiments showed that a family of proteins called Hypoxia Inducible Factors (HIFs), which regulate the body’s response to varying oxygen levels, activated the genes for PLOD2 and LOXL2. This increased the number of cross-links between the collagen fibers and made the fibers stiffer. Imaging the collagen produced by these cells with an electron microscope revealed that the fibers had a smaller diameter, as had previously been observed in patients with idiopathic pulmonary fibrosis (Jones et al., 2018). This suggests that HIFs regulate tissue mechanics not by increasing the amount of collagen, but by altering collagen’s structure.
HIF is negatively regulated by another protein called FIH (short for Factor Inhibiting HIF), which is inactivated by the unstable byproducts of oxygen metabolism, also known as oxidative stress (Lando et al., 2002). Loss of FIH causes cells to enter ‘pseudohypoxia’, a state in which cells behave as if oxygen levels are low despite being in normal conditions (Masson et al., 2012). Brereton, Yao et al. found that reducing FIH – both in cells cultured in the laboratory as well as in lung tissue from patients with idiopathic pulmonary fibrosis – led to higher levels of HIF, which increased the activity of PLOD2 and LOX2, causing changes to collagen’s architecture and fibrosis.
These findings suggest that the pseudohypoxia state induced by oxidative stress is central to the pathology of idiopathic pulmonary fibrosis, and may help to explain how the fibrosis cycle starts (Figure 1): injuring or damaging the lung leads to a rise in oxidative stress, which dampens the level of FIH. This allows HIF to activate the genes for the enzymes PLOD2 and LOXL2, which then sequentially alter the structure of collagen. These changes increase the stiffness of the tissue, initiating the fibrosis cycle.
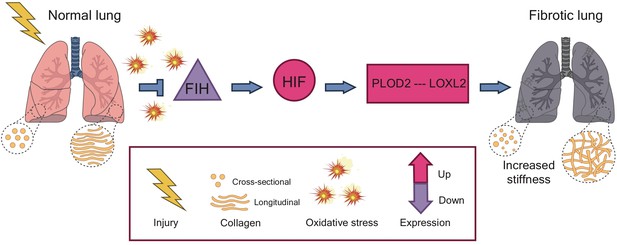
The pathway to fibrosis.
When cells in the lung become injured or damaged (left), the resulting increase in oxidative stress leads to a decrease in the expression of the genes that code for a protein called FIH (purple triangle). This results in a pseudohypoxia state which increases the activity of the protein HIF (pink circle), allowing it to upregulate the expression of the genes that code for two enzymes: PLOD2 and LOXL2. These enzymes act in sequence to increase and strengthen the bonds, or ‘cross-links’, between fibers of collagen. The altered structure stiffens the tissue, triggering cells in the lung to deposit more collagen and start the process of fibrosis. HIF: Hypoxia Inducible Factor; FIH: Factor Inhibiting HIF.
Image credit: Iris de Jong (CC BY 4.0).
This work provides new insights in to how fibrosis is triggered. Particularly intriguing is the suggestion that intervening at the level FIH or HIF may be better at treating idiopathic pulmonary fibrosis than targeting the machinery that synthesizes collagen, which is currently considered to be the most effective approach. Further work is needed to fully understand how the architecture of collagen becomes dysregulated and to test how substances that block this newly discovered pathway impact fibrosis.
References
-
Mechanical and physical regulation of fibroblast-myofibroblast transition: from cellular mechanoresponse to tissue pathologyFrontiers in Bioengineering and Biotechnology 8:609653.https://doi.org/10.3389/fbioe.2020.609653
-
Increased stiffness of the rat liver precedes matrix deposition: implications for fibrosisAmerican Journal of Physiology. Gastrointestinal and Liver Physiology 293:G1147–G1154.https://doi.org/10.1152/ajpgi.00032.2007
-
Mechanosensing and fibrosisThe Journal of Clinical Investigation 128:74–84.https://doi.org/10.1172/JCI93561
-
Lysine post-translational modifications of collagenEssays in Biochemistry 52:113–133.https://doi.org/10.1042/bse0520113
Article and author information
Author details
Publication history
Copyright
© 2022, Wells
This article is distributed under the terms of the Creative Commons Attribution License, which permits unrestricted use and redistribution provided that the original author and source are credited.
Metrics
-
- 7,643
- views
-
- 414
- downloads
-
- 16
- citations
Views, downloads and citations are aggregated across all versions of this paper published by eLife.
Download links
Downloads (link to download the article as PDF)
Open citations (links to open the citations from this article in various online reference manager services)
Cite this article (links to download the citations from this article in formats compatible with various reference manager tools)
Further reading
-
- Cancer Biology
- Cell Biology
Testicular microcalcifications consist of hydroxyapatite and have been associated with an increased risk of testicular germ cell tumors (TGCTs) but are also found in benign cases such as loss-of-function variants in the phosphate transporter SLC34A2. Here, we show that fibroblast growth factor 23 (FGF23), a regulator of phosphate homeostasis, is expressed in testicular germ cell neoplasia in situ (GCNIS), embryonal carcinoma (EC), and human embryonic stem cells. FGF23 is not glycosylated in TGCTs and therefore cleaved into a C-terminal fragment which competitively antagonizes full-length FGF23. Here, Fgf23 knockout mice presented with marked calcifications in the epididymis, spermatogenic arrest, and focally germ cells expressing the osteoblast marker Osteocalcin (gene name: Bglap, protein name). Moreover, the frequent testicular microcalcifications in mice with no functional androgen receptor and lack of circulating gonadotropins are associated with lower Slc34a2 and higher Bglap/Slc34a1 (protein name: NPT2a) expression compared with wild-type mice. In accordance, human testicular specimens with microcalcifications also have lower SLC34A2 and a subpopulation of germ cells express phosphate transporter NPT2a, Osteocalcin, and RUNX2 highlighting aberrant local phosphate handling and expression of bone-specific proteins. Mineral disturbance in vitro using calcium or phosphate treatment induced deposition of calcium phosphate in a spermatogonial cell line and this effect was fully rescued by the mineralization inhibitor pyrophosphate. In conclusion, testicular microcalcifications arise secondary to local alterations in mineral homeostasis, which in combination with impaired Sertoli cell function and reduced levels of mineralization inhibitors due to high alkaline phosphatase activity in GCNIS and TGCTs facilitate osteogenic-like differentiation of testicular cells and deposition of hydroxyapatite.
-
- Cell Biology
G protein-coupled receptors (GPCRs) are integral membrane proteins which closely interact with their plasma membrane lipid microenvironment. Cholesterol is a lipid enriched at the plasma membrane with pivotal roles in the control of membrane fluidity and maintenance of membrane microarchitecture, directly impacting on GPCR stability, dynamics, and function. Cholesterol extraction from pancreatic beta cells has previously been shown to disrupt the internalisation, clustering, and cAMP responses of the glucagon-like peptide-1 receptor (GLP-1R), a class B1 GPCR with key roles in the control of blood glucose levels via the potentiation of insulin secretion in beta cells and weight reduction via the modulation of brain appetite control centres. Here, we unveil the detrimental effect of a high cholesterol diet on GLP-1R-dependent glucoregulation in vivo, and the improvement in GLP-1R function that a reduction in cholesterol synthesis using simvastatin exerts in pancreatic islets. We next identify and map sites of cholesterol high occupancy and residence time on active vs inactive GLP-1Rs using coarse-grained molecular dynamics (cgMD) simulations, followed by a screen of key residues selected from these sites and detailed analyses of the effects of mutating one of these, Val229, to alanine on GLP-1R-cholesterol interactions, plasma membrane behaviours, clustering, trafficking and signalling in INS-1 832/3 rat pancreatic beta cells and primary mouse islets, unveiling an improved insulin secretion profile for the V229A mutant receptor. This study (1) highlights the role of cholesterol in regulating GLP-1R responses in vivo; (2) provides a detailed map of GLP-1R - cholesterol binding sites in model membranes; (3) validates their functional relevance in beta cells; and (4) highlights their potential as locations for the rational design of novel allosteric modulators with the capacity to fine-tune GLP-1R responses.